Abstract
To examine the relationships between soil age and the structural stability and natural 13C abundance (δ13C) of humic acids, buried humic layers in volcanic ash soil profiles near Mt Fuji, Japan, namely Yubunebara (present to 10,000 years before present (ybp); n = 5) and Ashitaka-Onoue (<1,000–30,000 ybp; n = 6), were analyzed. The contents and δ13C values of the fulvic acids (humic substances) and non-humic substances in the fulvic acid fractions were also determined. The degree of humification of the humic acids differed remarkably between the surface and buried layers, and the A 600/C value (absorbance at 600 nm mg−1 C mL−1 of humic acids in 0.1 mol L−1 NaOH) increased toward the older buried layers. The proportion of aromatic C in total C of the humic acids, estimated using cross polarization/magic angle spinning 13C nuclear magnetic resonance spectroscopy, also increased, whereas the proportions of alkyl and O-alkyl C decreased from the surface toward the older layers. These observations suggested higher stability in the aromatic than aliphatic components and the contribution of aromatic C to the increase in the A 600/C value. The composition of the C functional groups did not vary appreciably among layers older than 10,000 ybp. The δ13C value of bulk soil organic matter (SOM) fluctuated in both profiles, suggesting that the proportions of C3 and C4 plants grown when each layer was the surface layer differed among the layers. Although, in general, variation in the δ13C value of each humus fraction corresponded to that of bulk SOM, there were significant differences in the δ13C value between the humus fractions and bulk SOM, and the differences were more conspicuous in the younger layers and less conspicuous in the older layers. A negative correlation (with an exception) was also observed between the difference in the δ13C values of humic acids from that of bulk SOM and the relative content of aromatic C in the humic acids. Possible causes of the differences and changes in the δ13C values are discussed.
INTRODUCTION
Humic substances are defined as dark-colored amorphous polymers that were synthesized from biomass constituents or their metabolites biochemically and/or chemically in the environment (CitationStevenson 1994). It has been considered that initial humic substances lose their components that are relatively more easily decomposed, while condensation–polymerization and oxidative reactions proceed, which results in a more stable structure (CitationKumada 1987). These processes correspond to the progression of humification and are frequently evaluated based on an increase in the degree of darkening, particularly for soil humic acids, the alkali-soluble and acid-insoluble fraction. The degree of humification may enable us to predict the stability of humic acids in soil. However, detailed structural changes throughout the humification process have not been sufficiently elucidated, partly because of the long period of time required to trace them. For example, CitationOtsuka et al. (1994) estimated that the duration of the period required for the chemical formation of Type A humic acids, the highest class in terms of the degree of humification, was approximately 150–2,000 years, based on the incubation of plant residues with volcanic ash at 75°C and 90°C. Although it is difficult to determine the applicability to natural soil of the results they obtained at high temperatures in the absence of microbial activity, other data also indicated that humic acids in volcanic ash soils (Melanudands) approximately 50 and 240 years after ash fall belonged to Types P0 and B, intermediate classes in terms of the degree of humification (CitationKumada 1987).
A comparison of humic acids in soils of different ages has been used to analyze the long-term process of humification (CitationKumada 1987; CitationOtsuka and Kumada 1978; CitationSakai and Kumada 1982). In Japan, more than two buried humic layers that have developed in deposits of volcanic materials are sometimes observed in a soil profile. The soil age could be estimated from the presence of age-known volcanic ash or 14C measurement. Direct comparison among the layers is impossible if there is no evidence showing that climatic conditions and the type and amount of vegetation are identical among the layers. However, such soil profiles are better, as samples, to assume the diagenesis of humic acids than soils collected from different sites, because the influence of geological differences could be ignored, particularly in plains. Based on the analysis of published data on humic acids in several volcanic ash soil profiles, CitationKumada (1987) suggested that the increase in the degree of humification, the changes in elemental composition and the formation of a graphitic structure were likely to be almost complete within 1,000–3,000 years. Variations in more detailed structural characteristics, such as C functional groups, have not been determined.
Natural abundance of 13C, which is generally expressed as δ13C in unit of per mil () using Eq. Equation1, is a signature providing information about the origin and production process of each organic material (CitationBarrie and Prosser 1996):
where R is the molar ratio 13C/12C and standard refers to the Cretaceous carbonate fossil Bellemnitella americana from the Peedee Formations in South Carolina, USA. Variations in δ13C values are derived from differences in the rates between isotopes during the physical, chemical and biological processes. The δ13C value of plant cells varies depending on their photosynthetic pathway, C3 or C4 (CitationBender 1971; CitationSmith and Epstein 1971), which is reflected on the δ13C value of soil organic matter (SOM) where respective types of plants are grown (CitationBalesdent et al. 1987; CitationSchwartz et al. 1986). Even in soil profiles under constant vegetation historically, the increase in the δ13C value of bulk SOM (up to 5) with increasing depth or 14C age has been observed through horizons (CitationAgnelli et al. 2002; CitationBol et al. 1999; CitationHuang et al. 1996), suggesting a positive effect of diagenetic processes on 13C enrichment. Although the cause of the increase in the δ13C value has not been elucidated and there are few data indicating the chronological changes in δ13C values of humic acids (CitationAgnelli et al. 2002), the δ13C value could become a key index in the elucidation of long-term humification processes.
In the present study, the diagenesis of humic acids toward the development of a more stable structure was investigated by comparing the composition of C functional groups, estimated by solid state 13C nuclear magnetic resonance (NMR) spectroscopy, and the degree of humification among buried humic layers with different soil ages using volcanic ash soil profiles near Mt Fuji, Japan. In addition, the relationships between soil age or the structural properties of the humic acids and their δ13C values were analyzed. To obtain supplementary information, the content and δ13C values were also determined for bulk SOM and for the humic and non-humic substances in the fulvic acid fractions.
MATERIALS AND METHODS
Soil samples
Soil samples collected from one Yubunebara (Gotenba, Shizuoka, Japan) and two Ashitaka-Onoue (Numazu, Shizuoka, Japan) soil profiles were used. Detailed descriptions of the soil profiles were presented in the reports of CitationYoshida et al. (1978) and CitationTsutsuki and Kuwatsuka (1989), respectively. Layer designations were also referred to in these reports. Five humic layers in the Yubunebara profile, including the surface layer (Yu-1), a sub-layer aged 1140–1200 years before present (ybp; Yu-2), and three sub-layers that were assumed to have accumulated at ca. 3,000 (Yu-3), 5,000 (Yu-5) and 10,000 ybp (Yu-7) were used. From the Ashitaka-Onoue profile, six humic layers with approximate ages of <1,000 (At-A), 8,000 (At-D), 18,000 (At-4), 27,200 (At-8), 28,100 (At-15) and 30,000 (At-20) ybp were selected.
Analysis of humus composition
Total C content in the soil samples was determined using a NC analyzer, Sumigraph NC-800 (Sumika Chemical Analysis Service, Osaka, Japan). Humic and fulvic acid fractions were extracted according to the NAGOYA method (CitationIkeya and Watanabe 2003; CitationKuwatsuka et al. 1992) by continuous shaking with 0.1 mol L−1 NaOH (300 mL g−1 soil C) at 25oC for 24 h under N2. Purification procedures to obtain powder samples of humic acids also followed the NAGOYA method.
The fulvic acid fraction obtained as an acidic supernatant after precipitation of humic acids in the extract by acidification (pH < 1.5) was then fractionated, using a column packed with cross-linked insoluble polyvinylpyrrolidone (PVP; “Polyclar SB100”, Gokyo Sangyo, Osaka, Japan), into adsorbed and non-adsorbed fractions according to the method of CitationWatanabe and Kuwatsuka (1991). Because the humic and non-humic substances in the fulvic acid fractions were concentrated in PVP-adsorbed and non-adsorbed fractions, respectively (CitationWatanabe and Kuwatsuka 1991, Citation1992), these two fractions were designated as fulvic acids and water-soluble non-humic substances (WS-NHS) in the present study. The organic C concentration was determined for a portion of these two fractions using a dissolved C analyzer, TOC-VCPH (Shimadzu, Kyoto, Japan), under acidic conditions after bubbling with N2. Another portion of each fraction was allowed to dry on a hot plate at pH 3–4 and was mixed thoroughly in an agate mortar.
The logarithmic value of the ratio of the absorbances at 400 and 600 nm of humic acids in 0.1 mol L−1 NaOH, log(A 400/A 600), and the absorbance at 600 nm mg−1 C mL−1, A 600/C, were determined to express the degree of humification. The organic C concentration was determined using the TOC-VCPH (CitationIkeya and Watanabe 2003), and the absorbances were measured with a spectrophotometer, UV-2200 (Shimadzu).
All the above procedures were conducted in duplicate.
Measurement of solid state 13C NMR spectra
Solid state 13C NMR spectra of the humic acids were recorded at 75.6 MHz on a Chemagnetic CMX-300 spectrometer (Varian, Palo Alto, CA, USA) using the cross polarization/magic angle spinning (CPMAS) method under the following conditions: spinning rate, 5000 Hz; contact time, 1 msec; pulse delay time, 0.7 s; line broadening, 50 Hz. Accumulation was repeated 1,000–2,000 times. The spectra were divided into four regions, 0–45, 45–110, 110–160 and 160–190 ppm, and the relative area of each region in the total spectral area was determined. These four regions were represented by the major C species appearing in each region (i.e. saturated alkyl C, alkyl C substituted with hetero atom [O-alkyl C], aromatic C and carbonyl C from higher toward lower magnetic fields). The spinning side bands of the signals with maximums at approximately 130, 150 and 170 ppm were detected at approximately 200, 220 and 240 ppm, respectively. The counter side bands occurring at approximately 60, 80 and 100 ppm overlapped with the signals of O-alkyl C. For the correction, the sum of the areas for the peaks at 200, 220 and 240 ppm was subtracted from the area of O-alkyl C, while twice the area for the former two peaks and for the latter peak were added to the area of aromatic C and carbonyl C, respectively (CitationMonteil-Rivera et al. 2000).
Measurement of δ13C
The δ13C values of the powder samples of the three humus fractions as well as the pulverized soil samples were measured using a stable isotopic ratio mass spectrometer connected to an elemental analyzer (DELTA Plus-NC2500, Thermo Finnigan, San Jose, CA, USA). The experimental error was ±0.1 . The relationship and significance of the difference between the δ13C value of bulk SOM and each humus fraction were analyzed using a regression analysis and t-test, respectively.
RESULTS
Humus composition
shows the total C content in the soil samples and the proportions of the three humus fractions in total SOM on a C basis. Large decreases in the total C content were observed from the Yu-1 to Yu-3 samples in the Yubunebara profile () and between the At-A and At-D samples in the Ashitaka-Onoue profiles (). According to CitationTsutsuki and Kuwatsuka (1989), there were two humic layers designated as At-B and At-C (2,100 and 3,500 ybp of age, respectively) between At-A and At-D, and total C content decreased conspicuously between the two layers.
The proportion of humic acids in total SOM was in the range of 16.2–31.9% () and did not show a relationship with soil age. The proportion of fulvic acids in total SOM tended to be greater in the upper two layers (7.0–7.2% or 8.3–10.5%) than in the lower layers (2.0–2.8% or 5.1–6.3%) within the respective Yubunebara and Ashitaka-Onoue profiles. A similar trend was also observed for WS-NHS, namely 16.7–18.0% versus 5.6–7.8% in the Yubunebara and 16.5–17.2% versus 5.6–10.4% in the Ashitaka-Onoue profiles.
The difference in the degree of humification of the humic acids () was remarkable between the Yu-1 and Yu-2 samples. The Yu-1 humic acids were classified into Type B and the others belonged to Type A (CitationIkeya and Watanabe 2003; CitationKumada 1987). The A 600/C value of the humic acids in the buried layers appeared to increase gently with increasing soil age up to ca. 10,000 ybp.
13C CPMAS NMR spectra of humic acids
Changes in the 13C CPMAS NMR spectra were distinct from the Yu-1 to Yu-3 humic acids and between the At-A and At-D humic acids, respectively (, ). The resolution of the signals assigned to the methoxyl groups (54.0–55.9 ppm) and aromatic C substituted with hetero atom (151.5–153.8 ppm) was higher in the spectra of the younger samples, Yu-1, Yu-2 and At-A humic acids. The relative intensity of the signals of alkyl C with the maximum at 22.6–32.5 ppm, secondary alcohol (71.9–73.1 ppm) and acetal (103.3–105.7 ppm) was also greater. The difference in the signal intensity in each region between the older samples could be distinguished after correction of the influence of spinning side bands ().
Figure 1 Total carbon (C) content in (a) Yubunabara and (b) Ashitaka-Onoue soils and the proportions of the three humus fractions in total soil organic matter on a C basis in (c) Yubunebara and (d) Ashitaka-Onoue soils. (•), humic acids; (▴), fulvic acids (PVP-adsorbed fraction of fulvic acid fraction); (▵), water-soluble non-humic substances (PVP-non-adsorbed fraction of fulvic acid fraction).
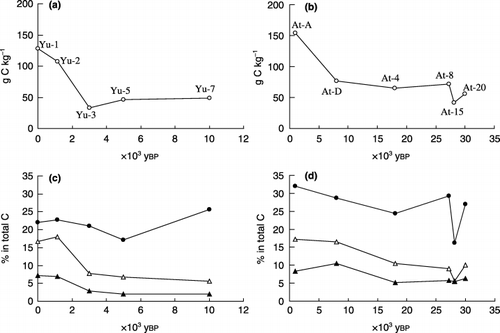
The proportion of aromatic C in total C estimated from the 13C CPMAS NMR spectra () increased from the Yu-1 (31.1%) to Yu-7 humic acids (66.7%) and from the At-A (45.4%) to the At-8 humic acids (68.5%), which corresponded to the decrease in the proportions of alkyl and O-alkyl C. A proportion of aromatic C above 63% was observed for all the buried layers aged 10,000 ybp (Yu-7) and older. The variations in the proportions of carbonyl C were small among all the humic acids and ranged between 15.7% (Yu-1) and 19.9% (At-D).
δ13C value of each humus fraction
The δ13C value of bulk SOM fluctuated both in the Yubunebara and Ashitaka-Onoue profiles (), suggesting that the proportions of C3 and C4 plants grown when each layer was the surface layer differed among the layers. In general, variations in the δ13C value of each humus fraction corresponded to that of bulk SOM (). In the regression analysis, the δ13C values of the three humus fractions were positively correlated with that of bulk SOM (n = 11; r = 0.835–0.896; P < 0.005). The δ13C values of the humic acids, fulvic acids and WS-NHS ranged between −17.4 and −23.2, −19.8 and −25.0 and −16.1 and −20.8, respectively. The δ13C values of the humus fractions in the surface soils were higher in the order, WS-NHS, humic acids and fulvic acids, with >0.9 difference between them. This was also applicable to younger buried layers from Yu-2 to Yu-5, whereas the δ13C values of the fulvic acids were higher than those of the humic acids, with >0.5 differences in the Yu-7, At-4, At-8 and At-15 layers.
DISCUSSION
The lower C content in the older buried layers does not necessarily indicate that C decreased with time because the initial C content before burial may have differed among the layers. However, the smaller proportions of WS-NHS, which frequently contain polysaccharides and peptides/proteinaceous materials as major components (CitationAnderson et al. 1989; CitationCheshire et al. 1992; CitationWatanabe and Kuwatsuka 1992), in total SOM and those of alkyl and O-alkyl C in humic acid C in the older layers, suggested the loss of components that are relatively more easily decomposed in humus after burial. The proportions of alkyl C and O-alkyl C in total C for the humic acids in the buried layers aged 10,000 ybp and older, 2.3–3.1% and 9.9–14.7%, respectively, also tended to be smaller than the values for Type A humic
Figure 2 Degree of humification of humic acids in (a) Yubunebara and (b) Ashitaka-Onoue soils. (▪), A600/C; (□), log(A400/A600).
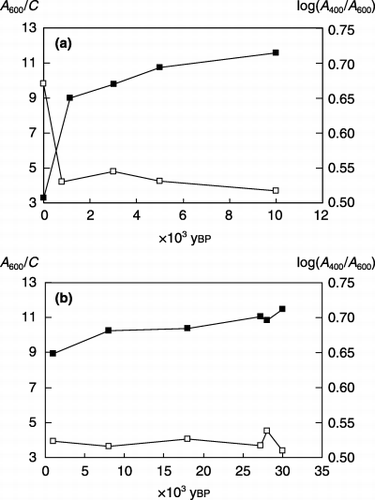
It is generally difficult to detect significant differences in the values of log(A 400/A 600) among Type A humic acids because of the small range, approximately 0.5–0.6 (CitationHiradate et al. 2004; CitationIkeya and Watanabe 2003; CitationKumada 1987). The distribution of the A 600/C value () and of the relative aromatic C content () was also narrow for the humic acids in the buried layer soils. However, regression analysis revealed a significant positive correlation between both values for all 11 humic acids (r = 0.926; P < 0.005) and for 10 samples lacking Yu-1 Type B humic acids (r = 0.929; P < 0.005), as previously observed for various soil humic acids including A, B, P and Rp types (CitationWatanabe et al. 2005). Thus, aromatic components in humic acids were more stable than aliphatic ones in soil, and their concentration probably contributed to the increase in the A 600/C value from the surface to the buried layers. The present results did not provide information about the progression of polycondensation of aromatic components.
Figure 3 13C cross polarization/magic angle spinning nuclear magnetic resonance spectra of Yubunabara humic acids.
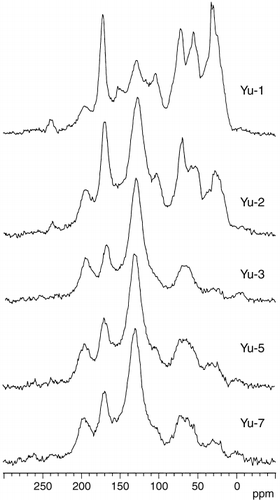
Fluctuations in the δ 13 C values, which were probably caused by the difference in the relative occupations by C 3 and C 4 plants, may have masked chronological changes in the δ 13 C values of the humus fractions. However, the differences in the δ 13 C values between each humus fraction and bulk SOM showed some characteristics of the δ13C values of the respective humus fractions. The δ13C values of WS-NHS were statistically higher (n = 11; P < 0.05) than that of bulk SOM, which may result from the large amount of carbohydrates and peptides/proteinaceous materials, because hemicellulose, cellulose and amino acids in plant cells tend to show higher δ13C values than whole plant cells (CitationBenner et al. 1987; CitationDeines 1980; CitationGleixner et al. 1993), and the carbohydrates and amino acids synthesized by microorganisms are expected to be enriched in 13C compared with the substrates (CitationMacko and Estep 1984; CitationŠantrůčkováet al. 2000). The differences in the δ13C values between WS-NHS and bulk SOM were smaller in the older layers. Possible interpretations include the microbial synthesis of WS-NHS using 13C-depleted organic matter (such as fulvic acids) as a C source while the
Figure 4 13C cross polarization/magic angle spinning nuclear magnetic resonance spectra of Ashitaka-Onoue humic acids.
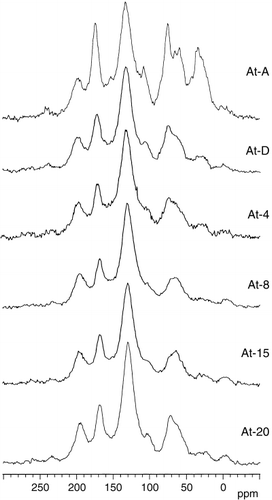
Lignin tends to show a lower δ13C value compared with whole plant cells (CitationBenner et al. 1987; CitationGleixner et al. 1993) and has been considered to be the major source of aromatic components in humic and fulvic acids. In general, the δ13C values of the humic and fulvic acids were lower (n = 11; P < 0.005) than the values of bulk SOM (maximum −3.3), which was possibly related to the contribution of lignin-derived C to humic and fulvic C. CitationLichtfouse et al. (1995) also reported higher and lower δ13C values of humin and humic acids than that of bulk SOM, respectively, and CitationHiradate et al. (2004) showed that the average δ13C values were significantly lower for humic and fulvic acids than for bulk SOM in surface volcanic ash soils. The higher δ13C values of the humic acids compared with those of the fulvic acids in the surface and younger buried humic layers might be due, in part, to the higher amino acid contents in the humic acids than in the fulvic acids (CitationWatanabe et al. 1994). The δ13C values of the humic acids after the loss of their aliphatic components in the older layers () were lower than those of the fulvic acids ().
shows the presence of a negative correlation (r = −0.921; P < 0.005) between the difference in the δ13C values of the humic acids and bulk SOM [δ13CHAs− δ13Cbulk SOM] and the proportion of aromatic C in total C of the humic acids for all samples except for the At-D layer. This observation could be explained by
Figure 5 Proportions of carbon (C) functional groups in total C of (a) Yubunebara and (b) Ashitaka-Onoue humic acids estimated from 13C cross polarization/magic angle spinning nuclear magnetic resonance. (○), alkyl C; (□), O-alkyl C; (•), aromatic C; (▴), carbonyl C.
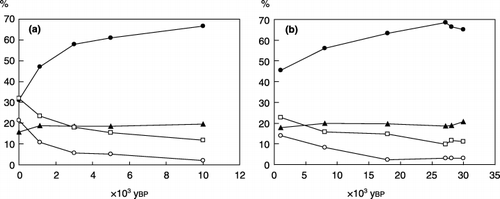
Figure 6 δ13C values of bulk soil organic matter (SOM) and humus fractions in (a) Yubunebara and (b) Ashitaka-Onoue soils. (□), bulk SOM; (•), humic acids; (▴), fulvic acids (PVP-adsorbed fraction of fulvic acid fraction); (▵), water-soluble non-humic substances (PVP-non-adsorbed fraction of fulvic acid fraction).
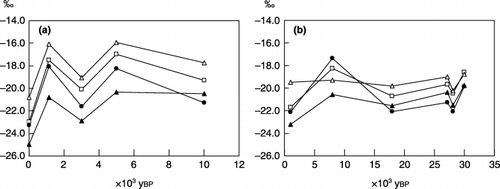
Figure 7 Relationship between the difference in the δ13C value of humic acids and bulk soil organic matter [δ13CHAs −δ13Cbulk SOM] and the proportion of aromatic carbon (C) in the total C of humic acids (n = 10).
![Figure 7 Relationship between the difference in the δ13C value of humic acids and bulk soil organic matter [δ13CHAs −δ13Cbulk SOM] and the proportion of aromatic carbon (C) in the total C of humic acids (n = 10).](/cms/asset/81f66a42-29fc-4bf8-aef5-a3f91fc4fc24/tssp_a_10382337_o_f0007g.gif)
CONCLUSIONS
The degree of humification of humic acids increased in soils within 10,000 ybp, and the corresponding increase in the relative content of aromatic C reflected the enhancement of the structural stability. The C composition of the humic acids did not vary appreciably among layers older than 10,000 ybp.
Although variation in the δ13C value of each humus fraction generally corresponded to that of bulk SOM, the difference in the δ13C value of bulk SOM varied among the fractions and among the layers.
There was a negative correlation between the relative content of aromatic C in the humic acids and [δ13CHAs −δ13Cbulk SOM].
REFERENCES
- Stevenson , FJ . 1994 . Humus Chemistry: Genesis, Composition, Reactions , 2nd edn , New York : John Wiley & Sons .
- Kumada , K . 1987 . Chemistry of Soil Organic Matter , Amsterdam : Elsevier .
- Otsuka , H , Kimiwada , K and Uehara , Y . 1994 . Genesis of humic acid derived from each plant residue of Susuki (Miscanthus sinensis), Sasa (Sasa nipponica), or Kashiwa (Quercus dentata) in fresh volcanic ash . JpnJSoil SciPlant Nutr , 65 : 629 – 636 . (in Japanese with English summary)
- Otsuka , H and Kumada , K . 1978 . Studies on a volcanic ash soil at Ohnobaru, Tarumizu City, Kagoshima Prefecture. I. Status of humus accumulated in the profile . Soil SciPlant Nutr , 24 : 265 – 276 .
- Sakai , C and Kumada , K . 1982 . Characteristics of buried humic horizons at the Shinji archaeological pits. III. Carbon, nitrogen, aluminium, silicon, iron, phenol and amino acid contents in humic and fulvic acids . Soil SciPlant Nutr , 28 : 205 – 216 .
- Barrie , A and Prosser , SJ . 1996 . “ Automated analysis of light-element stable isotopes by isotope ratio mass spectrometry ” . In Mass Spectrometry of Soils , Edited by: Boutton , TW and Yamasaki , S . 1 – 46 . New York : Marcel Dekker .
- Bender , MM . 1971 . Variations in the 13C/12C ratios of plants in relation to the pathway of photosynthetic carbon dioxide fixation . Phytochemistry , 10 : 1239 – 1244 .
- Smith , BN and Epstein , S . 1971 . Two categories of 13C/12C ratios for higher plants . Plant Physiol , 47 : 380 – 384 .
- Balesdent , J , Mariotti , A and Guillet , B . 1987 . Natural 13C abundance as a tracer for studies of soil organic matter dynamics . Soil BiolBiochem , 19 : 25 – 30 .
- Schwartz , D , Mariotti , A , Lanfranchi , R and Guillet , B . 1986 . 13C/12C ratios of soil organic matter as indicators of vegetation changes in the Congo . Geoderma , 39 : 97 – 103 .
- Agnelli , A , Trumbore , SE , Corti , G and Ugolini , FC . 2002 . The dynamics of organic matter in rock fragments in soil investigated by 14C dating and measurements of 13C . EurJSoil Sci , 53 : 147 – 159 .
- Bol , RA , Harkness , DD , Huang , Y and Howard , DM . 1999 . The influence of soil processes on carbon isotope distribution and turnover in the British uplands . EurJSoil Sci , 50 : 41 – 51 .
- Huang , Y , Bol , R , Harkness , DD , Ineson , P and Eglinton , G . 1996 . Post-glacial variations in distributions, 13C and 14C contents of aliphatic hydrocarbons and bulk organic matter in three types of British acid upland soils . OrgGeochem , 24 : 273 – 287 .
- Yoshida , M , Sakagami , K , Hamada , R and Kurobe , T . 1978 . Studies on the properties of organic matter in buried humic horizon derived from volcanic ash. I. Humus composition of buried humic horizon . Soil SciPlant Nutr , 24 : 277 – 287 .
- Tsutsuki , K and Kuwatsuka , S . 1989 . Degradation and stabilization of humus in buried volcanic ash soils. I. Humus composition, molecular size distribution of humic acids, and sugar composition of soils . Soil SciPlant Nutr , 35 : 207 – 216 .
- Ikeya , K and Watanabe , A . 2003 . Direct expression of an index for the degree of humification of humic acids using organic carbon concentration . Soil SciPlant Nutr , 49 : 47 – 53 .
- Kuwatsuka , S , Watanabe , A , Itoh , K and Arai , S . 1992 . Comparison of two methods of preparation of humic and fulvic acids, IHSS method and NAGOYA method . Soil SciPlant Nutr , 38 : 23 – 30 .
- Watanabe , A and Kuwatsuka , S . 1991 . Fractionation of soil fulvic acids using polyvinylpyrrolidone and their ionization difference spectra . Soil SciPlant Nutr , 37 : 611 – 617 .
- Watanabe , A and Kuwatsuka , S . 1992 . Chemical characteristics of soil fulvic acids fractionated using polyvinylpyrrolidone (PVP) . Soil SciPlant Nutr , 38 : 31 – 41 .
- Monteil-Rivera , F , Brouwer , EB , Masset , S , Deslandes , Y and Dumonceau , J . 2000 . Combination of X-ray photoelectron and solid-state 13C nuclear magnetic resonance spectroscopy in the structural characterization of humic acids . AnalChimActa , 424 : 243 – 255 .
- Anderson , HA , Bick , W , Hepburn , A and Stewart , M . 1989 . “ Nitrogen in humic substances ” . In Humic Substances II , Edited by: Hayes , MHB , MacCarthy , P , Malcom , RL and Swift , RS . 223 – 253 . Chichester : John Wiley & Sons .
- Cheshire , MV , Russell , JD Fraser , AR . 1992 . Nature of soil carbohydrate and its association with soil humic substances . JSoil Sci , 43 : 359 – 373 .
- Maie , N , Watanabe , A , Hayamizu , K and Kimura , M . 2002 . Comparison of chemical characteristics of Type A humic acids extracted from subsoils of paddy fields and surface ando soils . Geoderma , 106 : 1 – 19 .
- Hiradate , S , Nakadai , T , Shindo , H and Yoneyama , T . 2004 . Carbon source of humic substances in some Japanese volcanic ash soils determined by carbon stable isotopic ratio, δ13C . Geoderma , 119 : 133 – 141 .
- Watanabe , A , McPhail , DB , Maie , N , Kawasaki , S , Anderson , HA and Cheshire , MV . 2005 . Electron spin resonance characteristics of humic acids from a wide range of soil types . OrgGeochem , 36 : 981 – 990 .
- Benner , R , Fogel , ML , Sprague , EK and Hodson , RE . 1987 . Depletion of 13C in lignin and its implications for stable carbon isotope studies . Nature , 329 : 708 – 710 .
- Deines , P . 1980 . “ The isotopic composition of reduced organic carbon ” . In Handbook of Environmental Isotope Geochemistry , The Terrestrial Environment Edited by: Fritz , P and Fontes , JC . Vol. 1 , 329 – 406 . Amsterdam : Elsevier .
- Gleixner , G , Danier , H-J , Werner , RA and Schmidt , H-L . 1993 . Correlations between the 13C content of primary and secondary plant products in different cell compartments and that in decomposing Basidiomycetes . Plant Physiol , 102 : 1287 – 1290 .
- Macko , SA and Estep , MLF . 1984 . Microbial alteration of stable nitrogen and carbon isotopic compositions of organic matter . OrgGeochem , 6 : 787 – 790 .
- Šantrůčková , H , Bird , MI and Lloyd , J . 2000 . Microbial processes and carbon-isotope fractionation in tropical and temperature grassland soils . Functional Ecol , 14 : 108 – 114 .
- Lichtfouse , É , Dou , S , Houot , S and Barriuso , E . 1995 . Isotope evidence for soil organic carbon pools with distinct turnover rates–II. Humic substances . OrgGeochem , 23 : 845 – 847 .
- Watanabe , A , Itoh , K , Arai , S and Kuwatsuka , S . 1994 . Comparison of the composition of humic and fulvic acids prepared by the IHSS method and NAGOYA method . Soil SciPlant Nutr , 40 : 601 – 608 .
- Kramer , RW , Kujawinski , EB and Hatcher , PG . 2004 . Identification of black carbon derived structures in a volcanic ash soil humic acid by Fourier transform ion cyclotron resonance mass spectrometry . EnvironSciTechnol , 38 : 3387 – 3395 .
- Shindo , H , Honna , T , Yamamoto , S and Honma , H . 2004 . Contribution of charred plant fragments to soil organic carbon in Japanese volcanic ash soils containing black humic acids . OrgGeochem , 35 : 235 – 241 .
- Shindo , H , Yoshida , M , Yamamoto , A , Honma , H and Hiradate , S . 2005 . δ13C values of organic constituents and possible source of humic substances in Japanese volcanic ash soils . Soil Sci , 170 : 175 – 182 .