Abstract
The role of the Arabidopsis thaliana genes SNRK2s, which are similar to the sulfur-regulatory gene SAC3 of Chlamydomonas reinhardtii, in the response of plants to sulfur was studied. The Arabidopsis genome contains 10 genes (SNRK2.1 to SNRK2.10) similar to SAC3. Among these genes, transcript accumulation of several genes including SNRK2.3 was induced in response to sulfur starvation. Independently isolated transgenic A. thaliana lines carrying T-DNA insertions in SNRK2.3 exhibited reduced sulfur-starvation induction of the transcript of the sulfate transporter SULTR2;2 gene, whereas the accumulation of O-acetyl-l-serine under sulfur deficiency conditions increased. These results suggest that SNRK2.3 plays a beneficial role in the regulation of gene expression and metabolism in response to sulfur starvation.
INTRODUCTION
Sulfur is an essential macronutrient for the growth and development of higher plants. It is a constituent of a variety of substances including proteins, vitamins, coenzymes, and as glutathione (GSH) or ferredoxin sulfur plays a critical biochemical role in the reduction of molecular compounds or detoxification of heavy metals and xenobiotics. Sulfur is initially taken up in the form of sulfate from the environment. Reduced and assimilated sulfate is incorporated into the first carbon compound cysteine (Cys) and then converted into methionine, GSH or other sulfur-containing organic compounds.
Plants can adapt to restricted sulfur availability (referred to as –S) through developmental and physiological changes such as elongation of roots, anthocyanin of, and decrease in photosynthetic capacity. Metabolic and transcriptional adaptation involves the induction of sulfur-responsive genes, an increase in O-acetyl-l-serine (OAS) content, and a decrease in GSH, Cys and sulfate contents (see CitationLeustek and Saito 1999; CitationSaito 2000, Citation2004 for reviews). Plants have acquired this variety of responses to –S to enhance the exploitation of exogenous or endogenous sulfur and to survive in the absence of sulfur nutrition over a long period of time.
Available evidence suggests that several signal molecules play important roles in the regulation of the −S response. O-acetyl-l-serine, a direct precursor of Cys, is considered to be a putative positive regulator for the –S response. Application of OAS to sulfur-sufficient media (referred to as +S) resulted in increased activity and mRNA accumulation of adenosine 5′-phosphosulfate reductase (APR), a key enzyme in the sulfur assimilation pathway. In addition, OAS markedly increased the transcript levels of sulfite reductase, Cys synthase and serine acetyltransferase (SAT), all of which function in the sulfur assimilation pathway (CitationKoprivova et al. 2000). Furthermore, profiles of global transcriptome analysis revealed that OAS application triggers similar changes in the expression pattern of leaf transcripts to those of –S-treated leaves, suggesting that OAS is a global regulator of –S-responsive gene expression (CitationHirai et al. 2003). O-acetyl-l-serine is not likely to be the sole determinant of –S-regulated gene expression. The effect of OAS may possibly be ascribed to the depletion of intermediates such as sulfide (CitationHopkins et al. 2005). Glutathione and Cys are also considered to be negative signals for −S response. Application of either GSH or Cys to S-starved plants decreased the transcript level of ATP sulfurylase and sulfate transporter (CitationLappartient et al. 1999). Transcriptome (CitationHirai et al. 2003; CitationNikiforova et al. 2003) and metabolome (CitationHirai et al. 2004) analysis has indicated that plants carry a global network of genes and metabolites in response to −S, with integration of particular metabolic pathways such as flavonoid, auxin, jasmonate (CitationNikiforova et al. 2003) and glucosinolate. Genes and metabolites are regulated in a coordinated response to prolonged −S (CitationHirai et al. 2004).
Although the function of signal molecules such as OAS, GSH and Cys has become obvious, how changes in these metabolite levels and sulfur status, and by which mechanism the expression of sulfur-responsive genes change, still remain to be elucidated. This mechanism has not yet been determined in plants, unlike in bacteria, yeasts and algae.
Several regulatory genes of the −S response have been identified by mutant screening in a green alga Chlamydomonas reinhardtii (CitationDavies et al. 1994). One of the regulatory genes, SAC3, has been identified and characterized as a member of the plant Snf1-related protein kinase (SNRK2) family. Under –S conditions, Chlamydomonas cells exhibit responses involving the secretion of periplasmic proteins such as arylsulfatase (Ars) and the induction of a high-affinity transport system. In contrast to wild-type cells, the sac3 mutant accumulates Ars even under +S conditions and it cannot fully induce a high-affinity transport system during −S. These findings indicate that the SAC3 protein is a factor that regulates the response to −S both positively and negatively (CitationDavies et al. 1999).
In an attempt to elucidate the mechanism of acclimation to −S in vascular plants, we considered that the regulatory function of C. reinhardtii SAC3 (CrSAC3) on −S is also conserved in the vascular plant Arabidopsis thaliana, assuming that assimilatory mechanisms to −S are, at least in part, conserved in photosynthetic eukaryotes during biological evolution. In the present study, we targeted A. thaliana homologues with sequence similarity to CrSAC3 and considered the possibility that a conservation mechanism was involved in sulfur acclimation between C. reinhardtii and A. thaliana.
Based on a database search, A. thaliana has 10 SAC3 homologues, designated SNRK2.1–SNRK2.10 in the present study, all of which belong to the plant SNRK2 family and presumably encode protein kinases. Members of SNRK2 have been reported to participate in abscisic acid (ABA), salinity and osmotic stress signaling (CitationMustilli et al. 2002; CitationUmezawa et al. 2004; CitationYoshida et al. 2002). Thus, SNRK2 could be responsible for stress signaling associated with environmental changes involving a deficiency in major essential nutrients.
In the present study, we observed that several SNRK2 transcripts were induced in response to –S, and one of them, SNRK2.3, was targeted for detailed analysis. Repression of the SNRK2.3 gene reduced –S-induction of transcript accumulation of the sulfate transporter SULTR2;2 gene and enhanced OAS accumulation under –S conditions, suggesting that SNRK2.3 mediates part of the sulfur acclimation response.
MATERIALS AND METHODS
Plant materials and culture conditions
In the present study, A. thaliana ecotype Wassilewskija (Ws) was used as a wild-type control for the snrk2.3-1 mutant, and Columbia-0 (Col-0) was used as a control for the snrk2.3-2 and snrk2.3-3 mutants (see below for a description of these mutant lines). Surface-sterilized seeds were sown on a culture medium plate (CitationHirai et al. 1995) solidified with 0.6% agarose (LO3, Takara, Kyoto, Japan) containing 1% sucrose (w/v). For +S (S 1.5 mmol L−1) conditions, sulfate was provided as MgSO4 and for the –S (S 0.03 mmol L−1) conditions the MgSO4 was replaced with equi-molar of MgCl2. Plants were grown at 22ºC under a 16 h light/8 h dark cycle under fluorescent light after a 4-day vernalization at 4ºC. After 10 days of culture on a +S plate, the plants were transferred to a +S or –S plate and grown for 4 days before harvest.
Arabidopsis thaliana plants were also grown on rockwool (Nittobo Company, Tokyo, Japan) to which hydroponic culture solutions were provided at 22ºC under fluorescent light (16 h light/8 h dark). Plants were hydroponically watered with +S or –S culture medium throughout the 21-day period of culture.
Isolation of T-DNA insertion mutants in SNRK2.3
We used three independent snrk2.3 alleles carrying T-DNA insertion in the present study. Seeds were obtained from the SALK Institute (http://signal.salk.edu/index.html) for snrk2.3-2 and snrk2.3-3, and from the Arabidopsis Knockout Facility at the University of Wisconsin (http://biotech.wisc.edu/Arabidopsis/Index2.asp) for snrk2.3-1. Plants homozygously carrying the T-DNA insertion were isolated using polymerase chain reaction (PCR) analysis with Taq DNA polymerase (Rosche, Basel, Switzerland) and used throughout the experiment. The insertion sites of T-DNA were determined by sequencing using the ABI Prism BigDye terminator cycle sequencing ready reaction kit and an ABI 377-18 DNA sequencer (Applied Biosystems, Tokyo, Japan).
Quantitative real-time PCR analysis
Total RNA was extracted from shoots using the RNeasy Plant Mini Kit (Qiagen, Valencia, CA, USA), including treatment with DNase (Qiagen). RNA was reverse-transcribed with MuLV reverse transcriptase and an oligo-dT(16) primer (Applied Biosystems). The cDNAs obtained were used for quantitative PCR in the SmartCycler (Cepheid, Sunnyvale, CA, USA) instrument with ExTaq R-PCR Version (Takara). Transcript levels were detected using the following primers. For SNRK2.1 5′-ggcgagagagattattaatcacagatc-3′ and 5′-agatgagtaggtgtcaggatcacctc-3′, for SNRK2.2 5′-ccgattatgccgattgattt-3′ and 5′-tatcggtcatgagacgagca-3′, for SNRK2.3 5′-gcaatgcaggacggtttagt-3′ and 5′-gagcaggacttccatccaac-3′, for SNRK2.4 5′-gcgtatatgcagtgctggaa-3′ and 5′-agcaggacttccatccaaga-3′, for SNRK2.6 5′-aacttttcgagcgaatctgc-3′ and 5′-gccgggctaccatctaataac-3′, for SNRK2.7 5′-tggcaagatgtgttggtgtt-3′ and 5′-agccaacggagagttttacg-3′, for SNRK2.9 5′-ctcctccagctgcatactcc-3′ and 5′-ttcatcgatcgaggctacaa-3′, for APR1 5′-cattggagccaaaagtttcgc-3′ and 5′-tcctcaatctcaaccacatcaac-3′, for SULTR2;2 5′-cgacatgtcttgcgtgatgggcg-3′ and 5′-gctcgcttcaatttgtgaagtaccct-3′, and for β-tubulin as internal standard 5′-gctcgctaatcctacctttgg-3′ and 5′-agccttgggaatgggataag-3′ were used. The gene specificity of the primers was confirmed by electrophoresis of the PCR products and by melting curve analysis using the SmartCycler instrument.
Determination of OAS, GSH and sulfate concentrations
Extraction and analysis of OAS in shoots were carried out as previously described (CitationKim et al. 1999). Concentrations of sulfate and GSH in shoots were also determined as previously described (CitationKuzuhara et al. 2000; CitationOhkama et al. 2002).
RESULTS
Database search for CrSAC3 homologues of A. thaliana
To search for SAC3 homologues of A. thaliana, a BLAST search was carried out in the Arabidopsis Information Database, http://www.arabidopsis.org/ using C. reinhardtii SAC3 amino acid sequence as a query. Ten proteins with more than 60% identity with or similarity to CrSAC3 were designated as SNRK2.1–SNRK2.10, all of which belonged to Snf1-related protein kinase2 (SNRK2), in addition to CrSAC3. Based on the previous description by CitationDavies et al. (1999), the N-terminal kinase specific catalytic domain and C-terminal two subdomains and an acidic domain were conserved in all 10 SNRK2 sequences in A. thaliana. The phylogenetic trees of the SNRK2s and CrSAC3 are shown in .
In the present study, we examined the roles of SNRK2.3 in the –S response. Comparison of the amino acid sequences shown in revealed a high similarity in the serine/threonine protein kinase domain.
Identification of –S-induced SNRK2 gene, SNRK2.3
To determine whether the accumulation of any SNRK2 transcripts was altered in response to −S, quantitative reverse transcription-polymerase chain reaction (RT-PCR) was carried out to detect mRNA accumulation of SNRK2s in the shoots of the wild-type plants. A number of genes responsive to −S were also found to be responsive to OAS (CitationHirai et al. 2003). In the present study, we examined the accumulation of transcripts of the SNRK2 genes under two culture conditions, −S and +S with 5 mmol L−1 OAS (referred to as +OAS).
Seven of the 10 SNRK2 genes listed in were examined in this experiment. In four genes, SNRK2.1, SNRK2.3, SNRK2.4 and SNRK2.6, the transcript levels were increased in response to −S and OAS application, whereas the SNRK2.2 transcript was not significantly increased (). SNRK2.7 and SNRK2.9 transcripts were not detected in the present study. These results indicate that at least four of the SNRK2 genes were upregulated under −S conditions.
We then carried out a detailed analysis of the SNRK2.3 gene. SNRK2.3 was upregulated by −S and displayed the second highest sequence similarity to CrSAC3 among the 10 SNRK2s. Our subsequent experiment with quantitative RT-PCR represented the induction of the SNRK2.3 transcript in response to −S more clearly ().
Establishment of snrk2.3 knockout lines
For further observation of the SNRK2.3 gene, we obtained three independent alleles with T-DNA insertion from two different institutions, namely, snrk2.3-1, ecotype Ws, from the Arabidopsis Knockout Facility at the University of Wisconsin and snrk2.3-2 and snrk2.3-3, ecotype Col-0, from the SALK Institute (). The T4 plants harboring T-DNA homozygously were used for the following experiments. Positions of T-DNA insertion from the 5′UTR end in snrk2.3-1, snrk2.3-2 and snrk2.3-3 corresponded to 2228–2229, 1172–1183 and 327–349 bp, respectively. SNRK2.3 mRNA accumulation in the leaves of the snrk2.3-1, snrk2.3-2 and snrk2.3-3 mutants decreased to 16%, 0.019% and 1.4%, respectively, of that of the wild-type plants (). Shoot fresh weight and root length of the mutants were
Figure 1 (A) Phylogenetic tree of Chlamydomonas CrSAC3 and Arabidopsis SNRK2s. CrSAC3 and SNRK2.3 are enclosed within bold boxes. (B) Comparison of the amino acid sequence of CrSAC3 and SNRK2.3. Black and gray boxes indicate identical and similar amino acid residues, respectively. Black solid lines and gray dotted lines under the residues indicate Ser/Thr kinase domain and SNRK2 specific domain, respectively.
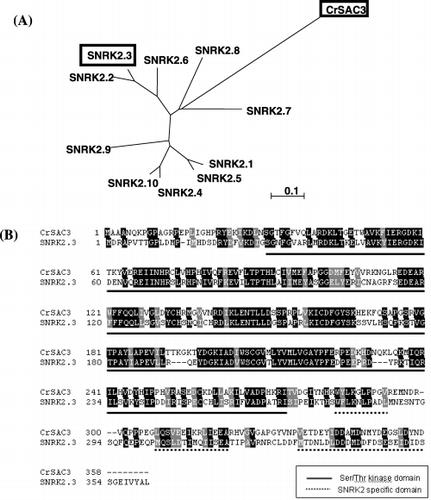
Sulfate concentration in snrk2.3-1 mutant plants
We measured the level of sulfate using capillary electrophoresis to evaluate the effect of SNRK2.3 on sulfate concentration. Shoots of 21-day grown plants under +S or −S conditions were measured. No differences in the sulfate level between the snrk2.3-1 mutant and the wild-type plants were detected, regardless of the environmental sulfur status (), which suggests that the concentration of sulfate was not directly controlled by the SNRK2.3 gene.
Extent of reduction of −S induction of SULTR2;2 in snrk2.3 mutants
No significant differences were observed in plant growth or endogenous sulfate concentration in the snrk2.3-1 mutant compared to the wild type. We then examined the possibility that SNRK2.3 may be involved in the
Table 1 List of SNRK2 genes in Arabidopsis thaliana
Figure 2 mRNA accumulation of five SNRK2 genes in shoots of wild-type Columbia-0 (Col-0). (A) Relative expression of SNRK2.1, SNRK2.2, SNRK2.3, SNRK2.4 and SNRK2.6 (mean ± standard deviation [SD], n = 3). (B) Additional measurement of SNRK2.3 relative expression (mean ± SD, n = 3). Asterisks indicate values significantly different from the +S conditions (P < 0.05, Student's t-test). Plants were initially grown on an agarose plate for 10 days under +S (1.5 mmol L−1 S) conditions, then grown for another 4 days after transfer to +S, −S (0.03 mmol L−1 S) or +S+O-acetyl-l-serine (OAS) (1.5 mmol L−1 S, 5 mmol L−1 OAS ) medium.
![Figure 2 mRNA accumulation of five SNRK2 genes in shoots of wild-type Columbia-0 (Col-0). (A) Relative expression of SNRK2.1, SNRK2.2, SNRK2.3, SNRK2.4 and SNRK2.6 (mean ± standard deviation [SD], n = 3). (B) Additional measurement of SNRK2.3 relative expression (mean ± SD, n = 3). Asterisks indicate values significantly different from the +S conditions (P < 0.05, Student's t-test). Plants were initially grown on an agarose plate for 10 days under +S (1.5 mmol L−1 S) conditions, then grown for another 4 days after transfer to +S, −S (0.03 mmol L−1 S) or +S+O-acetyl-l-serine (OAS) (1.5 mmol L−1 S, 5 mmol L−1 OAS ) medium.](/cms/asset/5ae52b5a-e974-4113-a143-532fd6c30d52/tssp_a_10382345_o_f0002g.gif)
Figure 3 (A) T-DNA insertion sites of snrk2.3 mutants. (B) SNRK2.3 mRNA accumulation in 14-day shoots of wild-type Wassilewskija (Ws) (▪) and snrk2.3 mutants (□) grown on +S agarose plates. Transcript level significantly decreased in all three mutants (mean ± standard deviation, n = 3). n.d., not detected.
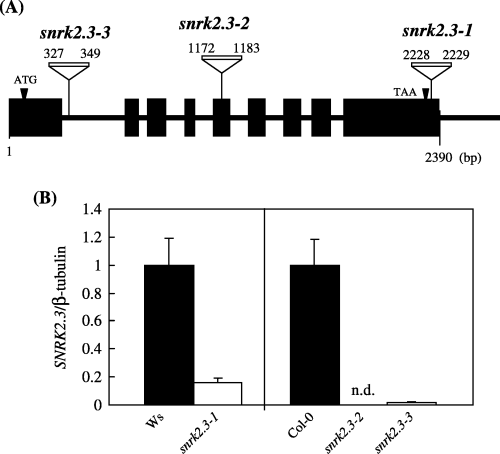
As shown in , SULTR2;2 gene expression was upregulated approximately 3.7-fold in the Ws wild-type plant, whereas in the snrk2.3-1 mutant, although
moderate induction of SULTR2;2 was detected, the induction rate (2.5-fold) was reduced compared to that in the wild-type plant. In the snrk2.3-2 and snrk2.3-3 mutants, the induction rates were approximately 1.2-fold and 0.8-fold, respectively, whereas in the Col-0 wild type the rate was approximately 2.6-fold, indicating the absence of –S-induced expression of the SULTR2;2 gene in these two mutants (). These results were observed in three independent snrk2.3 mutants and indicated that the extent of –S induction of SULTR2;2 was reduced when SNRK2.3 was destroyed.Increase in OAS concentration in the snrk2.3 mutants compared to the wild type under –S conditions
O-acetyl-l-serine, a direct precursor of Cys, is considered to be a key compound that positively regulates the −S response, whereas GSH is considered to be a signal compound regulating the −S response in a negative manner. The fact that the accumulation of SULTR2;2 transcripts was affected in the snrk2.3 mutants suggested that the concentration of these compounds was also altered in the mutants.
Concentrations of OAS and GSH in the shoots of the snrk2.3 mutants and the wild type are shown in . In all three snrk2.3 alleles, the OAS concentration under −S conditions increased compared to that in the wild type. In the snrk2.3-1 mutant, the increase in OAS concentration by –S was statistically 1.3-fold higher than that in the wild-type Ws (). In the snrk2.3-2 and snrk2.3-3 mutants, the OAS levels under −S conditions were 2.5-fold and 1.8-fold higher than the wild-type Col-0, respectively (). Meanwhile under +S conditions, the level of OAS in the snrk2.3-1 and
Figure 5 Changes in SULTR2;2 mRNA accumulations in shoots of (A) wild-type Wassilewskija (Ws) and snrk2.3-1 mutant and (B) wild-type Columbia-0 (Col-0), and snrk2.3-2 and snrk2.3-3 mutants (mean ± standard deviation, n = 3). (▪), +S conditions; (□) –S conditions. Plants were grown as indicated in Fig. 2.
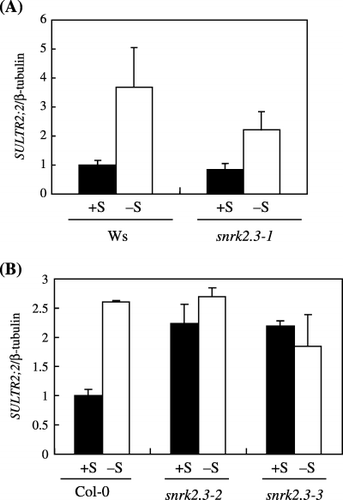
These results indicated that the mutants accumulated OAS excessively compared to the wild type when exposed to sulfur limitation, suggesting that the accumulation of OAS under −S conditions was regulated by SNRK2.3. Under normal conditions, SNRK2.3 may downregulate the OAS concentration to an appropriate level to prevent over-accumulation of OAS when sulfur is not available.
As shown in , no statistical differences were observed between the snrk2.3-1 mutant and the wild type under +S conditions, whereas under −S conditions the concentration of GSH in the snrk2.3-1 mutant moderately increased approximately 1.2-fold compared to the wild type. This slight increase is unlikely to reflect the effect of
Figure 6 O-acetyl-l-serine (OAS) concentration in shoots of (A) wild-type Wassilewskija (Ws) and snrk2.3-1 mutant and (B) wild-type Columbia-0 (Col-0), and snrk2.3-2 and snrk2.3-3 mutants (mean ± standard deviation, n = 4). (C) Glutathione (GSH) concentration in shoots of wild-type Ws and snrk2.1-1 mutant. Asterisk indicates values significantly different than the wild type (P < 0.05, Student's t-test). Plants were grown as indicated in Fig. 2.
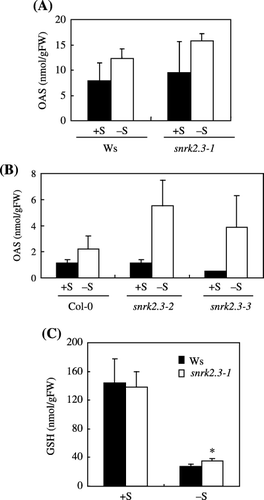
DISCUSSION
Plants have developed elaborate adaptations to enhance sulfur availability under −S conditions through changes in the expression of sulfur-responsive genes and metabolite levels. Nonetheless, the regulatory mechanism that controls the −S response remains to be elucidated. Chlamydomonas reinhardtii has been used as a model system to investigate the acclimation of photosynthetic organisms to −S, leading to the identification of several sulfur regulatory genes. One such gene, SAC3, encodes serine/threonine protein kinase and controls the sulfur acclimation response both positively and negatively.
A database search revealed the presence in A. thaliana of 10 homologous genes to SAC3, designated as SNRK2.1–SNRK2.10. In the present study, we observed that 4 of the 7 SNRK2 genes tested were induced by –S or OAS treatment at the mRNA level, including our targeted gene SNRK2.3. Several investigators have reported that SNRK2s were activated by ABA, salinity, drought and osmotic stresses in A. thaliana (CitationBoudsocq et al. 2004; CitationMustilli et al. 2002; CitationUmezawa et al. 2004; CitationYoshida et al. 2002), tobacco (CitationKelner et al. 2004; CitationMikolajczyk et al. 2000), soybean (CitationMonks et al. 2001), wheat (CitationAnderberg et al. 1992; CitationHolappa et al. 1995) and rice (CitationKobayashi et al. 2004). Based on these reports it is possible that the SNRK2 members in plants operate under certain environmental stresses (CitationDavies et al. 1999; CitationHalford and Hardie 1998), including sulfur starvation stress. An assay of SNRK2 in relation to –S would be indispensable to determine the function of SNRK2s in sulfur limitation.
Disruption of the SNRK2.3 gene affected two aspects of the sulfur starvation responses in our study. Independently isolated mutants carrying T-DNA insertions in the SNRK2.3 gene exhibited similar phenotypes, suggesting that the disruption of the SNRK2.3 gene is the cause of the commonly observed phenotype.
First, in three independent snrk2.3 mutants, the induction of the expression of the sulfate transporter SULTR2;2 gene was impaired in response to −S (). This result suggests that SNRK2.3 may participate in the control of SULTR2;2 expression at a lower level when the sulfur supply is sufficient, or in the positive induction of SULTR2;2 when the sulfur supply is insufficient. mRNA accumulation of other sulfur-responsive genes such as APR1 and SAT1, one of the isoforms coding for APR and SAT, respectively, was not statistically different between the mutants and the wild type (data not shown), indicating that it is unlikely that SNRK2.3 is involved in the general regulation of sulfur-responsive genes, but that it could be involved in the regulation of specific genes including SULTR2;2.
Second, the concentration of OAS increased under –S conditions in the three independent snrk2.3 mutants compared to the wild type, suggesting that SNRK2.3 may negatively control the OAS concentration at an appropriate level to prevent excessive accumulation of OAS, and to prevent abnormal transcriptional or metabolic changes when the sulfur supply is restricted. In addition to the present study, another Arabidopsis gene that controls OAS concentration has been previously identified by our group through osh1-1 mutant analysis. OSH1 encodes a thiol reductase and the osh1 mutant over-accumulated OAS under both +S and –S conditions, along with the elevated gene expression of APR1 and SULTR2;2 (CitationOhkama-Ohtsu et al. 2004). This report and our present finding indicate that the concentration of OAS can be controlled by genes other than those of the sulfur assimilation pathway, such as the OAS synthetic enzyme SAT. Evidence has revealed that overproduction of watermelon SAT in A. thaliana resulted in the over-accumulation of OAS together with an increase in the Cys and GSH levels (CitationNoji et al. 2002).
We can propose hypothetic roles of SNRK2.3 in sulfur assimilation. O-acetyl-l-serine is a putative signal compound of the −S response. Application of OAS triggers upregulation of sulfur-responsive genes in a similar way to that of −S. In this context, increased OAS levels in the snrk2.3 mutants under –S conditions would result in the over-accumulation of SULTR2;2 transcripts. Contrary to our expectation, however, the transcript levels of SULTR2;2 in the snrk2.3 mutants did not increase under –S conditions compared with those in the wild type, or rather, the induction of SULTR2;2 itself by –S was not, or only weakly, observed in the snrk2.3 mutants. Therefore, over-accumulation of OAS and reduced induction of SULTR2;2 in the snrk2.3 mutants may not be related. We suggest that SNRK2.3 functions independently in the prevention of OAS over-accumulation and in the active induction of SULTR2;2 gene expression, and that it plays a role in the response to sulfur starvation (). This is comparable to C. reinhardtii SAC3, which controls both positive and negative responses to −S, namely, active sulfate transport under –S and prevention of Ars secretion under +S conditions. However, there were differences in the sulfur response, such as the lack of induction of CrSAC3 by –S and in indicators like Ars and OAS. Such characteristics may result from differences in species and from the diversity of genes because C. reinhardtii harbors only one SAC3 gene whereas A. thaliana has 10 homologues. Therefore, it should be noted that the suppression of only one SNRK2.3 gene may not correspond to the suppression of the CrSAC3 gene, and direct comparison between mutants of these species may not be appropriate. Still, the evidence clearly showed that SNRK2.3 and CrSAC3 displayed common properties, in that they affected some aspects of the sulfur starvation responses, suggesting that
Figure 7 Possible role of SNRk2.3 in sulfur assimilation. SNRK2.3 is likely to be involved in the sulfur response, as in the case of Chlamydomonas SAC3. The role of SNRK2.3 in sulfur assimilation would be to prevent the over accumulation of OAS while promoting the expression of the SULTR2;2 gene when the sulfur supply is limited.

It remains to be determined whether other members of the SNRK2 family control some aspects of the –S response, although at least four of them, SNRK2.1, SNRK2.3, SNRK2.4 and SNRK2.6, were found to be induced at the transcription level in the present study. Reverse genetic studies may elucidate this aspect and binding assay may reveal the interaction between the 10 homologues.
In our assumption, SNRK2.3 may act upstream of signal transduction in the regulation of the −S response by activating downstream structural genes such as SULTR2;2, presumably mediated by transcription factors, and the regulation may proceed through several stages of phosphorylation relay between other kinases. SPK1 and SPK2, soybean SNRK2 proteins, have been found to be involved in the perception of osmotic stress and signal transduction. Active forms of SPK1 and SPK2 are capable of phosphorylating Ssh1p, a soybean phosphatidylinositol transfer protein-like protein, leading to osmoprotection potentially through alterations of the phosphoinositide metabolism (CitationMonks et al. 2001). Another possible target of SNRK2 would be phosphatidic acid (PA), a lipid second messenger that participates in various stress signaling pathways. SNRK2.10 has been identified as a PA-binding protein by mass spectrometry (CitationTesterink et al. 2004). Identification of substrates or binding protein of SNRK2 kinase would lead to a better understanding of the −S response and to improved sulfur availability in higher plants.
In conclusion, we demonstrated that A. thaliana SNRK2.3, the homologue of C. reinhardtii SAC3, controls the OAS concentration and SULTR2;2 gene expression in response to −S. These findings indicate that the regulatory mechanism of the −S response may be evolutionarily conserved between algae and plants.
ACKNOWLEDGMENTS
The authors greatly appreciate the technical assistance of Yuko Kawara and Kayoko Aizawa. This work was supported in part by a Grant-in-Aid for Scientific Research on Priority Areas from the Ministry of Education, Culture, Sports, Science and Technology of Japan (grant no. 17078004 to T.F.) and by a 21st Century COE project (to T.F.).
Notes
Present address: 1-1-1 Yayoi, Bunkyo-ku, Tokyo 113-8657, Japan.
REFERENCES
- Leustek , T and Saito , K . 1999 . Sulfate transport and assimilation in plants . Plant Physiol , 120 : 637 – 643 .
- Saito , K . 2000 . Regulation of sulfate transport and synthesis of sulfur-containing amino acids . CurrOpinPlant Biol , 3 : 188 – 195 .
- Saito , K . 2004 . Sulfur assimilatory metabolism. The long and smelling road . Plant Physiol , 136 : 2443 – 2450 .
- Koprivova , A , Suter , M , Op den Camp , R , Brunold , C and Kopriva , S . 2000 . Regulation of sulfate assimilation by nitrogen in Arabidopsis . Plant Physiol , 122 : 737 – 746 .
- Hirai , MY , Fujiwara , T , Awazuhara , M , Kimura , T , Noji , M and Saito , K . 2003 . Global expression profiling of sulfur-starved Arabidopsisby DNA macroarray reveals the role of O-acetyl-l-serine as a general regulator of gene expression in response to sulfur nutrition . Plant J , 33 : 651 – 663 .
- Hopkins , L , Parmar , S , Baszczyk , A , Hesse , H , Hoefgen , R and Hawkesford , MJ . 2005 . O-acetylserine and the regulation of expression of genes encoding components for sulfate uptake and assimilation in potato . Plant Physiol , 138 : 433 – 440 .
- Lappartient , AG , Vidmar , JJ , Leustek , T , Glass , ADM and Bruno , T . 1999 . Inter-organ signaling in plants: regulation of ATP sulfurylase and sulfate transporter genes expression in roots mediated by phloem-translocated compound . Plant J , 18 : 89 – 95 .
- Nikiforova , V , Freitag , J , Kempa , S , Adamik , M , Hesse , H and Hoefgen , R . 2003 . Transcriptome analysis of sulfur depletion in Arabidopsis thaliana: interlacing of biosynthetic pathways provides response specificity . Plant J , 33 : 633 – 650 .
- Hirai , MY , Yano , M Goodenowe , DB . 2004 . Integration of transcriptomics and metabolomics for understanding of global responses to nutritional stresses in Arabidopsis thaliana . ProcNatl AcadSciUSA , 101 : 10205 – 10210 .
- Davies , JP , Yildiz , FH and Grossman , AR . 1994 . Mutants of Chlamydomonaswith aberrant responses to sulfur deprivation . Plant Cell , 6 : 53 – 63 .
- Davies , JP , Yildiz , FH and Grossman , AR . 1999 . Sac3, an Snf1-like serine threonine kinase that positively and negatively regulates the responses of Chlamydomonas to sulfur limitation . Plant Cell , 11 : 1179 – 1190 .
- Mustilli , AC , Merlot , S , Vavasseur , A , Fenzi , F and Giraudat , J . 2002 . ArabidopsisOST1 protein kinase mediates the regulation of stomatal aperture by abscisic acid and acts upstream of reactive oxygen species production . Plant Cell , 14 : 3089 – 3099 .
- Umezawa , T , Yoshida , R , Maruyama , K , Yamaguchi-Shinozaki , K and Shinozaki , K . 2004 . SRK2C, a SNF1-related protein kinase 2, improves drought tolerance by controlling stress-responsive gene expression in Arabidopsis thaliana . ProcNatl AcadSciUSA , 101 : 17306 – 17311 .
- Yoshida , R , Hobo , T Ichimura , K . 2002 . ABA-activated SnRK2 protein kinase is required for dehydration stress signaling in Arabidopsis . Plant Cell Physiol , 43 : 1473 – 1483 .
- Hirai , MY , Fujiwara , T , Chino , M and Naito , S . 1995 . Effects of sulfate concentrations on the expression of a soybean seed storage protein gene and its reversibility in transgenic Arabidopsis thaliana . Plant Cell Physiol , 36 : 1331 – 1339 .
- Kim , H , Hirai , MY , Hayashi , H , Chino , M , Naito , S and Fujiwara , T . 1999 . Role of O-acetyl-l-serine in the coordinated regulation of the expression of a soybean seed storage-protein gene by sulfur and nitrogen nutrition . Planta , 209 : 282 – 289 .
- Kuzuhara , Y , Isobe , A , Awazuhara , M , Fujiwara , T and Hayashi , H . 2000 . Glutathione levels in phloem sap of rice plants under sulfur deficient conditions . Soil SciPlant Nutr , 46 : 265 – 270 .
- Ohkama , N , Goto , DB , Fujiwara , T and Naito , S . 2002 . Differential tissue-specific response to sulfate and methionine of a soybean seed storage protein promoter region in transgenic Arabidopsis . Plant Cell Physiol , 43 : 1266 – 1275 .
- Takahashi , H , Yamazaki , M , Sasakura , N , Watanabe , A , Leustek , T , Engler , JD Engler , G . 1997 . Regulation of sulfur assimilation in higher plants: A sulfate transporter induced in sulfate-starved roots plays a central role in Arabidopsis thaliana . ProcNatl AcadSciUSA , 94 : 11102 – 11107 .
- Takahashi , H , Watanabe-Takahashi , A , Smith , FW , Blake-Kalff , M , Hawkesford , MJ and Saito , K . 2000 . The roles of three functional sulphate transporters involved in uptake and translocation of sulphate in Arabidopsis thaliana . Plant J , 23 : 171 – 182 .
- Boudsocq , M , Barbier-Brygoo , H and Lauriere , C . 2004 . Identification of nine sucrose nonfermenting 1-related protein kinases 2 activated by hyperosmotic and saline stresses in Arabidopsis thaliana . JBiolChem , 279 : 41758 – 41766 .
- Kelner , A , Pekala , I , Kaczanowski , S , Muszynska , G , Hardie , DG and Dobrowolska , G . 2004 . Biochemical characterization of the tobacco 42-kD protein kinase activated by osmotic stress . Plant Physiol , 136 : 3255 – 3265 .
- Mikolajczyk , M , Awotunde , OS , Muszynska , G , Klessig , DF and Dobrowolska , G . 2000 . Osmotic stress induces rapid activation of a salicylic acid-induced protein kinase and a homolog of protein kinase ASK1 in tobacco cells . Plant Cell , 12 : 165 – 178 .
- Monks , DE , Aghoram , K , Courtney , PD , DeWald , DB and Dewey , RE . 2001 . Hyperosmotic stress induces the rapid phosphorylation of a soybean phosphatidylinositol transfer protein homolog through activation of the protein kinases SPK1 and SPK2 . Plant Cell , 13 : 1205 – 1219 .
- Anderberg , RJ and Walker-Simmons , MK . 1992 . Isolation of a wheat cDNA clone for an abscisic acid-inducible transcript with homology to protein kinases . ProcNatl AcadSciUSA , 89 : 10183 – 10187 .
- Holappa , LD and Walker-Simmons , MK . 1995 . The wheat abscisic acid-responsive protein kinase mRNA, PKABA1, is up-regulated by dehydration, cold temperature, and osmotic stress . Plant Physiol , 108 : 1203 – 1210 .
- Kobayashi , Y , Yamamoto , S , Minami , H , Kagaya , Y and Hattori , T . 2004 . Differential activation of the rice sucrose nonfermenting1-related protein kinase2 family by hyperosmotic stress and abscisic acid . Plant Cell , 16 : 1163 – 1177 .
- Halford , NG and Hardie , DG . 1998 . SNF1-related protein kinases: global regulators of carbon metabolism in plants? . Plant MolBiol , 37 : 735 – 748 .
- Ohkama-Ohtsu , N , Kasajima , I , Fujiwara , T and Naito , S . 2004 . Isolation and characterization of an Arabidopsis mutant that overaccumulates O-acetyl-l-Ser . Plant Physiol , 136 : 3209 – 3222 .
- Noji , M and Saito , K . 2002 . Molecular and biochemical analysis of serine acetyltransferase and cysteine synthase towards sulfur metabolic engineering in plants . Amino Acids , 22 : 231 – 243 .
- Testerink , C , Dekker , HL Lim , ZY . 2004 . Isolation and identification of phosphatidic acid targets from plants . Plant J , 39 : 527 – 536 .
- Park , YS , Hong , SW , Oh , SA , Kwak , JM , Lee , HH and Nam , HG . 1993 . Two putative protein kinases from Arabidopsis thalianacontain highly acidic domains . Plant MolBiol , 22 : 615 – 624 .
- Present address: 1-1-1 Yayoi, Bunkyo-ku, Tokyo 113-8657, Japan.