Abstract
We investigated the factors affecting the microbial biomass in upland soils where calcium (Ca) had accumulated because of the application of composted lime-treated sewage sludge (LSS). Soil samples (0–10 cm) were taken from field plots that had received LSS compost at the rates of 0, 20, 40 and 60 Mg ha−1 year−1 for 4 years. The pH values and the amount of exchangeable Ca2+ increased with increasing amounts of compost. Total concentrations of cadmium, copper and zinc increased with the application of LSS compost, and at a rate of 60 Mg ha−1 year−1, they were 0.7, 41 and 144 Mg kg−1 soil, respectively. Electrical conductivity (EC) increased in the soils that had received LSS compost, and this was ascribed to an increase in the amount of water-soluble Ca2+. The application of LSS compost led to a decrease in the ratio of microbial biomass C to total soil C and an increase in biomass specific respiration (qCO2), indicating adverse effects of LSS compost application on soil microbial biomass, as observed in sewage sludge-amended soils. Analysis of the ergosterol content did not reveal any increase in the proportion of fungal biomass in the total microbial biomass in contrast to earlier findings on sewage sludge-amended soils. The concentrations of heavy metals in the soils used were below the levels known to exert adverse effects on soil microbial biomass. In contrast, the ratio of microbial biomass C to total soil C decreased significantly and the qCO2 increased significantly with the increase in EC and in the amount of water-soluble Ca2+. Therefore, it was suggested that the electrolyte concentration associated with the amount of water-soluble Ca2+ was the major factor affecting microbial biomass in soils amended with LSS compost.
INTRODUCTION
Large amounts of sewage sludge have been produced in advanced countries. In Japan, more than 400 million m3 of sewage sludge is generated annually and most of it is disposed of by landfilling. However, a recent lack of landfill sites has promoted the effective utilization of sewage sludge. One of the alternative methods to dispose of sewage sludge is the application of the sludge to agricultural land after composting. The application of sewage sludge compost to agricultural land has been practiced in many countries. Nevertheless, there is a concern about the toxic effects of heavy metals accumulated by the application of sewage sludge on the soil microbial biomass (CitationBrookes and McGrath 1984; CitationChander and Brookes 1991; CitationChander et al. 1995; CitationFließbach et al. 1994). Sewage sludge contains polymers or lime used for the coagulation process. Lime-treated sewage sludge (LSS) is relatively easy to compost, compared with polymer-treated sewage sludge. In our previous study (CitationAoyama et al. 2003), we reported that the application of compost made from LSS led to the accumulation of exchangeable Ca2+ and thus increased the soil pH. An increase in soil pH generally results in an increase in microbial biomass and microbial activities in soils (CitationAdams and Adams 1983; CitationCarter 1986; CitationSoon and Arshad 2005). In contrast, the accumulation of soluble salts exerts adverse effects on soil microbial biomass (CitationKillham 1985; CitationRietz and Hynes 2003; CitationSardinha et al. 2003).
Our objective in the present study was to investigate the factors affecting microbial biomass in upland soils where calcium (Ca) had accumulated because of the application of composted LSS. We measured microbial biomass, ergosterol content and respiration in soil samples taken from field plots that had received LSS compost at the rates of 0, 20, 40 and 60 Mg ha−1 year−1 for 4 years. Heavy metal concentrations, electrical conductivity (EC) and the composition of water-soluble ions were also analyzed.
MATERIALS AND METHODS
Site and soils
Field plots were established in 1998 at the Aomori Prefectural Agriculture and Forestry Research Center, Kuroishi, Japan. The soil at this site is an alluvial soil classified as a Gray Lowland soil (Udifluvent). Details of the trial have been reported by CitationAoyama et al. (2003). The experiment was arranged in a split-plot design with LSS compost as the main factor and fertilizer treatments as secondary factors. The compost was applied at the rates of 20, 40 and 60 Mg ha−1 year−1, in addition to a control (0 Mg ha−1 year−1). Fertilizer treatments consisted of NPK treatments at three different N fertilization rates (133, 200 and 266 kg ha−1 year−1) and a control (no-fertilizer treatment). The 20 Mg ha−1 year−1 treatment lacked the “no-fertilizer plot”. The size of each plot was 3.5 m × 10 m. Crops were cultivated twice a year as follows: cabbage (Brassica oleracea L.; first crop) and turnip (Brassica rapa L.; second crop) in 1998; cabbage (first crop) and Japanese radish (Raphanus sativus L.; second crop) in 1999 and 2000; and cabbage (first and second crops) in 2001. The LSS compost and fertilizer applications were carried out in April before the planting of the first crop every year. The composts were commercially available and made from sewage sludge treated with lime for coagulation. The chemical properties of the composts used are shown in .
Soil samples were taken from the 0–10 cm layer in July (after harvest of the first crop) and September (after harvest of the second crop) 2001. Four subsamples were taken and pooled in each plot. The soil samples were passed through a 2-mm mesh sieve. The moist subsamples were used for the analysis of microbiological properties and the residual samples were air-dried for chemical analyses.
Analysis of soil chemical properties
Soil pH values were determined using a glass electrode at a ratio of 1:2.5 in water and 1 mol L−1 KCl. The total C and N contents were simultaneously determined using a Sumigraph NC-90A automatic analyzer (Sumitomo Chemical Company, Osaka, Japan). Exchangeable cations were extracted with 1 mol L−1 CH3COONH4 (pH 7) using a soil-to-solution ratio of 1:10, and the amounts of Ca2+, Mg2+, K+ and Na+ in the extract were determined by atomic absorption spectrometry. The total concentrations of Cd, Cu and Zn in the soil samples taken in September were determined by atomic absorption spectrometry, following digestion with an HClO4–HNO3 mixture. The EC value was measured in a 1:5 water extract. In the same water extract, the amounts of water-soluble cations (Ca2+, Mg2+, K+ and Na+) were determined by atomic absorption spectrometry and the amounts of water-soluble anions (Cl−, NO− 3 and SO2− 4) by indirect photometric ion chromatography (CitationAoyama 1992).
Analysis of soil microbial properties
For the analyses of microbial biomass C (Cmic) and ergosterol content, moist soil samples equivalent to 60 g oven-dry soil were weighed in 100 mL plastic beakers and adjusted to 60% of the maximum water-holding capacity (MWHC) with distilled water and then pre-incubated for 7 days at 25°C. The Cmic was measured using the fumigation–extraction procedure proposed by CitationVance et al. (1987). In brief, a portion of the pre-incubated soil sample corresponding to 25 g oven-dry soil was fumigated with alcohol-free chloroform for 24 h. Organic C was extracted from the fumigated and unfumigated soil samples with 100 mL of 0.5 mol L−1 K2SO4 and quantified using a TOC analyzer (TOC-VE; Shimadzu Company, Kyoto, Japan). A k EC factor of 0.45 was used
Table 1 Chemical properties of the sewage sludge compost
Table 2 Chemical properties of soils
Table 3 Heavy metal concentrations of soils sampled in September
For the measurement of soil respiration, moist soil samples equivalent to 30 g oven-dry soil were weighed in 50 mL glass vials and then adjusted to 60% of the MWHC with distilled water. After pre-incubation for 7 days at 25°C, each vial was placed in a 900 mL airtight glass jar containing 5 mL of water to maintain a moist atmosphere and a plastic vial with 5 mL of 1 mol L−1 NaOH to trap CO2, and incubated for 7 days at 25°C (CitationAoyama and Nagumo 1997). The amount of CO2 in NaOH was determined by titration with 0.1 mol L−1 HCl using an automatic titrator.
Statistics
Data for the soil chemical and microbial properties were analyzed using two-way anovas (CitationYanai 1998). For all data, the effects of the fertilizer treatments were not statistically significant at P = 0.05. Thus, means for each compost application rate were separated by the least significant difference (LSD) test at P = 0.05 (CitationYanai 1998).
RESULTS AND DISCUSSION
Effects on soil chemical properties
The pH values in H2O and KCl were considerably lower in the soil without LSS compost application (). Both soil pH values increased significantly with increasing amounts of LSS compost. Both soil pH values exceeded 7 in the 60 Mg ha−1 year−1 treatment. The amount of exchangeable Ca2+ also increased with the application of LSS compost, reflecting the high concentration of Ca in the compost (). Thus, the increase in soil pH could be attributed to the accumulation of exchangeable Ca2+ derived from the LSS compost.
The application of LSS compost increased significantly the total C and N contents, demonstrating the accumulation of organic matter derived from LSS compost. However, the C/N ratio was not significantly affected by LSS compost application.
The concentrations of Cd, Cu and Zn in the soils sampled in September increased significantly with increasing amounts of LSS compost (). Nevertheless, the concentrations were relatively low, even in the 60 Mg ha−1 year−1 treatment.
Overall, the EC value was higher in September than in July (). The application of LSS compost
Table 4 Electrical conductivity (EC) and water-soluble ion contents in soils
Effects on soil microbial properties
In July, compared with the unamended treatment, LSS compost application did not significantly affect the amount of Cmic in the 20 and 40 Mg ha−1 year−1 treatments, but decreased it significantly in the 60 Mg ha−1 year−1 treatment (). In September Cmic significantly increased in the 20 Mg ha−1 year−1 treatment and significantly decreased in the 40 and 60 Mg ha−1 year−1 treatments. CitationChander and Brookes (1991) and CitationFließbach et al. (1994) considered that the ratio of Cmic to soil organic C was a useful indicator for evaluating the effects of heavy metals on the microbial biomass in soils amended with sewage sludge. When the ratio of microbial C to total soil C (Cmic/T − C) was calculated, it decreased significantly with an increase in the amount of LSS compost in
Figure 2 Amounts of microbial biomass C (Cmic) expressed on (a) an oven-dry soil basis and (b) on a total soil C basis in the soils sampled in July (□) and September (). Error bars represent standard error. Bars with the same letter do not differ significantly according to Fisher's protected least significant difference (P = 0.05).
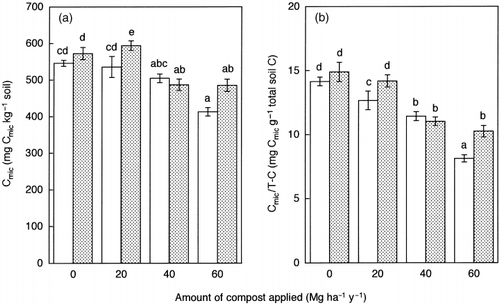
Ergosterol is peculiar to fungal cells and is closely related to the fungal biomass in soil (CitationRuzicka et al. 2000; CitationStahl and Parkin 1996). In July there were no significant differences in the ergosterol content among the treatments (). In contrast, the ergosterol content was significantly reduced by the application of LSS compost in September. When the ratio of ergosterol to Cmic (ergosterol/Cmic) was calculated, the results showed the same trend as that for ergosterol (). Thus, significant seasonal fluctuations in the proportion of fungal biomass in the total microbial biomass were revealed for the LSS compost treatments.
The application of LSS compost not only increased soil respiration but also biomass specific respiration (qCO2), regardless of sampling time (). CitationKillham (1985) observed that the ratio of respired C to biomass-incorporated C increased with increasing magnitude of environmental stresses, such as heavy metal pollution, acid rain and salinity, when 14C-labeled glucose was added to the soils. He ascribed the increase in the ratio caused by the environmental stresses to the increase in the diversion of carbon from biosynthesis to maintenance energy requirements. This mechanism has been adopted to explain the high qCO2 in heavy metal-polluted soils (CitationBrookes 1995). Therefore, in addition to the decrease in the Cmic/T − C ratio, the increase in qCO2 indicates the adverse effects of LSS compost on soil microbial biomass.
Factors affecting the microbial biomass
In the soils amended with sewage sludge, the ratio of Cmic to soil organic C decreased, whereas qCO2 increased (CitationChander and Brookes 1991; CitationFließbach et al. 1994; CitationValsecchi et al. 1995). These phenomena induced by sewage sludge application have been ascribed to the accumulation of heavy metals in the soil (CitationGiller et al. 1998). In the present study, the application of LSS compost also resulted in a decrease in the ratio of Cmic/T − C and in an increase in the qCO2.
The concentrations of Cd, Cu and Zn in the soil samples with 60 Mg ha−1 year−1 of LSS compost were 0.7, 41 and 144 mg kg−1 soil, respectively. In sewage-sludge-amended soils polluted with similar levels of Cd, Cu and Zn, a decrease in the ratio of Cmic to soil organic C and an increase in the qCO2 were not observed (CitationFließbach et al. 1994). CitationChander et al. (1995), who analyzed the Cmic in soils incubated with heavy metal-enriched sewage sludge, reported that individual heavy metals did not exert harmful effects on the Cmic at a concentration of 3 mg Zn kg−1 soil, 150 mg Cu kg−1 soil or 300 mg Zn kg−1 soil. CitationRost et al. (2001) showed that Cmic did not decrease at 467 mg Zn kg−1 soil, and further that the
Figure 3 Amounts of ergosterol expressed on (a) an oven-dry soil basis and (b) on a total soil C basis in the soils sampled in July (□) and September (). Error bars represent standard error. Bars with the same letter do not differ significantly according to Fisher's protected least significant difference (P = 0.05).
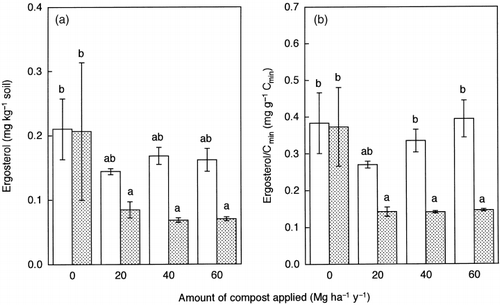
Figure 4 (a) Soil respiration and (b) biomass specific respiration (qCO2) in the soils sampled in July (□) and September (). Error bars represent standard error. Bars with the same letter do not differ significantly according to Fisher's protected least significant difference (P = 0.05).
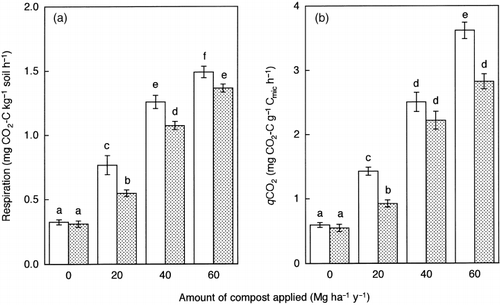
Figure 5 Relationships between (a) electrical conductivity (EC) and the ratio of microbial C to total soil C (Cmic/T-C), (b) between EC and biomass specific respiration (qCO2), (c) between the amount of water-soluble Ca2+ and Cmic/T-C ratio and (d) between the amount of water-soluble Ca2+ and qCO2. ***P < 0.001.
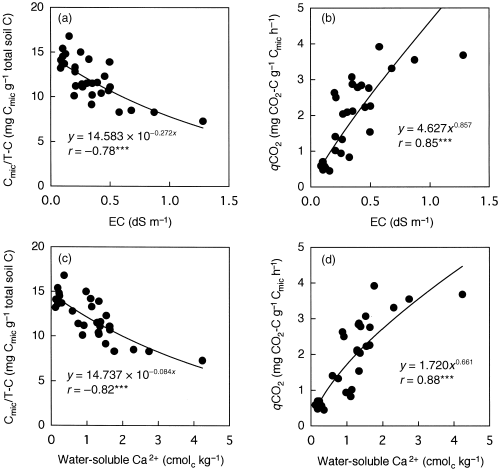
The application of LSS compost significantly increased the soil pH values. However, an increase in soil pH generally exerts favorable effects on soil microbial biomass and microbial activities (CitationAdams and Adams 1983; CitationCarter 1986; CitationSoon and Arshad 2005). In contrast, soil microbial biomass and its activities are influenced by soil salinity (CitationKillham 1985). In the present study, the application of LSS compost led to an increase in the EC and in the amounts of water-soluble ions as a consequence of the accumulation of Ca in soils. CitationRietz and Haynes (2003) reported that with an increase in EC, the ratio of Cmic to soil organic C decreased exponentially and the qCO2 increased exponentially in saline soils. They considered that osmotic stress was the main cause of the adverse effects on the microbial biomass. A significant negative correlation between EC and the ratio of Cmic to soil organic C and a significant positive correlation between EC and the qCO2 were also observed for acidic saline soils (CitationSardinha et al. 2003). These findings suggested that the increased electrolyte concentration induced by the application of LSS compost was the main cause of the adverse effects on soil microbial biomass. To verify this assumption, the ratio of Cmic/T − C and the qCO2 were plotted against EC (). The ratio of Cmic/T − C decreased significantly and the qCO2 increased significantly as EC increased. The EC was strongly correlated with the total amount of water-soluble cations (), and Ca2+ was the predominant water-soluble cation (). The amount of water-soluble Ca2+ also showed significant correlations with the ratio of Cmic/T − C and the qCO2 (). The correlation coefficients (r) were higher for the amount of water-soluble Ca2+ than for EC. These results indicate that the electrolyte concentration associated with the amount of water-soluble Ca2+ was the major factor affecting the microbial biomass in the soils amended with LSS compost. Osmotic stress was most probably the cause of the adverse effects on microbial biomass in the LSS compost-amended soils in the same way as for the saline soils. An imbalance of mineral nutrients may also inhibit microbial growth in the LSS compost-amended soils.
The ratio of ergosterol/Cmic is considered to represent the proportion of fungal biomass in the total microbial biomass in soil because the amount of ergosterol is closely related to fungal biomass. CitationKhan and Scullion (2000) have found that in soil samples incubated with heavy metal-enriched sewage sludge, the input of heavy metals increased the amount of ergosterol and decreased the ratio of bacterial phospholipid fatty acids (PLFA) to fungal PLFA. These findings suggested that the heavy metals derived from sewage sludge increased the proportion of fungal biomass in the total microbial biomass. In contrast, although significant seasonal fluctuations were observed, an increase in the ratio of ergosterol/Cmic was not confirmed in the present study. CitationSardinha et al. (2003) reported a negative correlation between EC and the ratio of ergosterol/Cmic for acidic saline soils. A decrease in the proportion of fungal biomass in the total microbial biomass was also found in alkaline saline soils using PLFA analysis (CitationPankhurst et al. 2001). Additional information that the fungal/bacterial ratio measured using the selective inhibition technique decreased significantly with increasing pH was provided by CitationBååth and Anderson (2003). Thus, the increased electrolyte concentration along with the high soil pH may inhibit fungal growth in soils that received LSS compost.
CONCLUSIONS
The application of LSS compost resulted not only in a decrease in the ratio of Cmic/T − C but also in an increase in the qCO2. These results revealed the adverse effects of LSS compost on soil microbial biomass. The analysis of heavy metals indicated that the total concentrations of Cd, Cu and Zn in the soils used in the present study were below the levels known to exert adverse effects on soil microbial biomass. In contrast, EC increased in the LSS compost treatments, and this increase was ascribed to an increase in the amount of water-soluble Ca2+. With the increase in EC and in the amount of water-soluble Ca2+, the ratio of Cmic/T − C decreased and qCO2 increased. It was, therefore, suggested that the electrolyte concentration associated with the amount of water-soluble Ca2+ was the major factor affecting microbial biomass in soils amended with LSS compost.
ACKNOWLEDGMENTS
We thank Ms J. Tazawa and Ms C. Tarusawa for their technical assistance. B. Zhou thanks the Aomori Prefectural Government for granting him a visiting fellowship during the course of this study.
REFERENCES
- Brookes , PC and McGrath , SP . 1984 . Effects of metal toxicity on the size of the soil microbial biomass . JSoil Sci , 35 : 341 – 346 .
- Chander , K and Brookes , PC . 1991 . Effects of heavy metals from past applications of sewage sludge on microbial biomass and organic matter accumulation in a sandy loam and silty loam UK soil . Soil BiolBiochem , 23 : 927 – 932 .
- Chander , K , Brookes , PC and Harding , SA . 1995 . Microbial biomass dynamics following addition of metal-enriched sewage sludges to a sandy loam . Soil BiolBiochem , 27 : 1409 – 1421 .
- Fließbach , A , Martens , R and Reber , HH . 1994 . Soil microbial biomass and activity in soils treated with heavy metal contaminated sewage sludge . Soil BiolBiochem , 26 : 1201 – 1205 .
- Aoyama , M , Zhou , B , Saitoh , M and Yamaguchi , N . 2003 . Effects of lime-treated sewage sludge compost application on soil chemical properties and soil organic matter . JpnJSoil SciPlant Nutr , 74 : 749 – 757 . (in Japanese with English summary)
- Adams , TMcM and Adams , SN . 1983 . The effects of liming and soil pH on carbon and nitrogen contained in the soil biomass . JAgricSci , 101 : 553 – 558 .
- Carter , MR . 1986 . Microbial biomass and mineralizable N in solonetzic soils: influence of gypsum and lime amendments . Soil BiolBiochem , 18 : 531 – 537 .
- Soon , YK and Arshad , MA . 2005 . Tillage and liming effects on crop and labile soil nitrogen in an acid soil . Soil Tillage Res , 80 : 23 – 33 .
- Killham , K . 1985 . A physiological determination of the impact of environmental stress on the activity of microbial biomass . EnvironPollut , 38 : 283 – 294 .
- Rietz , DN and Haynes , RJ . 2003 . Effects of irrigation-induced salinity and sodicity on soil microbial activity . Soil BiolBiochem , 35 : 845 – 854 .
- Sardinha , M , Müller , T , Schmeisky , H and Joergensen , RG . 2003 . Microbial performance in soils along a salinity gradient under acidic conditions . Soil BiolBiochem , 23 : 237 – 244 .
- Aoyama , M . 1992 . Analysis of inorganic anions in soil solutions by indirect photometric ion chromatography . JpnJSoil SciPlant Nutr , 63 : 597 – 601 . (in Japanese)
- Vance , ED , Brookes , PC and Jenkinson , DS . 1987 . An extraction method for measuring soil microbial biomass C . Soil BiolBiochem , 19 : 703 – 707 .
- Wu , J , Joergensen , RG , Pommerening , B , Chaussod , R and Brookes , PC . 1990 . Measurement of soil microbial biomass C by fumigation extraction: an automated procedure . Soil BiolBiochem , 22 : 1167 – 1169 .
- Gong , P , Guan , X and Witter , E . 2001 . A rapid method to extract ergosterol from soil by physical disruption . ApplSoil Ecol , 17 : 285 – 289 .
- Aoyama , M and Nagumo , T . 1997 . Effects of heavy metal accumulation in apple orchard soils on microbial biomass and microbial activities . Soil SciPlant Nutr , 43 : 601 – 612 .
- Yanai , H . 1998 . 4 Steps Excel Statistics , 151 – 162 . Tokorozawa : OMS . (in Japanese)
- Ruzicka , S , Edgerton , D , Norman , M and Hill , T . 2000 . The utility of ergosterol as a bioindicator of fungi in temperate soils . Soil BiolBiochem , 32 : 989 – 1005 .
- Stahl , PD and Parkin , TB . 1996 . Relationship of soil ergosterol concentration and fungal biomass . Soil BiolBiochem , 28 : 847 – 855 .
- Brookes , PC . 1995 . The use of microbial parameters in monitoring soil pollution by heavy metals . BiolFertilSoils , 19 : 269 – 279 .
- Valsecchi , G , Gigliotti , C and Farini , A . 1995 . Microbial biomass, activity, and organic matter accumulation in soils contaminated with heavy metals . BiolFertilSoils , 20 : 253 – 259 .
- Giller , KE , Witter , E and McGrath , SP . 1998 . Toxicity of heavy metals to microorganisms and microbial processes in agricultural soils: a review . Soil BiolBiochem , 30 : 1389 – 1414 .
- Rost , U , Joergensen , RG and Chander , K . 2001 . Effects of Zn enriched sewage sludge on microbial activities and biomass in soil . Soil BiolBiochem , 33 : 633 – 638 .
- Khan , M and Scullion , J . 2000 . Effects of soil on microbial responses to metal contamination . EnvironPollut , 110 : 115 – 125 .
- Pankhurst , CE , Yu , S , Hawke , BG and Harch , BD . 2001 . Capacity of fatty acid profiles and substrate utilization patterns to describe differences in soil microbial communities associated with increased salinity or alkalinity at three locations in South Australia . BiolFertilSoils , 33 : 204 – 217 .
- Bååth , E and Anderson , T-H . 2003 . Comparison of soil fungal/bacterial ratios in a pH gradient using physiological and PLFA-based techniques . Soil BiolBiochem , 35 : 955 – 963 .