Abstract
The chemical composition of the hydrophobic acid fraction, which is adsorbed to XAD-8 resin at low pH and desorbed with high pH solution, of water-extractable organic matter in soil probably influences its environmental role and, therefore, it is important to recognize the differences in its chemical composition with season and soil horizon. Hydrophobic acid fractions were collected seasonally in 2004 from Oi, Oe/Oa, A and B horizons of Cambisol and were investigated using elemental analysis, high-performance size exclusion chromatography and 1H nuclear magnetic resonance (NMR) spectroscopy. Atomic ratios of H/C and O/C decreased with increasing soil depth in winter and summer, suggesting that the hydrophobic acid fraction from lower mineral horizons had lower saturated bond content and carbohydrate content than the fractions from upper organic horizons. Based on high-performance size exclusion chromatography, the molecular weight at peak maximum of the hydrophobic acid fraction decreased significantly with increasing soil depth. The 1H NMR spectra of the hydrophobic acid fraction showed remarkable changes. The signal intensities of carbohydrate protons (δ 3.0–4.3 p.p.m.) in the hydrophobic acid fraction from organic horizons decreased from winter to summer and increased in autumn, while the signal of aliphatic protons (δ 0–3.0 p.p.m.) increased and decreased. In all seasons of the year, the signal intensities of carbohydrate protons were greater in organic horizons and decreased significantly with depth in mineral soil, but the signal for aliphatic protons increased. Our results demonstrate that season and soil depth influence the chemical composition of the hydrophobic acid fraction of water-extractable organic matter.
INTRODUCTION
Water-soluble organic matter (WSOM) in soil is composed of both relatively high molecular weight humic substances and specific identifiable organic compounds, such as carbohydrates and peptides (CitationHerbert and Bertsch 1995). The humic substances are mainly included in the hydrophobic acid fraction (HoA) of WSOM, which is defined as the XAD-8 resin adsorbable fraction (CitationLeenheer 1981), and is the dominating constituent of bulk dissolved organic matter (DOM) in soil solutions (CitationNambu and Yonebayashi 1999). As HoA is preferentially adsorbed on soil over hydrophilic fractions (CitationJardine et al. 1989; CitationKaiser and Zech 1997), it can be a precursor of humic substances in the illuvial horizon (CitationGuggenberger and Zech 1994). It is also assumed that a part of HoA is exported to streams and constitutes aquatic humic substances.
Adsorption and desorption of HoA may control co-transport in geochemical, environmental and ecological processes because of its interactions with other solutes (CitationKalbitz et al. 2000; CitationZsolnay 1996). In addition, complexation of HoA and organic contaminants reduced
Table 1 Selected properties of the soil horizons investigated
The interactions of HoA with mineral phase (CitationKaiser 2003; CitationKaiser et al. 1997) and contaminants (CitationMcCarthy 1989; CitationRaber and Kögel-Knabner 1997) can be substantially influenced by the molecular properties (e.g. functional group composition, molecular size, polarity, aromatic content and molecular configuration) of HoA. Consequently, a comprehensive understanding of the chemical composition of HoA is necessary to discuss its functional properties. Furthermore, it is assumed that the functional properties of HoA might be subjected to changes in the chemical composition of HoA with season and soil depth (CitationAnderson et al. 1990; CitationKaiser et al. 2001). However, information on changes in the chemical composition of HoA with season and soil depth is still vague.
The objective of the present study was to elucidate the effects of seasonal variations and soil depth on the chemical composition of HoAs from a Dystric Cambisol under a broad-leaf forest in 2004. The HoA was extracted from soil by shaking extraction with water. We previously demonstrated that the operational conditions for water extraction, such as soil-to-solution ratio, shaking time and the storage method of soil samples, affected the chemical composition of HoA (D Asakawa et al., unpubl data, 2006). Thus, in the present study, the operational conditions were selected to obtain high yield and to avoid effects of sample storage on the chemical composition of HoA.
MATERIALS AND METHODS
Site description and sampling
The study site was located at Yamashiro, Kyoto Prefecture, Japan (34°47.16′ N, 135°50.48′ E, 188 m a.s.l.). The dominant tree species were Japanese oak (Quercus serrata Thunb.) and Aquifoliaceae (Ilex pedunculosa Miq.), and the ground vegetation was dominated by Sasa sp. (Pleioblastus chino Makino). The mean annual temperature and annual precipitation in 2004 were 16.0°C and 1,592 mm, respectively.
Soil samples (Oi, Oe/Oa, A and B horizons) were taken from the east-facing strong slope in winter (late February), spring (late May), summer (early August) and autumn (late October) 2004. In winter and autumn, each horizon sample was taken on separate days just before extraction. Soil sampling was carried out ≥ 2 days after precipitation. The soil samples were collected in plastic bags and placed in a cooler with ice for transport back to the laboratory. The soil was classified as a Dystric Cambisol (WRB-classification) derived from granite material. Some of the data on the soil properties are given in .
Preparation of water-extractable organic matter and the hydrophobic acid fraction
Water-extractable organic matter (WEOM) was extracted from Oi, Oe/Oa, A and B horizon samples stored in field-moist conditions at 1°C. Operational conditions were based on our previous report (D Asakawa et al., unpubl data, 2006). Except for the B horizon sample, the soil samples were extracted within 3 days of collection to avoid storage effects on the chemical composition of the HoAs. The B horizon sample was extracted within 1 week after collection. The Oi, Oe/Oa, A and B horizon samples were extracted with ultra-pure water at a soil-to-solution ratio of 1:80, 1:20, 1:5, and 1:5, respectively. All extractions were carried out on an oven-dry mass basis. The suspensions from Oi and Oe/Oa samples were shaken for 12 h, and those from A and B samples were shaken for 24 h on a reciprocal shaker at 110 strokes min−1 at 1°C. After centrifugation (20,000 g, 30 min), the extracts were filtrated through a pre-washed 0.45-µm pore cellulose acetate membrane filter (Advantec, Tokyo, Japan).
The HoA of WEOM was isolated using the method of CitationThurman and Malcolm (1981) with some modification. The extract was acidified to pH 2 with 6 mol L−1 HCl and passed through a glass column of the Supelite DAX-8 resin (Supelco, Bellefonte, PA, USA), which is comparable to the Amberlite XAD-8 resin (Rohm and Haas, Philadelphia, PA, USA). The HoA was eluted from resin with 0.1 mol L−1 NaOH followed by distilled water. The eluate was acidified with 6 mol L−1 HCl immediately and concentrated hydrofluoric acid (HF) was added to a final concentration of 0.3 mol L−1 HF. The mixture was shaken overnight at room temperature. This solution was passed through a column of DAX-8 resin again and HF was removed by rinsing the column with diluted HCl. The HoA was desorbed with 0.1 mol L−1 NaOH followed by distilled water. The HoA was protonated using an ion exchange column of AG MP-50 resin (Bio-Rad Laboratories, Hercules, CA, USA) and freeze-dried.
For quantitative analysis of carbon content in WEOM (WEOC) and in HoA (HoA-C), soil samples were extracted within 12 h after collection. The extraction was the same as that described above. After centrifugation and filtration, WEOC was determined using a Shimadzu TOC-V CPH total organic carbon analyzer (Shimadzu, Kyoto, Japan). The extract was acidified, passed through the DAX-8 column and eluted from resin with 0.1 mol L−1 NaOH. The HoA-C in the eluate was also analyzed. Carbon content for the non-HoA (non-HoA-C), mainly consisting of hydrophilic fractions, was calculated by subtracting HoA-C from WEOC. The experiments were carried out in triplicate. The content of organic carbon was expressed as the extracted amount of carbon per soil oven-dry mass.
Elemental analysis
The amounts of carbon, nitrogen and hydrogen in the HoAs and humic substances were determined using a Perkin-Elmer 240-C elemental analyzer (Perkin-Elmer Japan, Yokohama, Japan). Oxygen content was estimated by subtracting the sum of the C, H and N contents from the total organic matter content. All results were expressed on a moisture- and ash-free basis. Moisture and ash contents were determined by heating separate samples at 105°C for 24 h and by ignition in micro ceramic crucibles at 550°C for 6 h, respectively.
High-performance size exclusion chromatography
Estimating the molecular weight of humic molecules using high-performance size exclusion chromatography (HPSEC) is affected by the eluent and standardization materials and is still controversial. Therefore, the purpose of HPSEC analysis in the present study was to compare molecular size distribution of HoAs rather than to determine the absolute molecular weights of HoAs. The HPSEC was carried out using a high-performance liquid chromatograph system consisting of a Waters 600E system controller, a Waters 717 plus autosampler and a Waters 2487 dual wavelength absorbance detector (Waters Incorporated, Milford, MA, USA). A Shodex OHpak SB-803 HQ column (Showa Denko Company, Tokyo, Japan; 8.0 mm internal diameter × 300 mm) protected by a Shodex OHpak SB-G guard column (8.0 mm internal diameter × 50 mm) was used for the separations depending on relative molecular size. The columns were kept at 40°C in a Waters column oven. The eluent was 10 mmol L−1 sodium phosphate buffer (pH 7.0) containing acetonitrile in the volume ratio of 3:1, the flow rate was 0.8 mL min−1, and the injection volume was 20 µL. All solutions and buffers were prepared using analytical-grade chemicals and MilliQ water.
Two milligrams of the HoA was first suspended in 2 mL of MilliQ water and adjusted to pH 8.0–9.0 with diluted NaOH. After shaking overnight, a portion of the HoA solution was diluted with eluent to a concentration of 20 mg L−1. Before injection, the diluted HoA solution was passed through a 0.2-µm membrane filter (DISMIC-13HP; Advantec, Tokyo, Japan). Absorbance was measured at 260 nm and peaks were analyzed using Waters Millenium 32 Chromatography Manager version 3.06 software. The total effective column volume (V 0 + V i) was determined using acetone, and the void volume (V 0) was determined using Blue Dextran (2,000 kDa). Sodium polystyrene sulfonates (680, 356, 188, 86.5, 35.7, 15.8, 4.92 and 1.37 kDa; Polyscience Incorporated, Warrington, PA, USA) and sodium p-styrenesulfonate (184 Da; Wako Chemical, Osaka, Japan) were used as standards.
1H nuclear magnetic resonance spectroscopy
Liquid-state 1H nuclear magnetic resonance (NMR) spectra of the HoA solutions were recorded on a Bruker Avance 500 spectrometer (Bruker GmbH, Karlsruhe, Germany) using sample tubes 5 mm in diameter. A solution of HoA was prepared by suspending 30–50 mg in 0.4 mL of 0.5 mol L−1 NaOD. For the chemical shifts, sodium 3-trimethylsilylpropionate-2,2,3,3, D4 (TMSP; Euriso-top, Saint Aubin, France) was used as a reference. The 1H NMR spectra were recorded under the following conditions: spectrometer frequency, 500.130 MHz; homo-gated decoupling; pulse width, 14.0 µs (90°); acquisition time 5.4 s; pulse delay 4.8 s; line broadening factor, 5 Hz. The peak of residual protons of water (HOD; 4.8 p.p.m.) was irradiated. Eight scans were accumulated for each sample.
Chemical shift assignments for 1H NMR spectroscopy are referred to CitationKawahigashi et al. (1996), that is,
the 1H NMR spectra were broadly divided into five regions: 0.0–1.0 p.p.m. (Hγ, protons on terminal methyl groups attached to saturated aliphatic protons), 1.0–1.6 p.p.m. (Hβ, protons on methylene β attached to olefins or aromatic rings), 1.6–3.0 p.p.m. (Hα, protons on methyl and methylene α attached to aromatic carbons, carbonyl groups, ester groups and olefins), 3.0–4.3 p.p.m. (HC–O, protons on carbons attached to oxygen-sugars, olefins and methoxyl groups); 6.0–9.0 p.p.m. (Har, protons attached to carbons of heteroaromatic and aromatic rings and to carbonyl groups bonded to electronegative groups). Furthermore, the chemical shift region between 0 and 3.0 p.p.m. was assigned to aliphatic protons (Hal; Hγ + Hβ + Hα).RESULTS AND DISCUSSION
Yields of carbon contents in water-extractable organic matter and in the hydrophobic acid fraction
WEOC and HoA-C showed remarkable variability, with WEOC ranging from 33.2 to 6,138 mg C kg−1 soil and HoA-C ranging from 7.6 to 1,958 mg C kg−1 soil. Organic carbon contents data are shown in . The amount of WEOC from each horizon showed seasonal variation, with higher amounts recorded in summer and autumn than in winter and spring. This result is similar to seasonal variations in the fluxes of carbon in DOM in lysimeter solutions from organic forest floor layers (CitationKaiser et al. 2001). However, CitationHishi et al. (2004) observed relatively high yields of WEOC in surface mineral soil of a broad-leaved deciduous forest collected in spring and summer. This difference may result from soil moisture conditions and temperature, which largely control the production of soluble organic carbon (CitationChrist and David 1996; CitationKaiser et al. 2001). As CitationHishi et al. (2004) studied a mountainous area covered with snow until late April, the high yield of WEOC in spring may be attributed to snowmelt and increasing soil temperature.
The amount of HoA-C from the Oi horizon comprised 26–40% WEOC and showed similar seasonal variation to the WEOC (). In spring, WEOC from the Oe/Oa, A and B horizons contained higher rates of HoA-C than in the other seasons. The maximum HoA-C from organic horizons occurred in autumn, and from A horizon occurred in summer. The HoA-C from the B horizon was very low throughout the year.
shows that WEOC and HoA-C decreased with depth. However, the highest yield was obtained from A horizon when expressed as HoA-C m−2. The percentage of HoA-C in WEOC increased with depth from Oi to A horizon, but there were very low percentages (16–29%) in B horizon, except in spring. Significant decreases in the HoA-C and in the proportion of HoA-C in WSOC in lower mineral horizons have often been observed in soil solution studies (CitationCronan and Aiken 1985; CitationFunakawa et al. 1993; CitationQualls and Heines 1991).
Elemental analysis
The elemental composition of HoAs is shown in . The weight percentage of the C, H, N and O contents of HoAs ranged from 49.9 to 56.0%, 4.28 to 5.22%, 1.75 to 3.39% and 35.4 to 42.8%, respectively. The HoAs from each horizon in the present study contained more C but less O than the HoAs of WSOM from another type of soil (CitationKaiser 2003; CitationLeinweber et al. 2001). This may be because different materials were used or because of the different preparation methods of HoA (collection method of WSOM with or without HCl-HF treatment).
As shown in , seasons showed no consistent trends in the C and H contents of HoAs from the Oi and Oe/Oa horizons. In autumn, a lower O content was observed in the Oi horizon sample than in the other seasons. The N content of the Oi horizon sample increased from 1.75% in winter to 2.31% in autumn. However, the HoAs from the Oe/Oa and A horizons exhibited higher N content in winter. The highest values of the C and H contents of HoAs from the A horizon were observed in winter.
Table 2 Elemental composition of the hydrophobic acid fraction obtained from different horizons
Figure 2 Changes in the atomic ratio of (a) H/C, (b) O/C and (c) N/C of the hydrophobic acid fraction obtained from different horizons. •, winter; ○, spring; ▾, summer; ▿, autumn.
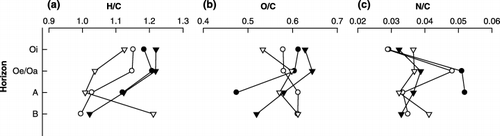
The elemental composition of HoAs changed with increasing soil depth, but different trends were observed in each season. We calculated the atomic ratios of H/C, O/C, N/C and O/H, which have been commonly used as indicators of structural characteristics of coal and humic substances (CitationKumada 1987). In general, the H/C ratio is an indicator of the saturated bond content. shows that the H/C ratio decreased with increasing soil depth, except for the HoA from the B horizon collected in autumn. This indicated that the HoAs from organic horizons show more saturated bond content than those from lower mineral horizons. The O/C ratio, which is assumed to indicate the content of carbohydrate and degree of oxidation, also decreased with soil depth in winter and summer (). This suggests that the HoAs derived from lower mineral horizons had lower carbohydrate contents. The HoAs from the Oe/Oa horizon had a greater N/C ratio than those from the other horizons, except in winter (). No constant relationship was detected between the O/H ratio and soil depth.
High-performance size exclusion chromatography
shows the size exclusion chromatograms of the HoAs from each horizon collected in winter. The chromatograms of all the HoAs had a broad peak with subtle shoulders and small subpeaks. The molecular weight at peak maximum (Mp) of HoAs from each soil horizon ranged from 924 to 1,665 Da (). This result is comparable to the results from several authors investigating the molecular weight of water-soluble humic substances. CitationBerdén and Berggren (1990) reported that the Mp of humic
Table 3 Molecular weight at peak maximum of the hydrophobic acid fraction obtained from different horizons
Figure 3 Size exclusion chromatograms of the hydrophobic acid fraction obtained from different horizons collected in winter.
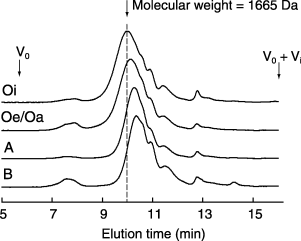
As shown in , no significant seasonal variation in the Mp values of HoAs was observed, although the HoAs collected in winter showed slightly larger Mp values than the HoAs collected in other seasons. However, the molecular size distribution of the HoAs varied with soil depth. The mean Mp values of HoAs decreased significantly with depth from 1,579 ± 64 in the Oi horizon to 1,083 ± 111 in the B horizon ().
Although analyses of another fraction of WSOM and/or bulk WSOM are necessary to evaluate the behavior of HoA, selective sorption and biodegradation of HoA might affect its molecular weight. Several studies indicate that higher molecular weight organic matter distributed in DOM and humic substances is preferentially adsorbed by soil minerals (CitationDawson et al. 1978; CitationGuo and Chorover 2003; CitationKaiser et al. 2002; CitationOchs et al. 1994). In addition, CitationAmon and Benner (1994, Citation1996) suggested that fresh macromolecules of aquatic DOM are decomposed by bacterial utilization to less bioreactive molecules that are lower in molecular weight. The decrease we observed in the Mp values of HoA from lower horizons may be consistent with these hypotheses.
Interestingly, changes in elution time of subtle shoulders and small subpeaks were not observed, but the relative intensities of these small peaks increased with depth. These results suggest that low molecular weight components of HoA may have a low affinity for mineral soil.
1H nuclear magnetic resonance spectroscopy
shows 1H NMR spectra of the HoAs from each horizon sampled in winter, spring, summer and autumn. All spectra consist of peaks in the same chemical shift regions. Similar peaks are found in 1H NMR spectra of terrestrial and aquatic fulvic and humic acids (CitationFujitake et al. 2003; CitationMalcolm 1990) and in those of bulk DOM from organic forest floors (CitationKaiser et al. 2002). The spectra of the HoA from the B horizon collected in winter and spring resembled that of humic substances isolated from fresh water, where resonances near 1 p.p.m. are more prominent (CitationMalcolm 1990). Consequently, the HoA in the B horizon may contribute to the formation of aquatic humic substances in fresh water, although allochthonous aquatic humic substances can consist of HoAs in both surface runoff water and subsurface lateral flow.
Signal intensities of each type of proton showed seasonal variation (), particularly in the HC–O (3.0–4.3 p.p.m.) region, indicative of carbohydrate structures, and the Hal (0–3.0 p.p.m.) region, indicative of aliphatic structures. As shown in , this observation was confirmed by integration of the signal areas of 1H resonance. The distribution of each type of proton species in HoAs from the Oi and Oe/Oa horizons showed similar seasonal patterns. The proportion of HC–O in HoA from the Oi horizon decreased from 36.7% in winter to 29.0% in summer, and increased to 33.9% in autumn. Comparing 1H NMR spectra of WEOM before and after incubation, CitationKalbitz et al. (2003b) observed preferential biodegradation of carbohydrates and relative enrichment of aromatic compounds in WEOM. Therefore, the decrease in the proportion of HC–O in HoAs could be explained by an increase in microbial activity from winter to summer, unless another fraction of WEOM was measured. However, as the proportion of HC–O decreased, no increase
Figure 4 1H nuclear magnetic resonance spectra of the hydrophobic acid fraction obtained from different horizons. Residual protons of water (HOD), TMSP, trimethylsilylpropionate-2,2,3,3.
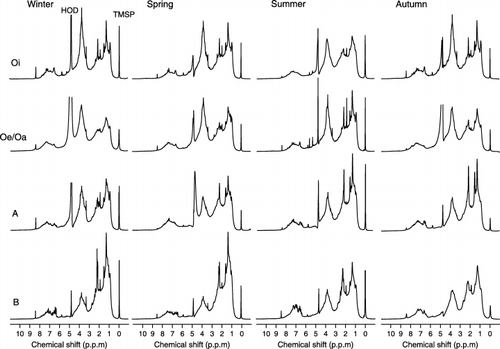
Seasonal variations in the distribution of proton species in HoA from the A horizon showed similarity to those in organic horizons. The proportion of HC–O in HoA from the A horizon decreased from 29.6% in winter to 22.8% in autumn, whereas the proportion of Hal increased (). In the B horizon, the proportion of HC–O in HoA increased from 15.6% in spring to 23.1% in autumn and the proportion of Hal was higher in winter and spring than in summer and autumn (). This result indicated that the chemical composition of HoA from the B horizon showed different seasonal variation from the HoA in the organic horizon. This difference might be related to the relative insensitivity of the lower horizon to changes in temperature or microbial activity and suggest another factor controlling seasonal variations in the lower horizon.
In all seasons, relative signal intensities for HC–O were greater in organic horizons and decreased significantly with depth in mineral soil, whereas signal intensities for Hal increased (). The changes in the proportions of HC–O and Hal in HoAs with depth are shown in . In winter, the proportion of HC–O in HoAs decreased with depth from 36.7% in the Oi horizon to 17.8% in the B horizon, whereas the proportion of Hal increased from 51.8 to 72.6% (). These same patterns were found in samples from the other seasons, although the changes in the proportion of HC–O in summer and autumn samples were relatively minor. Similar results have been reported by CitationUssiri and Johnson (2003), who observed decreasing O-alkyl carbon and increasing alkyl carbon with increasing depth in whole soils, humic acids and humin. CitationUssiri and Johnson (2003) suggested that an increase in alkyl carbon is likely to result from a selective preservation and a synthesis of alkyl structures by microorganisms. Therefore, the changes in the chemical composition of HoA with increasing soil depth might result from not only adsorption to mineral soil but also biodegradation.
Table 4 Distribution of proton species in the hydrophobic acid fraction obtained from different horizons
Conclusions
The present study shows the differences in chemical characteristics of HoA in water-extractable organic matter from different soil horizons of a Dystric Cambisol. With increasing soil depth, the relative molecular weights of HoAs estimated using HPSEC decreased. Furthermore, 1H NMR spectroscopy demonstrated that the proportion of carbohydrate protons (HC–O) in HoA significantly decreased with soil depth, while the proportion of aliphatic protons (Hal) increased. These changes in chemical composition of HoA appear to result from selective adsorption to minerals and/or biodegradation. These results reveal the differences in functional properties of HoA among horizons and the selective transportation of contaminants associated with HoA. In addition, seasonal variations in chemical composition of HoA were characterized by 1H NMR spectroscopy. The proportion of HC–O in HoA from organic horizons decreased from winter to summer, and slightly increased in autumn. However, in the lower horizon, the seasonal variations in the distribution of proton species in HoA showed a different trend. The seasonal variations in the amount and chemical composition of HoA can affect the functional properties of HoA in the environment.
These changes in the chemical composition of HoA with season and soil depth could influence the geochemical, environmental and ecological processes of HoA in soil. Therefore, it is important to recognize the differences in sampling season and soil depth as well as the origin of HoA when we assess the functional properties of HoA in detail.
ACKNOWLEDGMENT
This research was supported by a Grant-in-Aid for Scientific Research (B-15380107, 2003–2005, B-1530001, 2003–2005 and B-16380049, 2004–2006) from The Japan Society for the Promotion of Science.
Notes
Present address: Faculty of Horticulture, Minamikyushu University, Miyazaki 884-0003, Japan
REFERENCES
- Herbert , BE and Bertsch , PM . 1995 . “ Characterization of dissolved and colloidal organic matter in soil solution: A review ” . In Carbon Forms and Functions in Forest Soils , Edited by: Kelly , JM and McFee , WW . 63 – 88 . Madison : Soil Science Society of America .
- Leenheer , JA . 1981 . Comprehensive approach to preparative isolation and fractionation of dissolved organic carbon from natural waters and wastewaters . EnvironSciTechnol , 15 : 578 – 587 .
- Nambu , K and Yonebayashi , K . 1999 . Acidic properties of dissolved organic matter leached from organic layers in temperate forests . Soil SciPlant Nutr , 45 : 65 – 77 .
- Jardine , PM , Weber , NL and McCarthy , JF . 1989 . Mechanisms of dissolved organic carbon adsorption on soil . Soil SciSocAmJ , 53 : 1378 – 1385 .
- Kaiser , K and Zech , W . 1997 . Competitive sorption of dissolved organic matter fractions to soil and related mineral phases . Soil SciSocAmJ , 61 : 64 – 69 .
- Guggenberger , G and Zech , W . 1994 . Dissolved organic carbon in forest floor leachates: simple degradation products or humic substances? . SciTotal Environ , 152 : 37 – 47 .
- Kalbitz , K , Solinger , S , Park , JH , Michalzik , B and Matzner , E . 2000 . Controls on the dynamics of dissolved organic matter in soils: a review . Soil Sci , 165 : 277 – 304 .
- Haitzer , M , Hoss , S , Traunspurger , W and Steinberg , C . 1999 . Relationship between concentration of dissolved organic matter (DOM) and the effect of DOM on the bioconcentration of benzo[a]pyrene . Aquatic Toxicol , 45 : 147 – 158 .
- Kukkonen , J , MacCarthy , JF and Oikari , A . 1990 . Effects of XAD-8 fractions of dissolved organic carbon on the sorption and bioavailability of organic micropollutants . ArchEnvironContamToxicol , 19 : 551 – 557 .
- McCarthy , JF , Roberson , LE and Burrus , LW . 1989 . Association of benzo(a)pyrene with dissolved organic matter: prediction of Kdom from structural and chemical properties of the organic matter . Chemosphere , 19 : 1911 – 1920 .
- Jandl , R and Sollins , P . 1997 . Water-extractable soil carbon in relation to the belowground carbon cycle . BiolFertlSoils , 25 : 196 – 201 .
- Kalbitz , K , Schmerwitz , J , Schwesig , D and Matzner , E . 2003a . Biodegradation of soil-derived dissolved organic matter as related to its properties . Geoderma , 113 : 273 – 291 .
- Kaiser , K . 2003 . Sorption of natural organic matter fractions to goethite (α-FeOOH): effect of chemical composition as revealed by liquid-state 13C NMR and wet-chemical analysis . OrgGeochem , 34 : 1569 – 1579 .
- Kaiser , K , Guggenberger , G , Haumaier , L and Zech , W . 1997 . Dissolved organic matter sorption on subsoils and minerals studied by 13C-NMR and DRIFT spectroscopy . EurJSoil Sci , 48 : 301 – 310 .
- Raber , B and Kögel-Knabner , I . 1997 . Influence of origin and properties of dissolved organic matter on the partition of polycyclic aromatic hydrocarbons (PAHs) . EurJSoil Sci , 48 : 443 – 455 .
- Anderson , HA , Hepburn , A , Miller , JD , Stewart , M , Ferrier , RC and Walker , TAB . 1990 . Humic substances of surface waters: Litter and soil throughflow relationships in two forested ecosystems . AnalChimActa , 232 : 3 – 10 .
- Kaiser , K , Guggenberger , G , Haumaier , L and Zech , W . 2001 . Seasonal variations in the chemical composition of dissolved organic matter in organic forest floor layer leachates of old-growth Scots pine (Pinus sylvestrisL.) and European beech (Fagus sylvaticaL.) stands in northeastern Bavaria, Germany . Biogeochem , 55 : 103 – 143 .
- Thurman , EM and Malcolm , RL . 1981 . Preparative isolation of aquatic humic substances . EnvironSciTechnol , 15 : 463 – 466 .
- Kawahigashi , M , Fujitake , N and Takahashi , T . 1996 . Structural information obtained from spectral analysis (UV-VIS, IR, 1H NMR) of particle size fractions in two humic acids . Soil SciPlant Nutr , 42 : 355 – 360 .
- Hishi , T , Hirobe , M , Tateno , R and Takeda , H . 2004 . Spatial and temporal patterns of water-extractable organic carbon (WEOC) of surface mineral soil in a cool temperate forest ecosystem . Soil BiolBiochem , 36 : 1731 – 1737 .
- Christ , MJ and David , MB . 1996 . Temperature and moisture effects on the production of dissolved organic carbon in a spodosol . Soil BiolBiochem , 28 : 1191 – 1199 .
- Cronan , CS and Aiken , GR . 1985 . Chemistry and transport of soluble humic substances in forested watersheds of the Adirondack Park, New York . GeochimCosmochimActa , 49 : 1697 – 1705 .
- Funakawa , S , Yonebayashi , K and Kyuma , K . 1993 . Characteristics of humic substances and dynamics of dissolved organic matter in forest soils in northern Kyoto with special reference to their pedogenetic processes . Soil SciPlant Nutr , 39 : 169 – 181 .
- Qualls , RG and Haines , BL . 1991 . Geochemistry of dissolved organic nutrients in water percolating through a forest ecosystem . Soil SciSocAmJ , 55 : 1112 – 1123 .
- Leinweber , P , Schulten , HR , Kalbitz , K , Meißner , R and Jancke , H . 2001 . Fulvic acid composition in degraded fenlands . JPlant NutrSoil Sci , 164 : 371 – 379 .
- Kumada , K . 1987 . “ Elementary composition of humic acids and fulvic acids ” . In Chemistry of Soil Organic Matter , Edited by: Kumada , K . 70 – 94 . Tokyo : Japan Scientific Societies Press .
- Berdén , M and Berggren , D . 1990 . Gel filtration chromatography of humic substances in soil solutions using HPLC-determination of the molecular weight distribution . JSoil Sci , 41 : 61 – 72 .
- Aoyama , M . 2002 . Characterization of water-soluble organic matter in soils by size exclusion chromatography and fractionation with polyvinylpyrrolidone . Soil SciPlant Nutr , 48 : 475 – 481 .
- Dawson , HJ , Ugolini , FC , Hrutfiord , BF and Zachara , J . 1978 . Role of soluble organics in the soil processes of a Podzol, central Cascades, Washington . Soil Sci , 126 : 290 – 296 .
- Guo , M and Chorover , J . 2003 . Transport and fractionation of dissolved organic matter in soil columns . Soil Sci , 168 : 108 – 118 .
- Kaiser , K , Guggenberger , G , Haumaier , L and Zech , W . 2002 . The composition of dissolved organic matter in forest soil solutions: changes induced by seasons and passage through the mineral soil . OrgGeochem , 33 : 307 – 318 .
- Ochs , M , Osoviç , B and Stumm , W . 1994 . Coordinative and hydrophobic interaction of humic substances with hydrophilic Al2O3and hydrophobic mercury surfaces . GeochimCosmochimActa , 58 : 639 – 650 .
- Amon , RMW and Benner , R . 1994 . Rapid cycling of high-molecular-weight dissolved organic matter in the ocean . Nature , 369 : 549 – 552 .
- Amon , RMW and Benner , R . 1996 . Bacterial utilization of different size classes of dissolved organic matter . LimnolOceanogr , 41 : 41 – 51 .
- Fujitake , N , Kusumoto , A , Yanagi , Y , Suzuki , T and Otsuka , H . 2003 . Properties of soil humic substances in fractions obtained by sequential extraction with pyrophosphate solutions at different pHs III. FT-IR and 1H NMR spectra of humic acids . Soil SciPlant Nutr , 49 : 347 – 353 .
- Malcolm , RL . 1990 . The uniqueness of humic substances in each of soil, stream and marine environments . AnalChimActa , 232 : 19 – 30 .
- Kalbitz , K , Schwesig , D Schmerwitz , J . 2003b . Changes in properties of soil-derived dissolved organic matter induced by biodegradation . Soil BiolBiochem , 35 : 1129 – 1142 .
- Hatcher , PG , Spiker , EC , Szeverenyi , NM and Maciel , GE . 1983 . Selective preservation and origin of petroleum-forming aquatic kerogen . Nature , 305 : 498 – 501 .
- Wilson , MA , Heng , S , Goh , KM , Pugmire , RJ and Grant , DM . 1983 . Studies of litter and acid insoluble soil organic matter fractions using 13C-cross polarization nuclear magnetic resonance spectroscopy with magic angle spinning . JSoil Sci , 34 : 83 – 97 .
- Ussiri , DAN and Johnson , CE . 2003 . Characterization of organic matter in a northern hardwood forest soil by 13C NMR spectroscopy and chemical methods . Geoderma , 111 : 123 – 149 .
- Zsolnay , A . 1996 . “ Dissolved humus in soil waters ” . In Humic Substances in Terrestrial Ecosystems , Edited by: Piccolo , A . 171 – 223 . Amsterdam : Elsevier .
- Zsolnay , Á . 2003 . Dissolved organic matter: artefacts, definitions, and functions . Geoderma , 113 : 187 – 209 .
- Present address: Faculty of Horticulture, Minamikyushu University, Miyazaki 884-0003, Japan