Abstract
Tropical peatlands in Sarawak are exploited on a large scale for crop plantations. After reclamation of primary forests, large amounts of gaseous and soluble organic compounds could be released from peatlands. These decomposing products are probably related to the composition and properties of peat humus. Furthermore, these properties might be influenced by land use and topography. Peat soils on the surface and beneath the water table were collected from the edge and the center of a peat dome under sago palm plantations located between two tributaries. The content of alkali-soluble carbon in peat soils and the degree of humification of peat were higher on the edge than in the center of the peat dome and in the deeper horizons compared with the surface horizons. A similar trend was also observed for the contents of carboxylic groups and aromatic constituents in humic acids. The opposite trend was observed for the viscosity of humic acids related to molecular weight. These results indicate that the degree of humification of peat progressed at sites located close to tributaries and in deeper horizons. Peat decomposition might presumably be promoted at locations along the tributaries because of the higher microbial activities supported by a higher mineral nutrient supply. Concurrently, the contents of carbohydrates were lower in the surface horizons than in the deep horizons, suggesting that peat decomposition is accelerated on the ground surface after reclamation. Reclamation of primary forests for the establishment of sago plantations has gradually changed the redox conditions and has promoted biodegradation of peat soils.
INTRODUCTION
Tropical peat has mainly been developed under swamp forests in coastal basin areas and contains fragments of logs and roots originating from woody plant debris (CitationAndriesse 1974). A high water table, that is, a high content of water in peat soil, probably results in the deposition of a large quantity of organic carbon, preventing oxidative degradation of peat. Despite the relatively small distribution of tropical peatlands compared with temperate and boreal peatlands, it has been estimated that tropical peatlands deposit at least 20% of the global peat carbon (CitationGorham 1991). This estimation implies the existence of a fairly high accumulation rate of peat in tropical peatlands. It has been assumed that tropical peat significantly contributed to a large carbon sink in global terrestrial ecosystems. However, tropical peat ecosystems could easily change from a net carbon sink to a carbon source because of artificial or natural disturbances affecting the vegetation and hydrological characteristics, such as forest fire and agricultural use. Therefore, land-use changes in tropical peatlands might be responsible for organic carbon storage both in the present and in the future.
Approximately two-thirds of the tropical peatlands in the world are distributed along the coastal area of the Sunda land shelf in South-East Asia (CitationDriesen 1978). In Sarawak, where tropical peatlands cover 1.7 million hectares, plantations of several crops tolerant to acidity have been established on a large scale despite unfavorable production conditions (CitationAmbak and Melling 2000). It has been assumed that all peatlands in Sarawak could potentially be developed for crop cultivation (CitationChew et al. 2000). Reclamation of primary forest, periodical clearance of wild vegetation and installation of ditches for water drainage are essential in order for tropical peatlands to be used as agricultural fields. The exploitation and management of peatlands for agriculture physically and biologically lead to their deterioration and to the release of dissolved and particulate organic matter. The organic carbon lost from the terrain affects the carbon dynamics in the aquifers and contributes to the increase in atmospheric CO2 by biodegradation in the ecosystems.
The release of dissolved organic matter and CO2 from peat soils after clear cutting and vegetation changes probably depends on the composition and the properties of humus in the soil. The relationships between the biodegradation of dissolved organic carbon and humification indices (CitationKalbitz et al. 2003) and between the decolorization of humic acids by microorganisms and their structural properties (CitationYanagi et al. 2002) have already been documented, although these samples did not include tropical peat soils. Therefore, the decomposition stage of tropical peat soils might be recorded based on the properties of humus. Humus chemical characteristics after land-use changes in tropical peatlands are important for the estimation of carbon release and land degradation.
In the present study, to clarify the effect of land-use changes and topography differences on the humus composition and the properties of humic acids, experimental plots were selected on the transect of a peat dome after reclamation of a primary forest for the establishment of sago palm (Metroxylon sagu L.) plantations. The two sites that were located on the edge of a raised bog were close to tributaries, and the other sites were located between these two sites. The establishment of sago palm plantations is one form of land use that is not associated with damage to tropical peatlands because of the requirement of a relatively higher water table and low frequency of planting, compared with other crops (CitationAmbak and Melling 2000; CitationChew et al. 2000). However, the rather long growth period, approximately 10–15 years (CitationChew et al. 2000), is a distinct disadvantage. However, even such suitable land use may affect peatland degradation, which could be reflected in alterations to humus.
MATERIALS AND METHODS
The study area was located between Mukah and Dalat in Sarawak facing the South China Sea on Borneo Island (). This peatland had developed in the coastal basin. The low contribution of alluvial minerals to the basin area had resulted in deep organic deposits originating from decayed woody materials, and rapid vertical accumulation of peat had led to the formation of a dome-shaped topography, which is typical of tropical peatlands (CitationAndriesse 1974). The soil of the peat dome was oligotrophic because of the lack of mineral supply, except for atmospheric deposition. The mean annual temperature and precipitation observed at the Sibu meteorological station were 26.3°C and 3194 mm, respectively. Based on the climatic data, this area belongs to Af (tropical rain forest climate) using the Köppen climatic classification system.
This region accounts for approximately 60% of the sago-palm-planted area (approximately 7700 ha) on peat soils in Sarawak (CitationTie et al. 1991). In general, this area is covered with deep peat soils (organic soil > 1.5 m in depth). Shallow peat soils (organic soil < 1.5 m in thickness) were also found along the edge of the peat dome. The peat dome was formed between the Oya River and the Igan River, which run within the study area.
Soil surveys and sampling were conducted in August 1999. Soils were collected from the surface and groundwater level at four sites differing in the year of reclamation. Site descriptions and chemical properties cited from the report of CitationKawahigashi et al. (2003) are shown in . The older the reclamation year, the better the average sago growth. However, growth rate did not appear to directly depend on time after reclamation. The groundwater level measured in August, which did not exhibit appreciable seasonal variation in the sago plantation fields (CitationMelling et al. 2005), was different at each site. The position of groundwater level in the center of the dome was higher than those at the edges of the dome, which displays a relatively low water level because of the presence of a ditch for drainage. Shallow peat soils containing sulfidic materials below a depth of 40 cm were found in the coastal area of the river. After the removal of visible plant materials and excessive soil water by centrifugation at 1800 g, the soils were freeze-dried.
Humic solutions were extracted from the freeze-dried peat soils by end-over-end shaking extraction under a N2 atmosphere at 25°C for 24 h with 0.1 mol L−1 NaOH, corresponding to three hundredth of the soil carbon content. The extracts obtained after two extractions were combined and separated from the soil residues by centrifugation at 11,000 g for 15 min. The extracts were acidified with 1 mol L−1 HCl to pH 1.5. After centrifugation of the acidified extracts at 11,000 g for 30 min, the humic acid and fulvic acid fractions were obtained as precipitates and supernatants, respectively.
Preparation of the precipitates was carried out by removing salts and fulvic acids using repeated dissolution with dil. NaOH and precipitation with dil. HCl and dialysis to distilled water under acidic conditions (pH < 1.5), according to the method of CitationKawahigashi et al. (1998). After preparation, the humic acids appeared as a powder by freeze-drying.
Table 1 General information about peat soils and experimental sites
The amount of total and inorganic carbon in the alkaline extracts and fulvic acid solutions was determined using TOC-5000 (Shimadzu, Kyoto, Japan). The amount of organic carbon in each solution was determined by subtraction of the amount of inorganic carbon from that of total carbon. The amount of organic carbon in the humic acid fraction was calculated from the difference between the amount in the extracts and the fulvic acid solutions. Humus composition was calculated from the proportion of carbon contained in the fulvic and humic acid fractions. The amount of humin carbon was calculated as the difference in the carbon content between the extracts and the total carbon in soils.
The contents of carboxylic groups and phenolic groups in the humic acids were determined by non-aqueous titration according to the method of CitationYonebayashi and Hattori (1985). A 5 mg aliquot of humic acid was dissolved in 20 mL of dimethyl sulfoxide in a reaction cell. The humic acid solution was titrated under a N2 atmosphere with 0.05 mol L−1 sodium methoxide in a benzene : methanol (1:3) solution. Benzoic acid and phenol were used as internal standards for the determination of the contents of carboxylic acids and phenolic acids, respectively.
The 1H nuclear magnetic resonance (NMR) spectra of the humic acids were recorded using a JEOL 270EX spectrometer (JEOL, Tokyo, Japan) at 270.05 MHz under homo-gated decoupling conditions with irradiation of the HOD peak at 4.6 p.p.m. to remove water impurities. Two hundred scans were accumulated for each humic acid. Conditions for 1H NMR were as follows: acquisition time, 3.018 s; pulse delay, 5.0 s; line broadening factor, 0.5 Hz. A 5 mg aliquot of humic acid was dissolved in 3 mL of a 1 mol L−1 NaOH solution. The sodium salt of the humic acid was evaporated and the humic acid was desiccated to remove the moisture using NaOH and P2O5 under vacuum. The humic acid redissolved in D2O (99.99% D) was transferred to a 5 mm NMR tube. The recorded 1H NMR spectra were divided into five signal regions: aromatic H, 6.0–9.0 p.p.m.; carbohydrate H, 3.0–4.3 p.p.m.; aliphatic Hα, 1.6–3.0 p.p.m.; aliphatic Hβ, 0.9–1.6 p.p.m.; aliphatic Hγ, 0.6–0.9 p.p.m. (CitationKawahigashi et al. 1996). The signal area for each type of hydrogen was calculated using electronic integration.
Intrinsic viscosity was determined using the extrapolation method for reduced viscosity depending on the concentration (CitationKawahigashi and Fujitake 2001). A 5 mL aliquot of 10 g L−1 humic acids adjusted to pH 7.0 and 0.1 mol L−1 NaCl concentration were prepared according to the method of CitationKawahigashi and Fujitake (2001). Reduced viscosity was measured using an Ubbelohde-type viscometer with a flow time of 450 s for distilled water at 25 ± 0.01°C. Concentration of the solution was changed successively by the addition of a suitable volume of buffer solution (0.1 mol L−1 Na4P2O7−0.1 mol L−1 H4P2O7, pH 7.0) containing 0.1 mol L−1 NaCl and the viscosity measurement was carried out over a wide range of concentrations from 1 to 10 g L−1. Data were treated as previously described (CitationKawahigashi and Fujitake 2001).
The degree of humification of peat was determined according to the method of CitationSchnitzer and Desjardins (1965). Extracts from peat samples using one hundred times the volume of a 0.025 mol L−1 sodium pyrophosphate solution were obtained. After adequate dilution with distilled water, the absorbance of the solution at 550 nm was recorded and multiplied by 100 to obtain the index.
Linear regression analyses between the analyzed properties were calculated and evaluated. Significant differences between the average values in surface peat and deep peat were also tested. The statistical analyses were conducted using SPSS 10.0 J for Windows (SPSS Japan, Tokyo, Japan).
RESULTS
Degree of humification
The degree of humification was significantly (P < 0.05) lower in the surface horizons than in the deep horizons (). At both depths, sites 2 and 3, which were close to the center of the dome, showed a lower degree of humification of peat than sites 1 and 4. The degree of humification of peat was significantly correlated (r = 0.75, P < 0.05) with the concentration of carbon in the alkaline extracts.
Humus composition
At all sites, the content of alkali-soluble carbon was significantly (P < 0.05) lower in the surface horizons than in the deep horizons (). The proportions of alkali-soluble carbon ranged from 35% to 44% in the surface horizons and from 38% to 46% in the deep horizons. Among the sites, the proportions were the lowest at site 2 and the highest at site 4 in both horizons. In the extracts, the proportions of fulvic acids,
which were much lower than those of humic acids, were similar among the sites at both depths. The proportions of alkali-soluble carbon were highly correlated with those of extracted humic acids (r = 0.96, P < 0.001). The order of the proportion of humic acids was as follows: site 4 > 1 > 3 > 2 in the surface horizons and site 4 > 3 > 1 > 2 in the deep horizons.Functional groups of humic acids
Contents of carboxylic groups, which ranged between 2.4 and 3.4 mmolc g−1 humic acids, were lower in the surface horizons than in the deep horizons (). In both horizons, the contents of carboxylic groups showed a significant correlation with those of alkali-soluble carbon (r = 0.95, P < 0.001) and the degree of humification of peat (r = 0.83, P < 0.05). In contrast, the contents of phenolic hydroxyl groups, which were approximately one-fifth of those of the carboxylic groups, did not show any relationships with the parameters of humus composition.
1H NMR spectra of humic acids
The contribution of aromatic H to the 1H NMR spectra was the smallest of all the humic acids (, ). The proportion of aromatic H in the surface horizons was significantly (P < 0.001) lower than that in the deep horizons at each site. The order of the proportion of aromatic H in both horizons was as follows: site 4 > 1 > 3 > 2, which was the same as the degree of humification of peat. The rate of aromatic H showed a significant correlation with the rate of humic acids (r = 0.87, P < 0.01), the degree of humification of peat (r = 0.95, P < 0.01) and the contents of carboxylic groups (r = 0.92, P < 0.01). Aliphatic H accounted for between 41% and 44% in the surface horizons and between 31% and 35% in the deep horizons. The contribution of aliphatic H in the surface horizons was significantly (P < 0.001) greater than in the deep horizons at each site. Based on the composition of aliphatic H, sites 1 and 4 contained a large proportion of long alkyl chains with a higher rate of aliphatic Hβ than sites 2 and 3. The contribution of carbohydrate H was significantly (P < 0.001) greater in the deep horizons than in the surface horizons. The order of the proportion of carbohydrate H in both horizons was opposite to that of aromatic H.
Viscosity of humic acids
The [η] of humic acids, which is a physical property highly related to the molecular weight, were significantly (P < 0.05) higher in the surface horizons than in the deep horizons (). The order of the values in both horizons was as follows: site 2 > 3 > 1 > 4. The values of [η] showed a significant negative correlation with the degree of humification of peat (r = −0.86, P < 0.01), the amount of aromatic H (r = −0.92, P < 0.01) and the contents of carboxylic groups (r = −0.85, P < 0.01).
Figure 4 Contents of (a) carboxylic and (b) phenolic groups in humic acids at each depth and at each site.
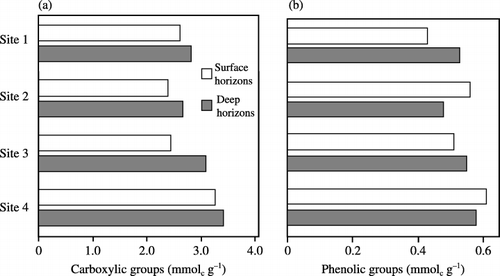
DISCUSSION
Influence of topography on humification in deep peat soils
Although the ash contents were extremely low in all peat soils (), those in the surface horizons were higher on the edge of the peat dome than in the center of the peat dome. It is likely that the tributaries bring a large amount of minerals to peatlands along the coast. The ash content, which is responsible for the supply of mineral nutrients to microorganisms, was closely related to the biodegradability of tropical peat soils (CitationMurayama and Bakar 1996). Cation contents and their composition influenced the biodegradation of peat soils accumulated in the surface horizons (CitationThomas and Pearce 2004). Concurrently, the degree of humification of peat increased during the biodegradation processes of humus (CitationYanagi et al. 2002; CitationKalbitz et al. 2003). The properties of the humic acids on the edge of the peat dome, which showed a high degree of humification of peat, a large proportion of aromatic H, a high amount of carboxyl groups and a low molecular weight, might be attributed to the higher biodegradability, which in
turn was ascribed to the higher ash content along the tributaries. However, these humic properties in the deep horizons were not related to the ash content. In the deep horizons, the degree of humification of peat might not be influenced by the present nutritional conditions but by previous conditions. Differences in the decomposition rate of boreal peat between surface and deep horizons were also reported under anoxic conditions (CitationThomas and Pearce 2004).The amount of alkali-soluble carbon in the extracts from the surface horizons was greater on the edge of the peat dome, presumably because of the contents of acidic functional groups and because an alkaline solution was used as an extractant. The content of carboxylic groups are higher in humic acids with a high humification index (CitationTsutsuki and Kuwatsuka 1978; CitationYonebayashi and Hattori 1988), and this was also observed in the present study as evidenced by the significant correlation between the contents of carboxylic acids and the degree of humification of peat. The relatively aerobic conditions in the surface horizons led to the production of carboxylic acids from aldehyde lignin derivatives by fungal degradation (CitationHatcher and Minard 1995; CitationHedges et al. 1988). The differences in the contents of carboxylic groups among the sites, therefore, might depend on microbial activity, which could also be responsible for biodegradation of carbohydrates. The higher the contents of carboxylic groups, the lower the rates of carbohydrate H. Peat soils on the edge of the peat dome could be considerably biodegraded because of the high ash content. The ash content responsible for nutrient supply in peatlands presumably influenced the biodegradation and/or the degree of humification of peat at each site.
The negative correlation between the viscosity and the degree of humification of peat, the rates of aromatic H and the contents of carboxylic acids indicated that humic acids with a higher molecular weight contained large amounts of colorless biochemical molecules without a charge, such as lipids, proteins and carbohydrates (CitationTomikawa and Oba 1991; CitationTsutsuki and Kuwatsuka 1984). These biochemical molecules are mainly supplied from fresh litter, twigs, dead roots and microorganisms. The lower biodegradability in the center of the peat dome, where humic acids showed a higher viscosity, enables the storage of large amounts of biochemical molecules. Aliphatic Hβ, the presence of which probably resulted from the long methylene chains, could be a constituent of lipids. In the composition of aliphatic H, humic acids in the center of the peat dome showed a lower rate of aliphatic Hβ, indicating that these humic acids contained shorter alkyl chains with a branched structure. In contrast, the long methylene chains were probably the predominant aliphatic constituents of the humic acids on the edge of the dome. CitationTulloch (1976) and CitationWeete (1976) confirmed that long and short alkyl chains composing lipids originated from higher plants and algae, respectively. The microbial habitats in the center of the peat dome, based on the lipid composition, suggest that the conditions were more reductive than those on the edge of the peat dome.
The reciprocal trend between the contents of carbohydrate H and aliphatic H or aromatic H along the topography may be related to their biodegradability. Aliphatic and aromatic constituents could be resistant to biodegradation, compared with carbohydrates (CitationSchnitzer 1991). Relatively lower rates of aliphatic H in the deep horizons than in the surface horizons might result from the greater contribution of aromatic H related to the higher humification degree in the deep horizons. Biodegradation that is likely to be accompanied by humification of peat should strongly influence the composition of organic components in humic acids.
Reclamation and humus decomposition
The properties of the humic acids and the humus composition at each site indicated that the progression of humification of peat was more conspicuous in the deep horizons than in the surface horizons. That is, the deeper and older the soil horizons, the higher the degree of humification of peat. In the surface horizons, however, the proportions of carbohydrate H in the humic acid fractions were smaller than those in the deep horizons, despite the lower degree of humification of peat. Thus, it can be assumed that the oxidative decomposition of carbohydrates in peat is faster in the surface horizons. A rapid decrease in the carbohydrates in the humic acid fraction from tropical peat soils was also observed after forest reclamation (CitationYonebayashi et al. 1994). The lower amount of total carbon in each surface horizon might also be consistent with the difference in the amount of carbohydrates with depth. Oxidation of surface peatlands on a large scale has probably taken place after reclamation and drainage. The oxidation leads to peat shrinkage and land subsidence. CitationTie and Kueh (1979) reported the existence of a subsidence of 60 cm for a drained peat in the first 2 years after reclamation, followed by a rate of 6 cm per annum.
Based on our experimental results, peat shrinkage has not occurred yet, as evidenced by the bulk density (CitationKawahigashi et al. 2003). Sago palm plantations are not associated with appreciable damage to peatlands because of the higher water table requirement compared with other crops, such as oil palm, pineapple, groundnut and maize (CitationAmbak and Melling 2000; CitationChew 2000). For most agricultural uses of peatlands, however, periodical clearance of wild vegetation, burning of peatlands to alleviate sprouts and removal of logs after reclamation are essential practices (CitationAmbak and Melling 2000). Installation of a canal for water table management is also important. These agricultural practices are likely to lead to oxidative conditions and degradation of peat. A decrease in the amount of carbohydrates in the humic acid fraction in the surface horizons may indicate the presence of oxidation and land degradation. Further oxidative degradation of peat might result in the induction of acid sulfate soils in the marginal zone of a peat dome. We confirmed the presence of acid sulfate soils in the plot close to site 4 (CitationKawahigashi et al. 2003).
Analysis of organic matter in tropical peatlands as well as monitoring of the water table and nutrient requirements should be conducted for peatland management. Organic matter on the peatland surface is highly responsible for the water environment in and around the ecosystem, particularly after reclamation and planting of crops because of the loss of the canopy and vegetation cover. Labile organic constituents that are released with a change in land use in tropical peatlands play an important role in greenhouse gas emissions (CitationHadi et al. 2001).
CONCLUSION
Humus composition and the properties of humic acids in deep peat soils were affected by the topography in the surface horizons as well as in the deep horizons below the water table. The peat soils on the edge of the peat dome underwent a higher degree of biodegradation, presumably because of the higher nutrient supply and gradual oxidative conditions, than those in the center of the dome. Land use changes, even if land exploitation is likely to be associated with less degradation, might induce a remarkable oxidative biodegradation of the surface peat soils along with peat shrinkage, land subsidence, release of dissolved and particulate organic matter, and further greenhouse gas emissions. Properties of humus in peat soils corresponding to the topography should be taken into account for the exploitation of deep peat. Investigations of the relationship among the properties of humus in a peat soil according to topography, record of land use changes and hydrological management of arable land may allow us to estimate the degree of land degradation and to anticipate the emergence of acid sulfate soils, which may preclude their use in fields for the cultivation of crops.
ACKNOWLEDGMENTS
This study was partly supported by the fund for research on tropical bioresources from the Japanese Society for the Promotion of Science (JSPS). We thank the staff members of the Land Custody and Development Authority for their assistance in soil sampling in the field and for supplying information about the land use and management. We are also grateful to Dr H. Tanaka and Dr C. K. Yamaguchi for their suggestions and discussions.
REFERENCES
- Andriesse , JP . 1974 . The characteristics, agricultural potential and reclamation problems of tropical lowland peats in South-East Asia , Amsterdam : Royal Tropical Institute .
- Gorham , E . 1991 . Northern peatlands – Role in the carbon-cycle and probable responses to climatic warming . Ecological Appl , 1 : 182 – 195 .
- Driesen , PM . 1978 . “ Peat soil ” . In Soil and Rice , 763 – 779 . Ros Baños : IRRI .
- Ambak , K and Melling , L . Management practices for sustainable cultivation of crop plants on tropical peatland . Proc. of the International Symposium of Tropical Peatland . November 22–23 1999 , Bogor, Indonesia. pp. 119 – 134 .
- Chew , TA , Abu Hassan , I and Mohayidin , MG . 2000 . “ Estimating the environmental benefits of sago cultivation ” . In Repositioning of the Agriculture Industry in the Next Millenium , Edited by: Radam , A and Arshad , FM . 235 – 251 . Universiti Putra Malaysia Sedang Selagor .
- Kalbitz , K , Schmerwitz , J , Schwesig , D and Matzner , E . 2003 . Biodegradation of soil-derived organic matter as related to its properties . Geoderma , 113 : 273 – 291 .
- Yanagi , Y , Tamaki , H , Otsuka , H and Fujitake , N . 2002 . Comparison of decolorization by microorganisms of humic acids with different 13C NMR properties . Soil BiolBiochem , 34 : 729 – 731 .
- Tie , TL , Loi , KS and Lim , ET . The geographical distribution of sago (Metroxylonspp.) and the dominant sago-growing soils in Sarawak, InTowards Greater Advancement of Sago Industry in the 90s . Proceedings of the 4th International Sago Symposium . August 6–9 1990 , Kuching, Sarawak, Malaysia. pp. 36 – 45 .
- Kawahigashi , K , Sumida , H , Yamamoto , K , Tanaka , H and Yamaguchi , CK . 2003 . Chemical properties of tropical peat soils and peat soil solutions in sago palm plantation . Sago Palm , 1 : 55 – 63 .
- Melling , L , Hatano , R and Goh , KJ . 2005 . Methane fluxes from three ecosystems in tropical peatland of Sarawak, Malaysia . Soil BiolBiochem , 37 : 1445 – 1453 .
- Kawahigashi , M and Fujitake , N . 1998 . Surface-active properties of particle size fractions in two humic acids . Soil SciPlant Nutr , 44 : 497 – 505 .
- Yonebayashi , K and Hattori , T . 1985 . Nonaqueous titration of functional groups in humic acid . OrgGeochem , 8 : 47 – 54 .
- Kawahigashi , M , Fujitake , N and Takahashi , T . 1996 . Structural information obtained from spectral analysis (UV-VIS, IR, 1H NMR) of particle size fractions in two humic acids . Soil SciPlant Nutr , 42 : 355 – 360 .
- Kawahigashi , M and Fujitake , N . 2001 . Relationship between viscosity and molecular weight in an Andosol humic acid . Soil Sci Plant Nutri , 47 : 399 – 404 .
- Schnitzer , M and Desjardins , JG . 1965 . Carboxyl and phenolic hydroxyl groups in some organic soils and their relation to the degree of humification . CanJSoil Sci , 45 : 257 – 264 .
- Murayama , S and Bakar , ZA . 1996 . Decomposition of tropical peat soils 2. Estimation of in situ decomposition by measurement of CO2flux Japan International Research Center for Agricultural Sciences Ministry of Agriculture, Forestry and Fisheries, Tsukuba . JARQ , 30 : 145 – 151 .
- Thomas , PA and Pearce , DME . 2004 . Role of cation exchange in preventing the decay of anoxic deep bog peat . Soil BiolBiochem , 36 : 23 – 32 .
- Tsutsuki , K and Kuwatsuka , S . 1978 . Chemical studies on soil humic acids: II. Composition of oxygen-containing functional groups of humic acids . Soil SciPlant Nutr , 24 : 547 – 560 .
- Yonebayashi , K and Hattori , T . 1988 . Chemical and biological studies on environmental humic acids. I. Composition of elemental and functional groups of humic acids . Soil SciPlant Nutr , 34 : 571 – 584 .
- Hatcher , PG and Minard , RD . 1995 . Comment on the origin of benzenecarboxylic acids in pyrolysis methylation studies . OrgGeochem , 23 : 991 – 994 .
- Hedges , JI , Blanchette , RA , Weliky , K and Devol , AH . 1988 . Effects of fungal degradation on the CuO oxidation products of lignin: A controlled laboratory study . GeochimCosmochimActa , 52 : 2717 – 2726 .
- Tomikawa , A and Oba , Y . 1991 . Characteristics of soil humic substances fractionated in relation to particle weight VI. Particle weight distribution, optical properties, and infrared absorption spectra of fractions of humic acids with different particle weights . Soil SciPlant Nutr , 37 : 211 – 221 .
- Tsutsuki , K and Kuwatsuka , S . 1984 . Molecular size distribution of humic acids affected by the ionic strength and the degree of humification . Soil SciPlant Nutr , 30 : 151 – 162 .
- Tulloch , AP . 1976 . “ Chemistry of waxes of higher plants ” . In Chemistry and Biochemistry of natural waxes , Edited by: Kolattukudy , PE . 235 – 287 . Amsterdam : Elsevier .
- Weete , JE . 1976 . “ Algal and fungal waxes ” . In Chemistry and Biochemistry of natural waxes , Edited by: Kolattukudy , PE . 349 – 418 . Amsterdam : Elsevier .
- Schnitzer , M . 1991 . Soil organic matter – The next 75 years . Soil Sci , 151 : 41 – 58 .
- Yonebayashi , K , Pechayapisit , J , Vijarnsorn , P , Zahari , AB and Kyuma , K . 1994 . Chemical alterations of tropical peat soils determined by Waksman's proximate analysis and properties of humic acids . Soil SciPlant Nutr , 40 : 435 – 444 .
- Tie , YL and Kueh , HS . 1979 . A review of lowland organic soils of Sarawak , Technical paper No. 4 Sarawak, , Malaysia : Research Branch, Department of Agriculture .
- Hadi , A , Haridi , M , Inubushi , K , Purnomo , E , Razie , F and Tsuruta , H . 2001 . Effects of land-use change in tropical peat soils on the microbial population and emission of greenhouse gases . Microbes Environ , 16 : 79 – 86 .