Abstract
To examine the possibility of breeding higher-yielding cultivars with a high nitrogen use efficiency (NUE) and to provide simple criteria for the selection and breeding of high-yielding cultivars with a high NUE and useful information for the mapping of quantitative trait loci (QTL) controlling NUE, recombinant inbred lines (RILs) of rice were subjected to hydroponic culture in 2000 and 2001. Grain yield and yield components, total nitrogen absorption (NTA), pre-heading and post-heading nitrogen absorption (pre-NA and post-NA, respectively), and nitrogen use efficiency for grain yield (NUEg) were analyzed. Transgressive segregations for grain yield and yield components, NTA and NUEg were observed. RILs with a high grain yield tended to show a larger number of panicles m−2 and of spikelets per panicle, and a higher filled grain percentage than RILs with a low grain yield. Increasing NTA and NUEg resulted in increases in the number of spikelets m−2 and grain yield, while increasing NTA resulted in a decrease in NUEg. The contribution ratio of the number of panicles m−2 to grain yield was higher than that of the other yield components in both 2000 (31.8%) and 2001 (32.2%). In both years, the relative contribution ratios of NUEg and NTA to grain yield were approximately 56% and 43%, respectively. At the same high-yielding level, there was a significant difference in the NTA and NUEg values. These results suggest the possibility of breeding high-yielding cultivars with higher NUEg.
INTRODUCTION
Nitrogen is one of the most important nutrients for growth regulation and grain production of rice in paddy fields (CitationMastsushima 1976; CitationMurayama 1967, Citation1979; CitationTanaka et al. 1964; CitationWada 1969). It is generally recognized that all high-yielding rice varieties respond well to applied N, requiring large amounts of N fertilizer (CitationTanaka et al. 1964; CitationYoshida 1981). However, heavy application of chemical fertilizers not only increases the cost of rice production, but also causes agro-environmental pollution, including eutrophication of rivers and lakes and nitrate pollution of surface and underground water, and contributes to the greenhouse effect through the release of NOx gases from paddy soils (CitationDuxbury et al. 1993; CitationGalbally et al. 1987; CitationMurayama 1979). Therefore, many studies have been conducted to improve N use efficiency through agronomic management, for example, by controlling the timing, rate, placement and source of fertilizers (CitationCassman et al. 1994; CitationDatta and Buresh 1989; CitationFillery and Vlek 1986; CitationSchnier et al. 1990). However, the acceptance and adoption of N management strategies, which have often been associated with high labor requirements, had been discouraging (CitationSingh et al. 1998). Recently, field experiments have revealed the existence of genetic variability for N use efficiency in rice (CitationBroadbent et al. 1987; CitationInthapanya et al. 2000; CitationSingh et al. 1998; CitationTirol-padre et al. 1996), and the possibility of improving N use efficiency in rice through genotype selection (CitationKoutroubas and Ntanos 2003).
The genetic basis of nitrogen use efficiency has not been fully elucidated because of its complexity and quantitative nature. For quantitative trait loci (QTL) analysis of such a trait, DNA markers and genome mapping techniques have become powerful tools (CitationTanksley 1993). To date, QTL technology has been suitable for studies on important agricultural traits in rice, such as a yield and yield-related traits, plant height and heading (CitationCao et al. 2001). Another important aspect is the detailed evaluation of the traits of each genotype. To minimize the effect of heterozygosity, the use of doubled haploid lines or recombinant inbred lines (RILs) is preferable because the traits can be measured in replicated experiments and the effect of environmental factors can also be minimized.
We analyzed the characteristics of nitrogen absorption and nitrogen use efficiency in relation to grain yield among RILs derived from a cross between Zhenshan 97 and Minghui 63, parents of Shanyou 63, a famous F1 hybrid rice from China. In the current study, the RILs were cultured using the same nutrient solution and under the same environmental conditions because it is difficult to assess genotypic differences in a paddy field. This information should also be useful for the selection and breeding of high yielding rice cultivars with high N use efficiency and for the mapping of QTL controlling NUEg.
MATERIALS AND METHODS
Rice materials
A total of 118 (F12) and 191 (F13) RILs were subjected to hydroponic culture in 2000 and 2001, respectively. They were developed using the single-seed descent method from a cross between the cultivar Zhenshan 97 (ZX 97, an inbred indica and the female parent of Shanyou 63 [SY 63], the traditional hybrid rice grown in China) and the cultivar Minghui 63 (MH 63, an inbred indica and the male parent of Shanyou 63) to the F12/F13 generations. MH 63 is one of the most important restorer lines in hybrid rice production in China, expressing abundant agronomically desirable traits such as superior grain quality and resistance to various types of bacterial blight caused by Xanthomonas oryzae pv. oryzae and blast caused by the heterothallic ascomycete fungus Magnaporthe grisea (CitationPeng and Zhang 1999). ZX 97 is an elite maintainer line of hybrid rice extensively used in rice production in China (CitationLiu et al. 2003).
Experimental design and growth conditions
Hydroponic culture was carried out outside at Yangzhou University, China, in the rice-growing seasons of 2000 and 2001. Eight concrete ponds (880 cm in length × 135 cm in width × 50 cm in depth) were connected to each other using iron tubes (10 cm in diameter) running along the bottom. The ponds were covered with concrete planks (135 cm in length, 16.7 cm in width), each containing 14 holes (4 cm in diameter) for seedling fixation. One 2-leaf-old seedling per hole (62 hills m−2), grown for 20 days in a soil seedbed, was fixed into the holes with a sponge on 2 June in each year. Twenty-eight seedlings were prepared for each RIL material. The nutrient solution was formulated according to the method described by CitationYoshida et al. (1976). Ten mg L−1 nitrogen (Urea-N) with 250 mg L−1 Mg2+, 34 mg L−1 K+, 50 mg L−1 Fe3+, 2.86 mg L−1 BO3− 4, 0.22 mg L−1 Zn2+, 2.21 mg L−1 Mn2+, 0.08 mg L−1 Cu2+, 0.02 mg L−1 MoO2− 4 and 41.86 mg L−1 Ca2+ were added to the ponds at the time of transplanting and replaced at 10-day intervals. During the rice-growing season, the weather was unstable across the 2-year period. Mean temperature was higher from 1–10 July and 21–31 July in 2001 compared with 2000, and vice versa from 11–20 July and 1–20 August. Sunshine hours were longer from 21–30 June, 1–10 September and 21–30 September in 2001 compared with 2000, and vice versa from 11–20 June, 11–20 July, 11–20 September and 1–10 August.
Sampling and measurements
Two plants from each RIL were sampled at heading and maturity, respectively, separated into leaf blades, leaf sheaths plus stems, roots and panicles, and milled to determine N content using the Kjeldahl distillation method. At maturity, 10 plants were sampled to determine the number of panicles m−2, the number of spikelets per panicle, filled grain percentage and 1000-grain weight. Filled grain was selected using tap water with a gravity of 1.00 g cm−3 and the grain (unhulled) yield was adjusted to a moisture content (MC) of 13.5%. A further five plants were sampled to determine dry weight after drying at 85°C to a constant weight.
Traits
Calculations for the traits included dry weight and nitrogen content determinations and the traits were defined as follows: (1) total nitrogen absorption (NTA g m−2) = plant nitrogen content at maturity, (2) pre-heading nitrogen absorption (pre-NA g m−2) = total plant nitrogen absorption from transplanting to heading, (3) post-heading nitrogen absorption (post-NA g m−2) = NTA minus pre-NA, (4) grain yield (g m−2) = unhulled grain weight, (5) nitrogen use efficiency for grain yield (NUEg gGW gN−1) = grain yield divided by NTA.
Statistical analyses
Hierarchical cluster analysis procedure was used in the current study to identify relatively homogeneous groups of cases (or variables) based on selected characteristics using an algorithm that started with each case (or variable) in a separate cluster and combined clusters until only one was left (SPSS, Chicago, IL, USA, 1998).
All data were subjected to one-way analysis anova to determine the differences among the clusters.
The calculation of the contribution ratio of each component was as follows: data analysis involved linearising the multiplicative relationships using logs and then determining the contribution of each component trait to the sum of squares of the resultant trait. The sum of the cross products of each component trait by the resultant trait (Σxiyi) divided by the resultant trait (Σy2 i) gave the relative contribution of each component variable to the resultant variable (CitationMoll et al. 1982).
RESULTS
Growth duration
Heading of the RILs occurred from 26 July to 2 September (56–92 days after transplanting [DAT]) in 2000 and from 29 July to 6 September (59–96 DAT) in 2001. Maturity occurred from 25 August to 25 September (80–115 DAT) in 2000 and from 24 August to 27 September (83–117 DAT) in 2001.
Distribution of the mean values of grain yield and yield components, NTA and NUEg
The distribution of the mean values of grain yield and yield components, NTA and NUEg in the RILs is shown in . Significant genetic variations in these measured traits were detected among the parents in 2000 and among RILs in 2000 and 2001, respectively, except for the 1000-grain weight in the RILs. Grain yield and yield components, NTA and NUEg values of MH 63 were significantly higher than those of ZX 97. The values of the grain yield, number of spikelets per panicle and NUEg of the F1 hybrid (SY 63) were significantly higher than those of both parents. Transgressive segregation of yield and yield components, NTA and NUEg in the RILs in 2000 was also observed, and the mean values of these traits were closer to those of MH 63 than ZX 97. Moreover, a number of the RILs in 2000 showed higher values than SY 63.
Grain yield and yield components
Grain yield and yield components are listed in . According to the hierarchical cluster analysis procedure, the grain yield of the RILs was classified into 6 clusters (A–F) from high to low, respectively. Significant differences (P = 0.05) in grain yield were observed between adjacent clusters from A to F. The coefficient of variation (CV) of the lowest grain yield was comparatively higher than that of the medium and higher grain yields. The range of each yield component in the RILs was approximately the same between years, whereas the mean number of spikelets per panicle and filled grain percentage were higher in 2000 than in 2001. The mean values of all the yield components tended to increase with increasing grain yield in both years.
In both years, grain yield was significantly correlated with the number of panicles m−2, the number of spikelets per panicle, and the filled grain percentage. In both years, grain yield was also closely correlated with the number of spikelets m−2, which is a product of the number of panicles m−2 and the number of spikelets per panicle, the latter mainly determining the number of spikelets m−2 ().
The results of multiple regressions between the resultant trait (grain yield Y1) and the component traits (number of panicles m−2, X1; number of spikelets per panicle, X2; filled grain percentage, X3) are shown in . Eighty percent of the variation in grain yield in both years was explained by these three traits, the contribution ratio of each trait was 31.8%, 26.0% and 24.4% in 2000 and 32.2%, 25.5% and 24.1% in 2001, respectively.
Relationship between grain yield and growth duration
The duration of growth from transplanting to heading showed a significant positive correlation with grain yield in both years, although the correlation coefficients were comparatively low (r 00 = 0.271, P = 0.01; r 01 = 0.222, P = 0.05, respectively). In contrast, the correlation between the growth duration from transplanting to maturity and grain yield was not significant in either year (r 00 = 0.010, r 01 = −0.032, respectively).
Nitrogen absorption and NUEg
The NTA and NUEg values among the RILs for each cluster of grain yield are listed in . NTA was significantly different among all the RILs (P = 0.01) in both years. The mean value of NTA was 16.9 g m−2 with a CV of 24.0% and 15.3 g m−2 with a CV of 22.1% in 2000 and 2001, respectively. A wide variation range in NTA values was observed among RILs in the same cluster, except for cluster A, in 2000. In general, the higher the mean grain yield, the higher the mean value of NTA.
NTA could be divided into two components, pre-NA and post-NA. Pre-NA ranged from 7.5 to 24.6 g m−2 (CV,
Table 1 Grain yield and yield components, NTA and NUEg of Shanyou 63 (SY 63) and parents (ZX 97 and MH 63) in 2000 and of the RILs in 2000 and 2001
Table 2 Grain yield and yield components, NTA and NUEg in each cluster of the RILs grown in 2000 and 2001
There was considerable variation in the NUEg values among the clusters, with a mean value of 37.9 gGW gN−1 in 2000 and 35.6 gGW gN−1 in 2001. The CV was 19.0% in 2000 and 26.5% in 2001 (). The CVs of NUEg in each cluster tended to be higher with decreasing grain yield in both years. The higher the mean grain yield, the higher the mean NUEg value.
Relationships among grain yield and yield components, nitrogen absorption at different rice growth stages and NUEg
Relationships among grain yield and yield components, nitrogen absorption at different growth stages and NUEg are presented in . The NTA values were strongly correlated with the number of panicles m−2, number of spikelets m−2, pre-NA and post-NA and grain yield in both years. NUEg showed a significant positive correlation with the number of spikelets per panicle, number of spikelets m−2, filled grain percentage and grain yield as well as a significant negative correlation with NTA in both years.
Results obtained from multiple regression analysis indicated that 97.8% and 94.3% of the variation in grain yield in 2000 and 2001 was explained by NUEg and NTA, respectively. The contribution ratios of these traits were 56.3% and 43.7%, respectively, in 2000, and 56.6% and 43.4%, respectively, in 2001 ().
shows the relationships between the amount of NTA, NUEg in 2000 and 2001, respectively. NUEg showed a significant negative correlation with NTA (r 00 = −0.278, P = 0.01; r 01 = −0.361, P = 0.01, respectively). This correlation was strong at the same yielding level. In the current study, for example, along the high iso-grain yield line at 800 g m−2 among the RILs belonging to cluster B in 2000 and cluster A in 2001, the highest nitrogen use efficiency line showed a minimum NTA (14.9 g m−2) with a maximum NUEg (51.3 gGW gN−1) in 2000; these values were 13.2 g m−2 and 61.6 gGW gN−1, respectively, in 2001. In contrast, the lowest nitrogen use efficiency line showed a NUEg of 32.8 gGW gN−1 with an NTA of 28.1 g m−2; these values were 37.0 gGW gN−1 and 23.4 g m−2, respectively, in 2001.
Table 3 Correlation coefficients between grain yield and yield components or NTA and NUEg of RILs grown in 2000 and 2001
Table 4 Results of multiple regression analysis and contribution ratios
Figure 1 Relationship between total nitrogen absorption (NTA) and nitrogen use efficiency for grain yield (NUEg) among recombinant inbred lines (RILs) in (A) 2000 and (B) 2001. The curve lines in the figure indicate the iso-grain yield. Grain yield = NTA × NUEg. Numerals on the right side in both (A) and (Bb) indicate the grain yield (g m−2), **P = 0.01. Clusters A–F in the figure correspond to those in Table 2.
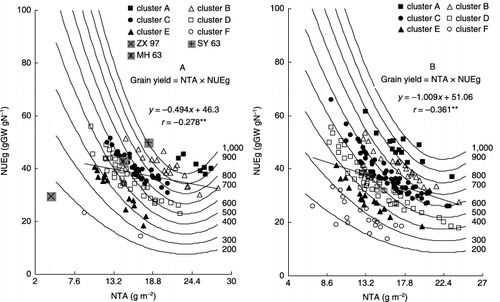
Relationships among growth duration, nitrogen absorption at different rice growth stages and NUEg
Growth duration from transplanting to heading showed a significant positive correlation with pre-NA (r 00 = 0.635, P = 0.01; r 01 = 0.363, P = 0.01, respectively) and NTA (r 00 = 0.447, P = 0.01, r 01 = 0.409, P = 0.01, respectively) in both years. Similar results were recorded between the growth duration from transplanting to maturity and pre-NA (r 00 = 0.606, P = 0.01; r 01 = 0.419, P = 0.01, respectively) and NTA (r 00 = 0.516, P = 0.01; r 01 = 0.402, P = 0.01, respectively). Growth duration from transplanting to heading showed a significant negative correlation with NUEg in both years (r 00 = −0.257, P = 0.01, r 01 = −0.404, P = 0.01, respectively), and similar results were obtained between the growth duration from transplanting to maturity and NUEg (r 00 = −0.348, P = 0.01; r 01 = −0.375, P = 0.01, respectively).
DISCUSSION
Experimental conditions and materials used
As it is not possible to avoid interference from soil fertility when using soil culture, the current experiment was carried out under hydroponic culture. Ponds were flooded with a nutrient solution continuously from transplanting to maturity to guarantee that all rice plants were grown under the same nutrition conditions, ensuring that any differences in nitrogen absorption and utilization for grain yield were caused by genotype differences only. In the current study, all the rice lines were cultured at a density of 62 plants (hills) m−2 because of limited space; this was higher than the Chinese conventional density of approximately 44 plants (hill) m−2. Grain yield and yield components and related functional components were determined at this density.
F1 (SY 63) plants crossed between ZX 97 and MH 63 showed larger values for grain yield, NTA and NUEg because of hybrid vigor. The results were in agreement with those from previous reports (CitationIchii and Nakamura 1990; CitationYao et al. 2000b). To produce RILs, the F1 (SY 63) plants were selfed, and then the generations were advanced using the single-seed descent method to the F12/F13 generations. With advancement of the generations up to F12 or F13, the genotypes of these rice lines became comparatively stable. Therefore, the abundant agronomically desirable traits of these RIL lines enabled the selection of superior genotypes according to the needs of rice production.
Grain yield and yield components of the RILs
The mean grain yield was lower in 2001 than in 2000, possibly because of differences in the weather conditions. The shorter sunshine hours before, during and/or after the heading period of most RILs in 2001 resulted in a lower number of spikelets per panicle and/or a lower filled grain percentage compared to those in 2000 () (CitationMastsushima 1976). Large genotypic variations in grain yield among the RILs were revealed under hydroponic culture. RILs with a higher grain yield tended to display a larger number of panicles m−2 and of spikelets per panicle, and a higher filled grain percentage. The number of spikelets m−2 exhibited the highest correlation coefficient with grain yield (). This was supported by the fact that further increases in rice yield depended on the presence of a higher number of spikelets per unit area (CitationMastsushima 1976; CitationYamamoto et al. 1991; CitationYao et al. 2000c). Compared to the other yield components, the number of panicles m−2 contributed most to grain yield in both years (). A similar finding was reported by CitationYoshida et al. (1972) for transplanted rice and by CitationMiller et al. (1991) for water-seeded rice.
Differences in NTA and NUEg among RILs
Genotypic variations in NTA and NUEg were significant among the RILs under hydroponic culture. Many authors have found significant genotypic variations in NTA and NUEg (CitationBroadbent et al. 1987; CitationDatta De and Broadbent 1990; CitationHasegawa 2003; CitationHiguchi and Yoshino 1986; CitationInthapanya et al. 2000; CitationJiang and Dai 2003; CitationMotomatsu et al. 1988; CitationSingh et al. 1998; CitationTirol-Padre et al. 1996; CitationYao et al. 2000 a,Citationb,Citationc; CitationYoshida 1981). However, the wide genotypic differences in NTA (CV was 24.0% and 22.1% in 2000 and 2001, respectively) and NUEg (CV was 19% and 26.5% in 2000 and 2001, respectively) observed in the current study had not been recorded in these previous reports. Therefore, the current results implied that selection of cultivars or lines with high NTA and NUEg values could be achieved, although differences in the NTA and NUEg values among the RILs should be clarified under different levels of nitrogen application in further studies (CitationSeino 1975).
NTA and NUEg in relation to grain yield and yield components
The functional components of grain yield were found to be NTA and NUEg. Genetic variations in grain yield among the RILs at the same N level were attributed to variability in NTA at different rice growth stages and NUEg. Increasing total pre-NA and post-NA or increased NUEg resulted in an increase in grain yield. The contribution of NUEg to the total variation in grain yield was highest among all the functional components, accounting for 56% of the variation in both years (). CitationTirol-Padre et al. (1996), using 180 lines, reported that NUEg may be more consistent across environments than the total nutrient content and may be a more useful character for developing new cultivars adapted to low soil fertility conditions. The current results suggested that more attention should be paid to NUEg for improving grain yield, because improving NUEg could reduce not only environment pollution but also agriculture costs. In the current study, some high-yielding lines showed a high NUEg value and comparatively low nitrogen absorption (). Along the iso-grain yield line at 800 g m−2, the highest nitrogen use efficiency showed a minimum NTA (14.9 g m−2) and maximum NUEg (51.3 gGW gN−1) in 2000, the corresponding values were 13.2 g m−2 and 61.6 gGW gN−1, respectively, in 2001. A similar phenomenon was also observed in winter wheat, which might be caused by the higher translocation of nitrogen to the panicles (CitationLi 2000).
Based on the present study, it can be concluded that there were significant differences in grain yield and yield components, and N absorption and use efficiency among RILs under hydroponic culture. In general, the higher-yielding rice plants displayed a larger number of panicles and spikelets per panicle, a higher filled grain percentage, higher total pre-NA and post-NA and NTA values, and higher NUEg values. At the same high-yielding level, there were large differences in the NTA and NUEg values. Therefore, it might be possible to breed higher-yielding cultivars with higher N use efficiency and provide a simple criterion for selecting and breeding such cultivars.
ACKNOWLEDGMENTS
The authors thank Dr Zhang QF, Huazhong Agricultural University, China, for providing the rice materials used in this study. The authors also thank Dr Kenji Nagata, National Agricultural Research. Center for the Tohoku Region and Dr Kenji Kato, Faculty of Agriculture, Okayama University for their valuable suggestions.
REFERENCES
- Mastsushima , S . 1976 . High-yielding Rice Cultivation-A Method for Maximizing Rice Yield Through “Ideal Plants” , Tokyo : Japan Scientific Societies Press .
- Murayama , N . 1967 . Nitrogen nutrition of rice plant . JARQ , 2 : 1 – 5 .
- Murayama , N . 1979 . “ The importance of nitrogen for rice production ” . In Nitrogen and Rice Edited by: Watanabe , I . 523 Los Baños, IRRI
- Tanaka , A , Navasevo , CV , Garcia , FT and Ramirez , E . 1964 . Growth habit of rice plant in the tropics and its effect on nitrogen response . InTech. Bull. , : 31 – 80 . IRRI, Los Baños
- Wada , G . 1969 . The effect of nitrogenous nutrition on the yield-determining process of rice plant . BullNatl InstAgric Sci , A16 : 1 – 167 . (in Japanese with English summary)
- Yoshida , S . 1981 . “ Rice plant characters in relation to yielding ability ” . In Fundamentals of Rice Crop Science , 127 – 230 . Los Baños : IRRI .
- Duxbury , JM , Harper , LA and Mosier , AR . 1993 . “ Contributions of agroecosystems to global climate change ” . In Agricultural Ecosystem Effects on Trace Gases and Global Climate Change , Edited by: Harper , LA , Mosier , AR , Duxbury , JM and Rolston , DE . 118 Madison, WI : Am. Agron. Soc. . ASA Spec. Publ. 55.
- Galbally , IE , Freney , JR , Muirhead , WA , Simpson , JR , Trecvitt , ACF and Chalk , PM . 1987 . Emission of nitrogen oxides (NOX) from a flooded soil fertilized with urea: Relation to other nitrogen loss processes . JAtmosChem , 5 : 343 – 365 .
- Cassman , KG , Kropff , MJ and Yan , Z . 1994 . “ A conceptual framework for nitrogen management of irrigated rice in high-yield environments ” . In Hybrid Rice Technology: New Development and Future Prospects Selected Papers , Edited by: Virmani , SS . 81 – 96 . Los Baños : IRRI .
- Datta , deSK and Buresh , RJ . 1989 . Integrated nitrogen management in irrigated rice . AdvAgron , 10 : 143 – 169 .
- Fillery , IRP and Vlek , PLG . 1986 . Reappraisal of the significance of ammonia volatilization as an N loss mechanism in flooded rice fields . FertRes , 9 : 78 – 98 .
- Schnier , HF , Dingkuhn , SK , Datta , deSK , Mengel , K and Faronilo , JE . 1990 . Nitrogen fertilization of direct-seeded vs. transplanted rice: I. Nitrogen uptake, photosynthesis, growth and yield . Crop Sci , 30 : 1276 – 1284 .
- Singh , U , Ladhab , JK , Castilloa , EG , Punzalanb , G , Tirol-Padre , A and Duqueza , M . 1998 . Genotypic variation in nitrogen use efficiency in medium-and long-duration rice . Field Crops Res , 58 : 35 – 53 .
- Broadbent , FE , Datta , deSK and Laureles , EV . 1987 . Measurement of nitrogen utilization efficiency in rice genotypes . Agron J , 79 : 786 – 791 .
- Inthapanya , P , Sipaseuth , SP , Sihathep , V , Chanphengsay , M , Fukai , S and Basnayake , J . 2000 . Genotype differences in nutrient uptake and utilization for grain yield production of rainfed lowland rice under fertilizer and non-fertilized conditions . Field Crops Res , 65 : 57 – 68 .
- Tirol-padre , A , Ladha , JK and Singh , U . 1996 . Grain yield performance of rice genotypes at suboptimal levels of soil N as affected by N uptake and utilization efficiency . Field Crops Res , 46 : 127 – 143 .
- Koutroubas , SD and Ntanos , DA . 2003 . Genotypic differences for grain yield and nitrogen utilization in Indica and Japonica rice under Mediterranean conditions . Field Crops Res , 83 : 251 – 260 .
- Tanksley , SD . 1993 . Mapping polygenes . AnnuRevGenet , 27 : 205 – 233 .
- Cao , GQ , Zhu , J , He , CX , Gao , YM and Wu , P . 2001 . QTL analysis for epistatic effects and QTL × environment interaction effects on final height of rice (Oryza sativaL.) . Acta GenetSin , 28 : 135 – 143 .
- Peng , K and Zhang , Q . 1999 . A cosmid library constructed to the elite rice cultivar Minghui63 . Acta Botanica Sinica , 41 : 337 – 339 .
- Liu , S , Lix , W , Li , X and He , Y . 2003 . Improvement of resistance to rice blast in Zhenshan 97 by molecular marker-aided selection . Acta Botanica Sinica , 45 : 1346 – 1350 .
- Yoshida , S , Forno , DA , Cock , JH and Gomez , KA . 1976 . “ Routine procedure for growing rice plants in culture solution ” . In Laboratory Manual for Physiological Studies of Rice , 2nd edn , Edited by: Yoshida , S , Forno , DA , Cock , JH and Gomez , KA . 61 – 66 . Los Baños : IRRI .
- Moll , RH , Kamprath , EJ and Jackson , WA . 1982 . Analysis and interpretation of factors which contribute to efficiency of nitrogen utilization . AgronJ , 74 : 562 – 564 .
- Ichii , M and Nakamura , M . 1990 . Heterosis for nutrient uptake in F1 rice hybrid-seedlings . JpnJCrop Sci , 59 : 140 – 145 . (in Japanese with English summary)
- Yao , Y , Yamamoto , Y Wang , Y . 2000b . Heterosis in numbers of differentiated, degenerated and surviving spikelets and their relations to dry matter production in F1 hybrids of rice . Soil SciPlant Nutr , 46 : 951 – 962 .
- Yamamoto , Y , Yoshida , T , Enomoto , T and Yoshikawa , G . 1991 . Characteristics for the efficiency of spikelet production and the ripening in high-yielding japonica–indica hybrid and semi-dwarf indica rice cultivars . JpnJCrop Sci , 60 : 365 – 372 . (in Japanese with English summary)
- Yao , Y , Yamamoto , Y Wang , Y . 2000c . Role of nitrogen regulation in sink and source formation of high-yielding rice cultivars . Soil SciPlant Nutr , 46 : 825 – 834 .
- Yoshida , S , Cock , JH and Parao , FT . 1972 . “ Physiological aspects of higher yields ” . In Rice Breeding , 455 – 469 . Los Baños : IRRI .
- Miller , BC , Hill , JE and Roberts , SR . 1991 . Plant population effects on growth and yield in water-seeded rice . AgronJ , 83 : 291 – 297 .
- Datta , deSK and Broadbent , FE . 1990 . Nitrogen-use efficiency of 24 rice genotypes on a deficient soil . Field Crops Res , 23 : 81 – 92 .
- Hasegawa , H . 2003 . High-yielding rice cultivars perform best even at reduced nitrogen fertilizer rate . Crop Sci , 43 : 921 – 926 .
- Higuchi , M and Yoshino , T . 1986 . Nitrogen-absorption ability of high-yielding rice plants in a paddy field . JpnJSoil SciPlant Nutr , 57 : 131 – 141 . (in Japanese)
- Jiang , L and Dai , Y . 2003 . Genotypic differences and valuation in nitrogen uptake and utilization efficiency in rice . Acta Phytoecologica Sinica , 27 : 466 – 471 . (in Chinese with English abstract)
- Motomatsu , J , Takebe , M and Yoneyama , T . 1988 . Physiological characteristics of high-yielding rice varieties: dry matter production and nutrient uptake . BullNatl AgricResCent , 12 : 1 – 11 .
- Yao , Y , Yamamoto , Y Wang , Y . 2000a . Macro-element absorption at maturity in relation to grain yield in high-yielding rice cultivars . Soil SciPlant Nutr , 46 : 815 – 824 .
- Seino , K . 1975 . Accumulative yield of dry matter of rice plant as influenced by nitrogen nutrient . Nippon Dojo Hiryogaku Zasshi , 46 : 303 – 307 . (in Japanese)
- Li , S . 2000 . Yield and nitrogen utilization of different variation in winter wheat under water . Agricultural Research in the Arid Areas , 18 : 41 – 44 . (in Chinese with English summary)