Abstract
A neutral phosphate buffer (NPB) extraction method has been used to estimate the amount of available N in soil. However, the properties of soil NPB-extractable organic N have not been fully elucidated. The purpose of the present study was to characterize the properties of organic matter in the NPB extracts of soils. The NPB extracts were obtained from three soil samples, and the organic matter in the extracts was separated into three fractions according to its solubility in acid and adsorption onto polyvinylpyrrolidone (PVP). High-performance size exclusion chromatography (HPSEC) with ultraviolet (UV) and fluorescence detections was applied to the NPB extracts and their fractions. The HPSEC analysis of the NPB extract revealed the presence of a single broad peak, irrespective of the detection methods. The broad peak was identified as humic substances using the on-flow measurements of UV absorption spectra and fluorescence emission spectra. Among the fractions, the PVP-non-adsorbed fulvic acid (FA) fraction accounted for the largest proportion of organic C or N in the NPB extract, followed by the PVP-adsorbed FA and humic acid (HA) fractions. The peak of humic substances was observed for all fractions using HPSEC with the on-flow measurement of UV absorption and fluorescence emission spectra. The molecular weight of the humic substances varied with each fraction. When the Coomassie Blue-reactive substances (CBRS) were quantified using a Bradford protein assay, they were detected in the NPB extract and almost half were distributed in the PVP-non-adsorbed FA fraction. However, humic substances were considered to be the main constituents of CBRS in the soil NPB extract because of their reactivity with Coomassie Blue and the absence of proteinaceous materials. Furthermore, an incubation experiment revealed that the organic matter available to microorganisms was included in the HA and PVP-non-adsorbed FA fractions. Based on the HPSEC analysis of the NPB extracts and their fractions, it was observed that the humic substances in the NPB extract, particularly in the HA and PVP-non-adsorbed FA fractions, were available to microorganisms.
INTRODUCTION
A neutral phosphate buffer (NPB) extraction method was developed by CitationHiguchi (1981) for evaluating the amount of available N in soil. The major constituents of NPB-extractable organic N had been assumed to consist of proteinaceous materials (CitationHiguchi 1982; CitationMatsumoto et al. 2000; CitationSaito 1988). Recently, CitationMurase et al. (2003) have isolated proteins from the phosphate buffer (pH 6) extracts of several greenhouse soils using sodium dodecylsulfate-polyacrylamide gel electrophoresis after removal of bacterial cells using a membrane filter and purification by precipitation with trichloroacetic acid. They analyzed soils other than greenhouse soils, but did not detect any proteins in the phosphate buffer extract. This result suggested that the proteins isolated by CitationMurase et al. (2003) were produced under conditions unique to the greenhouse soils.
To quantify the proteinaceous materials in the NPB extracts of soils, CitationHiguchi (1982) used an ultraviolet (UV) absorption method. However, UV-absorbing substances, particularly humic substances and nitrate, in the NPB extracts from soils may interfere with the protein assay by UV absorption. In fact, the soil water extracts contained humic substances and nitrate (CitationAoyama 1996, Citation2002). In contrast, CitationMatsumoto et al. (2000) estimated the amount of proteins in the NPB extracts of 20 soil samples using the Bradford protein assay (CitationBradford 1976). However, because this method depends on the change in absorbance in Coomassie Blue G-250 upon binding of protein, interference by humic substances (CitationOgunseitan 1993) and phenols (CitationMattoo et al. 1987) might occur.
CitationMatsumoto et al. (2000) applied high-performance size exclusion chromatography (HPSEC) with UV detection at 280 nm to the NPB extracts of soil samples and observed one major peak on the HPSEC chromatograms, irrespective of the soil type. They considered that the peak corresponded to the peak of a “protein-like substance” because the peak area was correlated with the amounts of both NPB-extractable organic N and proteins estimated using the Bradford assay (CitationBradford 1976). A broad single peak was usually observed on the chromatograms when HPSEC with UV detection was applied to the water extracts of soils (CitationAoyama 1996; Citation2002; CitationBerdén and Berggren 1990). This peak was considered to be derived from humic substances (CitationAoyama 2002). CitationOgawa et al. (1989) observed that the NPB extract from soils generally showed a yellow to yellowish-brown color and that the color intensity increased with increasing concentration of organic N in the extract. They further demonstrated that the absorbance at 420 nm of the NPB extract was highly correlated with the amount of NPB-extractable organic N. Therefore, it is reasonable to assume that the single peak observed on the HPSEC chromatograms of the NPB extracts of soils was derived from the humic substances.
The purpose of the present study was to characterize the properties of organic matter in the NPB extracts of soils. In this study, the organic matter in the NPB extracts obtained from three soil samples was separated into humic acid (HA), polyvinylpyrrolidone (PVP)-adsorbed fulvic acid (FA) and PVP-non-adsorbed FA fractions according to its solubility in acid and adsorption onto PVP. HPSEC combined with the on-flow measurements of UV absorption spectra and fluorescence emission spectra was used to characterize the organic matter extracted using NPB. Moreover, an incubation experiment was carried out to identify the organic matter fraction available to microorganisms.
MATERIALS AND METHODS
Soil samples
Samples from three surface soils (0–10 cm) listed in were used for the experiments. The Fujisaki soil was collected from a chestnut orchard located at the Hirosaki University Farm, Aomori Prefecture, Japan. The Fukushima soil was collected from an upland field located at the Department of Upland Farming, National Agricultural Research Center for Tohoku Region, Fukushima Prefecture, Japan. The Nayoro soil was collected from an upland field that had received cattle manure at a rate of 40 Mg ha−1 year−1 for 7 years at the Nayoro Farm of the Hokkaido Prefectural Central Agricultural Experiment Station, Hokkaido Prefecture, Japan. The soil samples were air-dried and ground to pass through a 2-mm mesh sieve.
Preparation and fractionation of NPB extracts
Each soil sample corresponding to 60 g oven-dry soil was shaken with 300 mL of 67 mmol L−1 phosphate buffer (pH 7.0) for 1 h and centrifuged at 8,000 g for 10 min. The supernatant was filtered with an Advantec No. 6 filter paper (Advantec Toyo Company, Tokyo, Japan). This extract was designated as the NPB extract and further fractionated into three fractions: HA, PVP-adsorbed FA and PVP-non-adsorbed FA fractions. A 200 mL portion of each NPB extract was treated with H2SO4 (1:5) to reach a pH of 1.0 and centrifuged at 8,000 g for 30 min. The precipitate was washed with a small volume of distilled deionized water and dissolved in 10 mL of 0.1 mol L−1 NaOH. The solution was adjusted to pH 8.0 with 0.5 mol L−1 H2SO4 and made up to a total volume of 50 mL with distilled deionized water (HA fraction). The supernatant and the washing were combined and treated with PVP (1 g 100 mL−1) and shaken for 1 h (CitationLowe 1980). The PVP was separated by filtering through an Advantec No. 3 filter paper and washed with 10 mL of 0.25 mol L−1 H2SO4. The filtrate and the washing were combined and adjusted to pH 8.0 with 1 mol L−1 NaOH and made up to a total volume of 500 mL with distilled deionized water (PVP-non-adsorbed FA fraction). The PVP-adsorbed fraction
Table 1 Properties of the soil samples used
Chemical analysis of NPB extracts and their fractions
Organic C content was determined with a TOC analyzer (TOC-VE; Shimadzu Company, Kyoto, Japan). Total N content was determined using the alkaline persulfate oxidation-High Performance Liquid Chromatography (HPLC) method (CitationAoyama and Nagumo 1996). The amounts of NH4-N and NO3-N were analyzed using the methods of CitationMizushima (1984) and CitationAoyama and Nagumo (1996), respectively, using HPLC. Organic N content was calculated by subtracting the inorganic N (NH4-N + NO3-N) content from the total N content. The amount of Coomassie Blue-reactive substances (CBRS) was estimated using the Bradford protein assay (BioRad protein assay kit; Richmond, CA, USA) using bovine serum albumin as a standard.
HPSEC
The HPSEC was carried out using the same operating conditions as those used in a previous study (CitationAoyama 2002). The chromatograms were monitored by absorbance at 210, 280 and 400 nm using a JASCO UV1575 UV-Vis detector (JASCO Company, Tokyo, Japan) and by fluorescence at an excitation wavelength of 360 nm and an emission wavelength of 450 nm using a JASCO FP-920 fluorescence detector (JASCO). At the same time, the on-flow measurements of UV absorption spectra and fluorescence emission spectra (excitation at 254 nm) were carried out for individual peaks. For HPSEC, the NPB extracts and their fractions were mixed with an equal volume of 100 mmol L−1 phosphate buffer (pH 8.0), containing 600 mmol L−1 NaCl, and with half a volume of CH3CN then filtered through a 0.45-µm membrane filter (DISMIC-13CP; Advantec) and injected using a 100-µL injection loop. To estimate the molecular weight (MW), the columns were calibrated using polyethylene glycols as MW standards (CitationAoyama 2002). The void volume (V0) and the total effective column volume (V0 + Vi) were determined using Blue Dextran (Sigma-Aldrich Japan, Tokyo, Japan) and acetone, respectively. For an aqueous solution of bovine serum albumin (5 mg mL−1), HPSEC was carried out and UV absorption and fluorescence emission spectra were measured as described for the NPB extracts.
Incubation experiment
A 200 mL portion of the NPB extract from the Fujisaki soil was placed in a 300-mL beaker and inoculated with 1 mL of a 1:10 aqueous suspension of the fresh soil collected from the chestnut orchard located at the Hirosaki University farm. The beaker was covered with aluminum foil and incubated for 14 days at 25°C. The incubation was carried out in triplicate. Each beaker was weighed every 2–3 days and the weight loss was compensated by the addition of distilled deionized water. To prevent the extract from being subjected to anaerobic conditions, each beaker was swirled by hand every 2–3 days. After incubation, the extract was centrifuged at 16,000 g for 10 min because it was turbid. The supernatant solution was fractionated to the HA, PVP-adsorbed FA and PVP-non-adsorbed FA fractions. For the unfractionated extract and fractions, the amounts of organic C and N were determined, and HPSEC was carried out.
RESULTS AND DISCUSSION
HPSEC of NPB extracts
When HPSEC with UV detection was applied to the soil NPB extracts, a broad peak was eluted at an elution volume of 7.3 mL, irrespective of the detection wavelengths (). The elution volume corresponded to a MW of 1,500 Da using polyethylene glycols as MW standards. The intensity of the broad peak decreased with increasing wavelength. On the chromatograms recorded at 210 nm, a sharp peak was observed at an elution volume of approximately 11.0 mL. This peak was identified as NO− 3 by comparison with the authentic solution. HPSEC analysis of water-soluble humic substances usually reveals the presence of a broad peak, reflecting the polydispersive nature of humic substances (CitationArtinger et al. 2000; CitationO’Loughlin and Chin 2001). Humic substances exhibit featureless spectra in the UV and visible regions, with the absorbance decreasing as the wavelength increases (CitationSchnitzer and Khan 1972). The UV absorption spectrum obtained for the broad peak was typical of humic substances (). In addition, the UV absorption spectrum of protein (bovine serum albumin) showed an absorbance peak at 280 nm, which was different from that of the broad peak that appeared when HPSEC was carried out ().
When monitored by fluorescence at the near-optimum excitation and emission wavelengths (excitation wavelength = 360 nm, emission wavelength = 450 nm) of soil FAs (CitationSenesi et al. 1991), a broad peak was eluted later than that detected by UV absorption (). This phenomenon has been observed for soil and aquatic humic substances (CitationAlberts and Takács 2004; CitationAoyama 2001; CitationBuckau et al. 2001). The fluorescence emission spectra (excitation at 254 nm) of the peaks showed an emission maximum at approximately 445 nm (). The maximum emission wavelength had been found to be located at 440–450 nm for river water FAs (CitationMiano et al. 1988; CitationNagao et al. 1999) and at 446–455 nm for soil FAs
Figure 1 Size exclusion chromatograms of the whole neutral phosphate buffer (NPB) extracts. The moluecular weights (MWs) indicated in the figure were estimated using polyethylene glycols as standards. AU, absorbance unit; RFU, relative fluorescence unit.
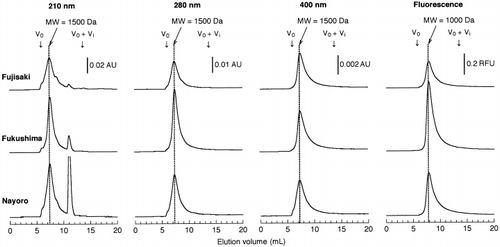
Figure 2 Ultraviolet (UV) absorption spectra and fluorescence emission spectra obtained for bovine serum albumin and the broad peaks of whole neutral phosphate buffer (NPB) extracts.
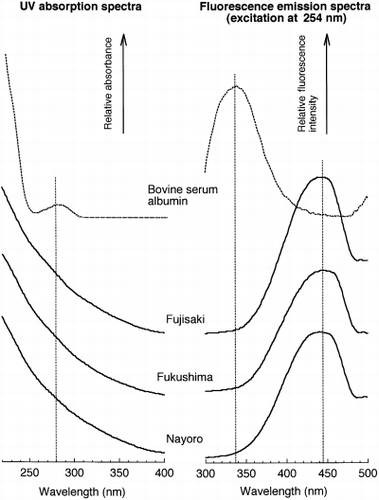
CitationMatsumoto et al. (2000) applied HPSEC with peak detection at 280 nm to soil NPB extracts and observed one major peak on the HPSEC chromatogram. They considered that the peak corresponded to the peak of a “protein-like substance” based on the fact that the intensity of the peak was related to the amount of CBRS in the NPB extract. CitationMatsumoto et al. (2000) suggested that the “protein-like substance” consisted of a complex of proteins and humic substances. However, measurements of the UV absorption spectrum and fluorescence emission spectrum indicated the absence of proteins in the substances responsible for the broad peak revealed by the HPSEC analysis of the soil NPB extracts.
Fractionation of NPB extracts
The amount of organic C extractable with NPB was largest in the Fukushima soil and smallest in the Fujisaki soil
Table 2 Contents of organic C, organic N and Coomassie Blue-reactive substances (CBRS) and their distribution among the fractions
Among the fractions, more than 60% of the organic C or N in the NPB extract was distributed in the PVP-non-adsorbed FA fraction, while less than 12% was found in the HA fraction. The predominance of the PVP-non-adsorbed FA fraction was also observed for the soil water extracts (CitationAoyama 2002). The C/N ratio was remarkably high in the PVP-adsorbed FA fraction compared with the other fractions. Almost half (42–54%) of the CBRS was distributed in the PVP-non-adsorbed FA fraction. However, the percentage distribution of CBRS in the PVP-non-adsorbed FA fraction was less than that of organic C or N. The CBRS-N/organic N was abnormally high in the PVP-adsorbed FA fraction and the HA fraction, exceeding 100% except for the HA fraction of the Fukushima soil.
All the fractions exhibited a broad peak upon HPSEC with UV detection at 280 nm (). The MW at peak maximum was highest for the HA fraction and lowest for the PVP-non-adsorbed FA fraction. On the chromatograms recorded at 210 nm and 400 nm, the broad peak was observed at the same elution volume as that monitored at 280 nm (data not shown). When monitored by fluorescence (excitation wavelength = 360 nm, emission wavelength = 450 nm), a broad peak was eluted later than that detected by UV absorption, as observed for the unfractionated NPB extracts (data not shown).
For all fractions, the broad peak showed a UV absorption spectrum with the absorbance decreasing as the wavelength increased (). The spectrum was similar to that of humic substances. When the fluorescence emission spectra (excitation at 254 nm) were measured for the broad peak, an emission maximum was located at 460 nm for the HA fraction and at 430–460 nm for both FA fractions. The fluorescence emission spectra of soil HAs showed two emission peaks at 460 nm and 510–530 nm (CitationAoyama 1999), the former corresponding to the peak observed for the HA fraction. In contrast, FAs showed an emission maximum at 430–460 nm, irrespective of their origin (CitationEwald et al. 1988; CitationMiano et al. 1988; CitationNagao et al. 1999; CitationSenesi et al. 1991), corresponding to the emission maxima observed for both FA fractions. These findings clearly indicated that the broad peaks observed on the chromatograms of the fractions were the peaks of humic substances.
The PVP-non-adsorbed FA fraction was the largest constituent of the organic matter in the NPB extract. CitationWatanabe and Kuwatsuka (1992) reported that the PVP-non-adsorbed fraction of soil FAs consisted mainly of non-humic substances, such as carbohydrates and peptides. Thus, the humic substances did not appear to be the main component of the organic matter in the PVP-non-adsorbed FA fraction. However, the peak observed by HPSEC with UV detection for the PVP-non-adsorbed FA fraction corresponded only to that of
Figure 3 Size exclusion chromatograms of the humic acid (HA), polyvinylpyrrolidone (PVP)-adsorbed fulvic acid (FA) and PVP-non-adsorbed FA fractions monitored by absorption at 280 nm. The molecular weights (MWs) indicated in the figure were estimated using polyethylene glycols as standards. AU, absorbance unit.
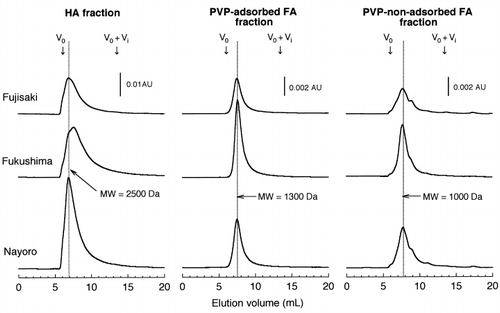
In the present study, CBRS were defined as the substances reactive with Coomassie Blue when a Bradford protein assay was conducted. This assay is based on the principle that Coomassie Blue reacts with aromatic and basic amino acids in proteins (CitationBradford 1976). Nevertheless, humic substances (CitationOgunseitan 1993) and phenols (CitationMattoo et al. 1987) also react with Coomassie Blue. Phenolic compounds are usually present in a relatively small amount in the soil solution (CitationGallet and Keller 1999). Hence, the fact that the presence of proteins was not confirmed by HPSEC suggested that the CBRS in the NPB extract consisted mainly of humic substances. Most of the organic matter in the HA and PVP-adsorbed FA fractions can be considered to consist of humic substances (CitationWatanabe and Kuwatsuka 1992). The assumption that CBRS are proteins would not be supported by the results showing that the amount of protein-N exceeded the amount of total organic N for the HA and PVP-adsorbed FA fractions of the NPB extracts. This result suggests that humic substances were the main constituents of the CBRS in the soil NPB extract.
Properties of bioavailable organic matter in the NPB extract
The amounts of organic C and N in the NPB extract from the Fujisaki soil decreased significantly during the 14-day period of incubation (). The NPB extract was turbid after incubation, and this turbidity could mainly be attributed to the formation of microbial cells. Thus, the organic matter in the NPB extract was not only mineralized, but also assimilated into the microbial cells.
The HA fraction disappeared and the amounts of organic C and N in the PVP-non-adsorbed FA fraction decreased significantly during the incubation (). In contrast, for the PVP-adsorbed FA fraction, the content of organic C remained constant and that of organic N increased slightly. The decrease in the content of organic matter in the PVP-non-adsorbed FA fraction can be attributed to mineralization and assimilation by microorganisms. In contrast, organic matter in the HA fraction was generally considered to be more refractory than that in the FA fraction (CitationTate 1987). Nevertheless, CitationBoyer and Groffman (1996) reported that in soil aqueous extracts, organic matter in the HA fraction was relatively more bioavailable than that in the FA fraction. Therefore, most of the organic matter in the HA fraction was likely to be mineralized or assimilated by microorganisms during the incubation period. However, the disappearance of organic matter in the HA fraction could, in part, result from precipitation or adsorption onto the microbial cells. Among the fractions, the decreases in the contents of organic C and N were highest in the PVP-non-adsorbed FA fraction (), indicating that the most bioavailable organic matter was present in the PVP-non-adsorbed FA fraction.
Figure 4 Ultraviolet (UV) absorption spectra and fluorescence emission spectra obtained for the broad peaks of the humic acid (HA), polyvinylpyrrolidone (PVP)-adsorbed fulvic acid (FA) and PVP-non-adsorbed FA fractions.
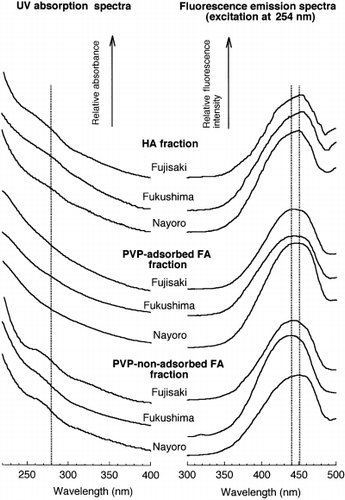
The HPSEC analysis of the NPB extracts before and after the incubation showed that the peak intensity of humic substances in the whole NPB extract decreased markedly during the incubation (). According to a Student's t-test, the decrease in the peak area was statistically significant (P = 0.001). This result strongly suggested that the humic substances in the NPB extract were available to microorganisms. Among the fractions, the HA fraction disappeared during the incubation. For the PVP-non-adsorbed FA fraction, a marked decrease in the peak intensity of humic substances was observed. The decrease in the peak area was statistically significant at P = 0.001. In contrast, no significant (P = 0.05) decrease in the peak area was observed for the PVP-adsorbed FA fraction. Hence, the humic substances in the HA and PVP-non-adsorbed FA fractions were more available to microorganisms than those in the PVP-adsorbed FA fraction.
Conclusions
The HPSEC analysis of the NPB extract revealed the presence of a single broad peak, irrespective of the
Figure 5 Amounts of organic C and N in the incubated (□) and unincubated (
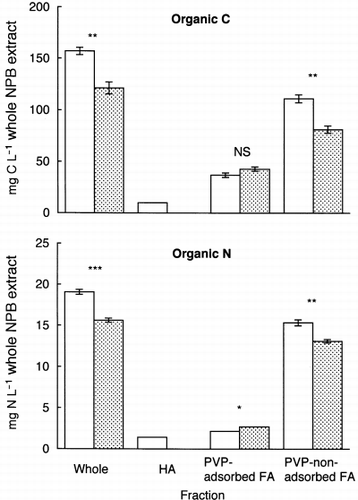
ACKNOWLEDGMENTS
We thank Dr S. Okano, National Agricultural Research Center for Tohoku Region, and Mr M. Onodera, Hokkaido Prefectural Central Agricultural Experiment Station, for providing the soil samples.
REFERENCES
- Higuchi , M . 1981 . Characterization of immobilized nitrogen and organic nitrogen extracted with various buffer solutions . JpnJSoil SciPlant Nutr , 52 : 481 – 489 . (in Japanese)
- Higuchi , M . 1982 . Characterization of soil organic nitrogen extracted with phosphate buffer . JpnJSoil SciPlant Nutr , 53 : 1 – 5 . (in Japanese)
- Matsumoto , S , Ae , N and Yamagata , M . 2000 . The status and origin of available nitrogen in soils . Soil SciPlant Nutr , 46 : 139 – 149 .
- Saito , M . 1988 . Estimation of nitrogen availability indices based on UV absorption of soil extracts . JpnJSoil SciPlant Nutr , 59 : 493 – 495 . (in Japanese)
- Murase , A , Yoneda , M , Ueno , R and Yonebayashi , K . 2003 . Isolation of extracellular protein from greenhouse soil . Soil BiolBiochem , 35 : 733 – 736 .
- Aoyama , M . 2002 . Characterization of water-soluble organic matter in soils by size exclusion chromatography and fractionation with polyvinylpyrrolidone . Soil SciPlant Nutr , 48 : 475 – 481 .
- Bradford , MM . 1976 . A rapid and sensitive method for the quantification of microgram quantities of protein utilizing the principle of protein-dye binding . AnalBiochem , 72 : 248 – 254 .
- Ogunseitan , OA . 1993 . Direct extraction of proteins from environmental samples . JMicrobiolMeth , 17 : 273 – 281 .
- Mattoo , RL , Ishaq , M and Saleemuddin , M . 1987 . Protein assay by Coomassie brilliant blue G-250 binding method is unsuitable for plant tissues rich in phenols and phenolases . AnalBiochem , 163 : 376 – 384 .
- Aoyama , M . 1996 . Use of high performance size exclusion chromatography to monitor the dynamics of water-soluble organic substances during the decomposition of plant residues in soil . Soil SciPlant Nutr , 42 : 21 – 30 .
- Berdén , M and Berggren , D . 1990 . Gel filtration chromatography of humic substances in soil solution using HPLC-determination of the molecular weight distribution . JSoil Sci , 41 : 61 – 72 .
- Ogawa , Y , Kato , H and Ishikawa , M . 1989 . A simple analytical method for index of soil nitrogen availability by extracting in phosphate buffer solution . JpnJSoil SciPlant Nutr , 60 : 160 – 163 . (in Japanese)
- Lowe , LE . 1980 . Humus fraction ratios as a means of discriminating between horizon types . CanJSoil Sci , 60 : 219 – 229 .
- Aoyama , M and Nagumo , T . 1996 . Factors affecting microbial biomass and dehydrogenase activity in apple orchard soils with heavy metal accumulation . Soil SciPlant Nutr , 42 : 821 – 831 .
- Mizushima , M , Tamase , K , Kitada , Y , Sasaki , M and Tanigawa , K . 1984 . Liquid chromatographic determination of ammonium in water . AnalChem , 56 : 603 – 605 .
- Artinger , R , Buckau , G , Geyer , S , Fritz , P , Wolf , M and Kim , JI . 2000 . Characterization of groundwater humic substances: influence of sedimentary organic carbon . ApplGeochem , 15 : 97 – 116 .
- O’Loughlin , E and Chin , Y-P . 2001 . Effect of detector wavelength on the determination of the molecular weight of humic substances by high-pressure size exclusion chromatography . Water Res , 35 : 333 – 338 .
- Schnitzer , M and Khan , SU . 1972 . Humic Substances in the Environment , New York : Marcel Dekker .
- Senesi , N , Miano , TM , Provenzano , MR and Brunetti , G . 1991 . Characterization, differentiation, and classification of humic substances by fluorescence spectroscopy . Soil Sci , 152 : 259 – 271 .
- Alberts , JJ and Takács , M . 2004 . Comparison of the natural fluorescence distribution among size fractions of terrestrial fulvic and humic acids and aquatic natural organic matter . Organic Geochem , 35 : 1141 – 1149 .
- Aoyama , M . 2001 . “ Do humic substances exhibit fluorescence? ” . In Understanding and Managing Organic Matter in Soils, Sediments, and Waters , Edited by: Swift , RS and Spark , KM . 125 – 131 . St. Paul : International Humic Substances Society .
- Buckau , G , Artinger , R , Kim , JI and Geyer , S . 2001 . “ Characterization of humic and fulvic acids from the Gorleben aquifer system by GPC with UV/Vis and fluorescence detection ” . In Understanding and Managing Organic Matter in Soils, Sediments, and Waters , Edited by: Swift , RS and Spark , KM . 399 – 405 . St. Paul : International Humic Substances Society .
- Miano , TM , Sposito , G and Martin , JP . 1988 . Fluorescent spectroscopy of humic substances . Soil SciSocAmJ , 52 : 1016 – 1019 .
- Nagao , S , Suzuki , Y , Nakaguchi , Y , Senoo , M and Hiraki , K . 1999 . Direct measurement of the fluorescence characteristics of aquatic humic substances by a three-dimensional fluorescence spectrophotometer . Bunseki Kagaku , 46 : 335 – 342 . (in Japanese with English summary)
- Ewald , M , Berger , P and Visser , SA . 1988 . UV-visible absorption and fluorescence properties of fulvic acids of microbial origin as functions of their molecular weight . Geoderma , 43 : 11 – 20 .
- Aoyama , M . 1999 . “ Chromatographic separation of fluorescent substances from humic acids ” . In Understanding Humic Substances: Advanced Methods, Properties and Applications , Edited by: Davies , G and Ghabbour , EA . 179 – 189 . Cambridge : The Royal Society of Chemistry .
- Watanabe , A and Kuwatsuka , S . 1992 . Chemical characteristics of soil fulvic acids fractionated using polyvinylpyrrolidone (PVP) . Soil SciPlant Nutr , 38 : 31 – 41 .
- Gallet , C and Keller , C . 1999 . Phenolic composition of soil solutions: comparative study of lysimeter and centrifuge waters . Soil BiolBiochem , 31 : 1151 – 1160 .
- Tate , RL III . 1987 . Soil Organic Matter: Biological and Ecological Effects , New York : Wiley .
- Boyer , JN and Groffman , PM . 1996 . Bioavailability of water extractable organic carbon fractions in forest and agricultural soil profiles . Soil BiolBiochem , 28 : 783 – 790 .