Abstract
A hydroponic experiment was conducted to observe the effect of arsenic (As) on a number of physiological and mineralogical properties of rice (Oryza sativa L. cv. Akihikari) seedlings. Seedlings were treated with 0, 6.7, 13.4 and 26.8 µmol L−1 As (0, 0.5, 1.0 and 2.0 mg As L−1) for 14 days in a greenhouse. Shoot dry matter yield decreased by 23, 56 and 64%; however, the values for roots were 15, 35 and 42% for the 6.7, 13.4 and 26.8 µmol L−1 As treatments, respectively. Shoot height decreased by 11, 35 and 43%, while that of the roots decreased by 6, 11 and 33%, respectively. These results indicated that the shoot was more sensitive to As than the root in rice. Leaf number and width of leaf blade also decreased with As toxicity. Arsenic toxicity induced chlorosis symptoms in the youngest leaves of rice seedlings by decreasing chlorophyll content. Concentrations and accumulations of K, Mg, Fe, Mn, Zn and Cu decreased significantly in shoots in the 26.8 µmol L−1 As treatment. However, the concentration of P increased in shoots at 6.7 and 13.4 µmol L−1 As levels, indicating a cooperative rather than antagonistic relationship. Arsenic and Fe concentration increased in roots at higher As treatments. Arsenic translocation (%) decreased in the 13.4 and 26.8 µmol L−1 As treatments compared with the 6.7 µmol L−1 As treatment. Arsenic and Fe were mostly concentrated in the roots of rice seedlings, assuming co-existence of these two elements. Roots contained an almost 8–16-fold higher As concentration than shoots in plants in the As treatments. Considering the concentration of Mn, Zn and Cu, it was suggested that chlorosis resulted from Fe deficiency induced by As and not heavy-metal-induced Fe deficiency.
INTRODUCTION
Arsenic (As) contamination in groundwater in Bangladesh was first discovered in 1993 by the Chemistry Division of the Atomic Energy Centre, Dhaka (AECD), at the village of Baroghari, Chapainawabganj District, Rajshahi Division (CitationAli 1995). The As-contaminated groundwater is the main source of drinking and home use for most village people of the country. It is also being used for agricultural purpose and this could be one of the major exposure routes to As toxicity in humans and animals. It was only in 1994 that the first groups of arsenicosis patients were found in Bangladesh; however, As contamination of groundwater had already been reported in 1978 in the state of West Bengal in India, the neighboring country on the western border of Bangladesh.
Around that time (1978), rice (Oryza sativa L.) growing during the dry season was started in Bangladesh under the “green revolution” to increase food production. Rice is the staple food in Bangladesh and covers approximately 75% of the total cropped area and 83% of the irrigated area (CitationDey et al. 1996). The average background concentration of As in Bangladesh is well below 10 mg kg−1 soil. However, in some areas where soils receive As-contaminated groundwater irrigation, the concentration has been found to be as high as 80 mg kg−1 soil (CitationHuq et al. 2003). The maximum As concentration in irrigation water was found to be 0.55 mg L−1. If a rice field is irrigated with this water when the water requirement is 1000 mm year−1, it is calculated that the As load will be 5 kg ha−1 year−1 (CitationHuq et al. 2003). Many crops receiving As-contaminated water as irrigation have been found to accumulate As at levels that exceed the minimum allowable daily limit (MADL) of 0.2 mg kg−1 dry weight (dw). Rice and wheat (Triticum aestivum L.) receiving As-contaminated irrigation water have been found to sequester the toxic metalloid into roots and stems (CitationHuq et al. 2003). However, the amount of As obtained through rice grains per person per day may, in many instances, surpass the MADL in Bangladesh.
More than 80% of the people in Bangladesh are involved in agriculture. Approximately 90% of men and 80% of women in rural areas of the country are engaged in agriculture (CitationBangladesh Bureau of Statistics 1998). Most groundwater used for irrigation in Bangladesh is contaminated with As (CitationKhan et al. 1998). If the groundwater contaminated with As is applied as irrigation water, it may reduce the growth as well as the production of the crops. CitationDavis and Coker (1979) reported that a 20% loss of cereal resulted from high concentrations of As (30 mg kg−1). Although the groundwater contained a high level of As, to date the source is obscure. There are a number of assumptions regarding the source of As in groundwater in Bangladesh. Deep tubewells were dug and a large volume of groundwater has been withdrawn for irrigation, particularly in the dry season. It is considered that withdrawal of groundwater may change the geochemical and physical changes of the underground, causing As contamination in turn. In general, groundwater contains 50% arsenate and 50% arsenite (CitationSamanta et al. 1999), which may convert from one form to another. Redox potential is generally governing this transformation (CitationMasscheleyn et al. 1991; CitationOnken and Hossner 1995). Arsenite is the dominant form in flooded paddy soil (CitationTakamatsu et al. 1982), which is considered to be the most toxic form. Arsenic toxicity is responsible for shorter plant height, weaker tillering, thinner leaf coloring, earlier root coloring to yellowish brown or brown, and curled leaves under sunlight in rice plants (CitationYamane 1989). Some data have already been published regarding plant responses at high As levels in, for example, rice (CitationAbedin et al. 2002), Chinese Brake fern (Pteris vittata L.) (CitationMa et al. 2001), bush bean (Phaseolus vulgaris L.) (CitationWallace et al. 1980), and tomato (Lycopersicum esculentum L.) (CitationCarbonell-Barrachina et al.1995). However, there is little data on the physiological response of rice under As toxicity. It is, therefore, necessary to observe the effect of As toxicity on the physiological and mineralogical properties of rice.
MATERIALS AND METHODS
Seed germination and plant culture
Rice (Oryza sativa L. cv. Akihikari) seeds were surface sterilized with 2% chlorinated lime [Ca(OCl)2] for 45 min and washed with tap water for 1 h. After washing, the seeds were wrapped between moistened towels and kept in a seed growth chamber at 25°C for 48 h. Then, the seeds were transferred on a net in a box containing 2% CaCl2 for 7 days and the seedlings were transferred to half-strength nutrient solution and grown for 14 days. Twenty-one days after germination, seedlings were hand-transplanted in groups of five in one bunch, and each bucket (10 L) contained 16 bunches. When the seedlings were suitable for transplantation (21 days after germination, at the fourth–fifth leaf stage of the seedlings), the treatments were started with full-strength solution containing 1 mmol L−1 NH4NO3, 1 mmol L−1 K2SO4, 0.8 mmol L−1 MgSO4, 0.5 mmol L−1 CaCl2, 0.5 mmol L−1 NaH2PO4, 10 µmol L−1 MnSO4, 1 µmol L−1 CuSO4, 1 µmol L−1 ZnSO4, 3 µmol L−1 H3BO3, 0.05 µmol L−1 H2MoO4 and 10 µmol L−1 Fe-citrate. The As treatments were 0, 6.7, 13.4 and 26.8 µmol L−1 (0, 0.5, 1.0 and 2.0 mg As L−1) and the duration of the As treatments was 14 days. Arsenic was added as sodium arsenite (NaAsO2). The pH (pH 5.5) was adjusted daily with a digital pH meter (Horiba Korea, Seoul, Korea) and with 1 mol L−1 HCl and/or 1 mol L−1 NaOH at approximately 16.00 hours during the experiment (August–September 2004). The solution was renewed every week and was not aerated.
SPAD value
The chlorophyll index (SPAD value) of fully developed (fifth leaf) new leaves was measured using a SPAD-502 chlorophyll meter (Minolta Camera Company, Tokyo Japan) 14 days after treatment. In each leaf, the SPAD values of three points were measured and the average was calculated. Means of each bunch were obtained. An average of the data of three bunches was calculated.
Analysis of plant samples
The rice seedlings were collected and washed with deionized water three times. Shoots and roots were separated and dried at 55°C for 48 h. The oven-dried samples were digested with a nitric acid–perchloric acid mixture (CitationPiper 1942). The amounts of K, Ca, Mg, Fe, Mn, Zn and Cu were determined by atomic absorption spectroscopy (CitationLoeppert and Inskeep 2001). Phosphorus was determined colorimetrically using an ultraviolet (UV)-visible spectrophotometer (model UV mini 1240, Shimadzu Corp., Kyoto Japan) at 420 nm wavelengths after developing the yellow color with vanadomolybdate as described by CitationBarton (1948) and CitationJackson (1958).
Determination of arsenic
Arsenic was measured using a hydride generation atomic absorption spectrophotometric (HGAAS) technique using a Hitachi HFS-3 instrument. We digested the samples with a nitric–perchloric acid mixture, and the volume of the solution was approximately 5 mL. After that, the volume of the digested solution was made up to 50 mL with MQ water. For As determination, the samples were further diluted up to 100–2000 times. As a result, the interference of nitrate on As determination could be minimized. Reduced nitrogen oxides (resulting from HNO3 digestion) and nitrite could suppress the instrumental response for As (CitationHuang and Fujii 2001).
Calculation of the parameters
Concentration in mg or µg of element g−1 dw; accumulation in shoot in mg or µg of element plant−1 shoot; accumulation in root in mg or µg of element plant−1 root; and translocation % in nutrient accumulation in shoot/total accumulation (shoot + root) × 100. CitationTu et al. (2004) defined the translocation factor (TF) as the ratio of the As concentration in fronds of Chinese Brake fern to that in the roots of the plant.
Reagents
All chemicals used were of analytical reagent grade. All solutions were prepared previously with pure water. The stock solution of As was prepared by dissolving NaAsO2 (Kanto Chemical Company, Tokyo, Japan) in pure water and was kept at room temperature.
Statistical analysis
The experiment was arranged in randomized blocks with three replications. Data on shoot and root dry matter yield, shoot height, root length, leaf number, leaf blade, SPAD value and mineral elements were subjected to anova. Differences between means were evaluated using a Ryan–Einot–Gabriel–Welsch multiple range test (P = 0.05) (CitationSAS 1988) using computer origin 5 at Iwate University, Morioka, Japan.
RESULTS AND DISCUSSION
Visible symptoms
Arsenic toxicity produced whitish chlorotic symptoms in the youngest leaf of rice seedlings after 14 days of treatment at 13.4 and 26.8 µmol L−1 As levels. At 13.4 µmol L−1 As treatment, the chlorotic symptoms were more pronounced than the other As treatments. We assumed that the chlorotic symptoms of rice induced by As were most probably Fe chlorosis because the symptoms were observed in the youngest leaves (CitationMengel and Kirkby 2001). If the chlorotic symptoms could be found in old leaves, it would be Mg chlorosis (CitationMaynard 1979). We found that the chlorophyll index was also lowest in the chlorotic leaves (). Not only chlorosis symptoms, but also necrosis (burning of leaf tip) was observed. In the early stage of As treatments, the leaves showed symptoms of curling at day time and the youngest leaves failed to unfold compared with control plants. This symptom may indicate water deficit on rice seedlings under As toxicity (CitationYamane 1989). The most common visible symptom was growth reduction, particularly in the shoot. A reddish color along the root length was found and felt slippery to touch because of As toxicity. Reduction of root length because of As toxicity could be termed “little root”. The depth of reddish color was greater with increasing As supply. The reddish color of the roots may result from the
Figure 1 (a) Leaf number, (b) width of leaf blade and (c) SPAD value of fully developed young leaves (fifth) of rice seedlings with different levels of As. Bars with different letters are significantly different (P < 0.05) according to a Ryan–Einot–Gabriel–Welsch multiple range test.
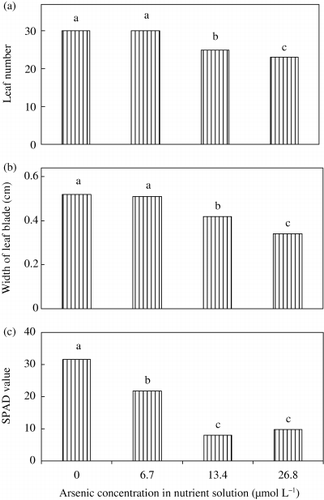
Dry matter yield
It was clearly indicated that dry matter yield in shoot and root decreased significantly with As treatments in the nutrient solution (). In the presence of As, the activity of enzyme or protein or plant growth regulators may be decreased, decreasing plant growth. This speculation needs verification. Dry matter yield decrease may also be associated with the reduction of leaf number, width of leaf blade; and shoot height and root length (,). Shoot dry matter yield decreased by 23, 56 and 64%, while the values of the roots were 15, 35 and 42% for the 6.7, 13.4 and 26.8 µmol L−1 As treatments, respectively. This result indicated that As caused greater reduction of shoot dry matter yield than that of the root. Our result suggested that the threshold value of As sensitivity in hydroponic culture was between 0 and 6.7 µmol L−1 As (0.5 mg As L−1), considering that elemental concentration inducing > 10% reduction of dry matter yield is a critical toxic level (CitationOhki 1984). CitationAbedin et al. (2002) found considerable reduction in straw and root biomass of rice with an 8 mg As L−1 concentration in a greenhouse pot experiment.
Shoot height and root length
Shoot height and root length decreased significantly in the As treatments (). Shoot height decreased by 11, 35 and 43%, while the values for root length were 6, 11 and 33% for 6.7, 13.4 and 26.8 µmol L−1 As treatments, respectively. This result indicated that shoot
Figure 2 (a) Dry matter yield and (b) shoot height and root length of rice seedlings with different levels of As. Bars with different letters are significantly different (P < 0.05) according to a Ryan–Einot–Gabriel–Welsch multiple range test.
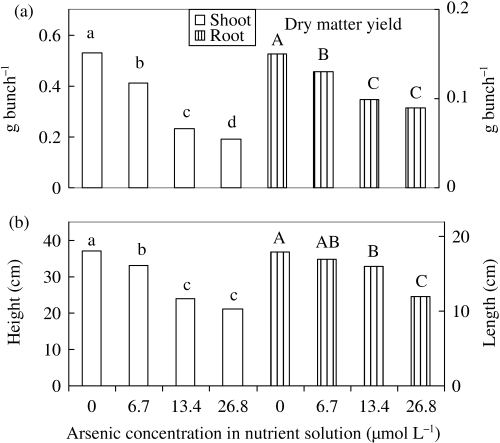
Tiller number, leaf number and leaf blade
During the duration of this experiment (35 days), we did not find any new tiller even in control plants. However, leaf number and width of leaf blade decreased significantly in the 13.4 and 26.8 µM As treatments (), and these parameters were considered to be responsible for the reduction in dry matter yield. CitationAbedin et al. (2002) found lower numbers of tiller of rice in the 8 mg As L−1 treatment in their greenhouse pot experiment. CitationMarin et al. (1993) reported that rice leaf area decreased in 0.8 and 1.6 mg As (dimethylarsinic acid, DMAA) L−1 treatments.
SPAD value (chlorophyll index)
Chlorophyll content in the fully developed youngest leaf of rice seedlings decreased significantly with As treatment (). The lowest value was recorded in the 13.4 µmol L−1 As treatment. We found that the concentration of Fe decreased with As treatments and the lowest value was recorded in the 13.4 µmol L−1 As treatment (). It is well known that Fe is essential for the formation of chlorophyll. In this experiment, Fe concentration decreased; therefore, the reduction in Fe concentration could be highly responsible for the reduction in chlorophyll content. It is our belief that we are presenting chlorophyll data in As toxic rice plants for the first time. It was considered that As-induced chlorosis resulted from Fe-deficiency because the chlorosis was found in the youngest leaves (CitationMengel and Kirkby 2001).
Arsenic concentration, accumulation and translocation
Arsenic concentration increased both in shoot and root as a result of the applied As in the nutrient solution (). Arsenic concentration in the roots was 8, 16 10-fold greater than the concentration in the shoots at 6.7, 13.4 and 26.8 µmol L−1 As treatments, respectively, indicating that As was mostly concentrated in the root.
Figure 3 Effect of As on the concentration of (a) Fe, (b) Mn, (c) Zn and (d) Cu in shoots and roots of rice seedlings. Bars with different letters are significantly different (P < 0.05) according to a Ryan–Einot–Gabriel–Welsch multiple range test.
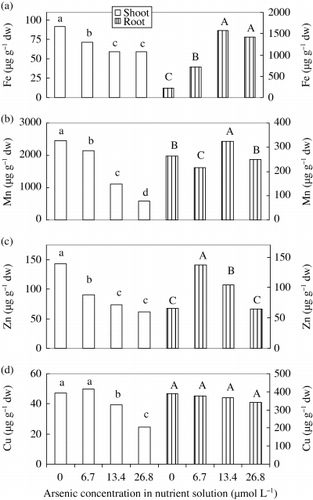
Figure 4 Effect of As on the (a) concentration and (b) accumulation of As in shoots and roots of rice seedlings. Bars with different letters are significantly different (P < 0.05) according to a Ryan–Einot–Gabriel–Welsch multiple range test.
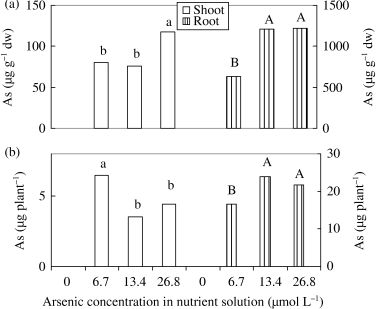
In a previous experiment, CitationWallace et al. (1980) could not detect As in root, stem and leaves of bush bean plants in 0, 10−6 and 10−5 mol L−1 H2AsO− 4 treatments in the nutrient solution, but observed 41.7, 18.8 and 3.6 µg As g−1 dw in 10−4 mol L−1 H2AsO− 4 treatment for root, stem and leaves, respectively. It was also reported that fronds contained an almost 24-fold greater As concentration than the roots in Brake fern (CitationMa et al. 2001). In our experiment, As translocation decreased in 13.4 and 26.8 µmol L−1 As treatments compared to the 6.7 µmol L−1 As treatment (). This suggested that the toxicity was very severe and reduced translocation
Figure 5 Effect of As on the translocation (%) of As from roots to shoots of rice seedlings. Bars with different letters are significantly different (P < 0.05) according to a Ryan–Einot–Gabriel–Welsch multiple range test.
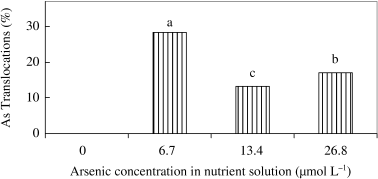
Phosphorus concentration, accumulation and translocation
We observed that the concentration of P increased in shoots significantly in the 6.7 and 13.4 µmol L−1 As treatments and marginally in the 26.8 µmol L−1 As treatment () compared to the control plants, which might be a concentration effect because of dry matter yield () and the accumulation of P () decreased. CitationMarin et al. (1993) found that P concentration increased marginally in shoot and root of rice seedlings in 0.8 and 1.6 mg As (DMAA) L−1 treatments. It has been well documented that arsenate is taken up by the phosphate uptake system in plants (CitationAsher and Reay 1979; CitationMeharg and Macnair 1990). We found that the accumulation of P in shoots and roots decreased significantly (), but that translocation (%) increased () with As toxicity. The antagonistic relationship is probably dependant on the concentration of P, the species of plant and the composition of the rooting medium. The relationship of the mechanism for P and As needs to be investigated.
Potassium concentration, accumulation and translocation
Concentration of K decreased significantly in shoots in the 26.8 µmol L−1 As treatment in the nutrient solution; but K concentration in roots decreased according to the increase of As concentration, indicating an antagonistic relationship between K and As (). Accumulation was also negatively influenced by As (), but translocation (%) was not markedly affected ().
Figure 6 Effect of As on the concentration of (a) P, (b) K, (c) Ca and (d) Mg in shoots and roots of rice seedlings. Bars with different letters are significantly different (P < 0.05) according to a Ryan–Einot–Gabriel–Welsch multiple range test.
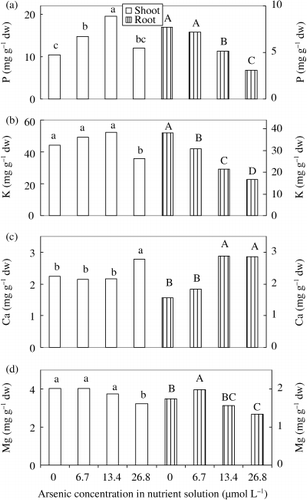
Calcium concentration, accumulation and translocation
In shoots, the concentration of Ca was constant in the range between 0 and 13.4 µmol L−1 As, but increased significantly in the 26.8 µmol L−1 As treatment. Similar results were also found in roots in the 13.4 and
Table 1 Accumulation of nutrients in shoots and roots of rice seedlings grown in nutrient solution with different levels of As
Table 2 Translocation (%) of elements from roots to shoots in rice seedlings grown in nutrient solution with different levels of As
Magnesium concentration, accumulation and translocation
It was clearly observed that the concentration of Mg in shoots was statistically constant in the range between 0 and 13.4 µmol L−1 As; however, it decreased significantly in the 26.8 µmol L−1 As treatment (). A similar result was also obtained for roots at the highest As treatment. This may be the toxic effect of As on the nutritional status of Mg in the plant. Accumulation of Mg in the shoots was also negatively influenced by the increase in As (). We observed that the translocation (%) of Mg was also negatively influenced by As toxicity (). It has been reported that Mg concentration in shoots increased in 1.6 mg As (DMAA) L−1 treatment, but there was no change in rice roots (CitationMarin et al. 1993). This dissimilarity may result from varietal differences in rice plants and/or the form of As. CitationWallace et al. (1980) reported that Mg concentration increased in leaves and stems in 10−4 mol L−1 H2AsO− 4 treatment, but decreased in roots at the same condition in bush bean plants. CitationCarbonell-Barrachina et al. (1997) reported that Mg concentration in leaves decreased in 2 and 5 mg As L−1 treatments at the first fruiting stage, but increased at the harvesting stage.
Iron concentration, accumulation and translocation
Concentration of Fe was negatively influenced by As in shoots, but the opposite results were obtained for roots (). In shoots, the highest concentration was recorded in control plants and the lowest in the 13.4 and 26.8 µmol L−1 As treatments. The highest value was for 13.4 and 26.8 µmol L−1 As treatments in roots. These results indicated that As concentrated Fe in roots and blocked Fe translocation from roots to shoots, which was supported by . It was found that the translocation (%) of Fe was mostly reduced by As among the metal micronutrients (). The obtained data of our water culture experiment suggested that Fe might be inactivated by As in or on the surface of roots. By using an X-ray micro analyzer, CitationYamane (1989) suggested that Fe and As accumulated on the surface of rice roots. The author also discussed that Fe might be precipitated with As in the rhizosphere; therefore, application of Fe3+ or Fe2+ might alleviate As toxicity in the soil. CitationMarin et al. (1993) found that the concentration of Fe remained constant statistically in shoots but increased in the roots of rice plants in 1.6 mg As (DMAA) L−1 treatment. However, it has also been reported that Fe concentration increased in leaves, stems and roots of bush bean plants (CitationWallace et al. 1980). It is well established that Mg chlorosis is found first in old leaves (CitationMaynard 1979), but that the Fe chlorosis is found in young leaves (CitationMengel and Kirkby 2001). In our experiment, the chlorotic symptoms were observed in the youngest leaf, indicating Fe chlorosis induced by As toxicity. Generally, leaves containing 30–50 µg Fe g−1 dw are considered to be Fe-deficient leaves (CitationBergmann 1988). We found that plants treated with As contained Fe concentrations slightly higher than the deficient level, this may be because we digested the old leaf together with the new leaf and determined the Fe concentration.
Manganese concentration, accumulation and translocation
The effect of As toxicity on Mn concentration was more pronounced in shoots than roots. Manganese concentration decreased significantly with increasing As concentration and the lowest value was obtained for the highest As concentration (). CitationMarin et al. (1993) also found a similar result in roots of rice seedlings, although the differences were not significant. However, CitationYamane (1989) reported that the concentration of Mn increased both in shoots and roots of rice with the application of As3+ or As5+ at a rate of 33.5, 67 and 134 mg kg−1 of soil. Manganese concentration decreased in leaves and roots of bush bean plant in 10−4 mol L−1 H2AsO− 4 treatment, but increased in stems in the same treatment (CitationWallace et al. 1980). It was suggested that As did not induce Mn toxicity in our experiment. It is known that Mn toxicity induces Fe deficiency (CitationAlam et al. 2000). Shoots of rice containing 9,181 µg g−1 dw showed symptoms of Mn toxicity (CitationAlam et al. 2003). We found that accumulation () and translocation (%) of Mn () decreased significantly in the highest As treatment. In contrast, the critical deficient level of Mn in plants was similar; varying between 10–20 mg Mn kg−1 dw in fully expanded leaves (CitationMarschner 1998). The values of this experiment were much higher than the critical deficient level of Mn. The plants treated with 13.4 and 26.8 µmol L−1 As were not Mn deficient or suffering from Mn toxicity. Therefore, it was considered that Mn toxicity was not related to the induction of whitish chlorosis symptoms observed in the youngest leaves of the rice.
Zinc concentration, accumulation and translocation
Concentration of Zn decreased in shoots treated with As, but increased in 6.7 and 13.4 µmol L−1 As treatments in roots () compared with the control treatment. Not only the concentration, but also the accumulation, of Zn decreased at the highest As treatment. It is well known that P can induce Zn deficiency (CitationMarschner and Schropp 1977) and As could compete with P (CitationAsher and Reay 1979). The facts may explain the result that high quantities of As may reduce Zn concentration. In leaves, the critical deficiency levels are below 15–20 µg Zn g−1 dw. CitationMarin et al. (1993) found that the Zn concentration increased in shoots of rice in 1.6 mg As (DMAA) L−1 treatment but decreased in roots in 0.2 mg As (DMAA) L−1 treatment. It has been shown that Zn toxicity leads to chlorosis in young leaves (CitationMarschner 1998). The critical toxicity level of crop plants is between 100 and more than 300 µg Zn g−1 dw (CitationRuano et al. 1988), with the latter values more typical (CitationMarschner 1998). Our data was within the normal level (from 144 [in control plants] to 60 µg Zn g−1 dw [in 26.8 µmol L−1 As treated plants]). Based on our results, it was considered that Zn toxicity was not involved in the production of chlorotic symptoms in the leaves in this experiment.
Copper concentration, accumulation and translocation
Copper concentration in shoots decreased with increasing As. However, the concentration of Cu in the roots was constant (), indicating that As hindered the translocation of Cu from roots to shoots. Accumulation and translocation (%) of Cu decreased with As toxicity (,). This was considered to be a toxic effect of As. It has been reported that Cu concentration increased in roots of rice in 1.6 mg As L−1 treatment (CitationMarin et al. 1993). Our experimental rice plants contained Cu concentrations (24.4–50.0 µg g−1 dw) over the critical toxic level. For most crop species, the critical toxicity level of Cu in the leaves is above 20–30 µg g−1 dw (CitationRobson and Reuter 1981). However, marked differences in Cu tolerance among plant species may also be possible. In certain Cu-tolerant species (metallophytes) of the natural vegetation, the Cu content in leaves can be as high as 1,000 µg g−1 dw (CitationMorrison et al. 1981). Considering the fact that the Cu concentration in shoots decreased with increasing As, Cu toxicity may not be involved in the production of chlorosis symptoms in leaves.
Conclusion
Arsenic toxicity decreased the dry matter yield, shoot height, root length, leaf number and leaf blade. Shoots were more sensitive to As than roots. The critical toxic level for As in this rice cultivar in hydroponic culture may be between 0 to 6.7 µmol L−1 As (0–0.5 mg L−1). Arsenic induced chlorotic symptoms in the youngest leaves. In the plants treated with higher As concentrations, Fe, Mn, Zn and Cu concentrations in shoots were reduced compared with control plants. Therefore, it was suggested that the chlorosis was most probably Fe chlorosis caused by Fe deficiency induced by As, and was not the symptom of heavy-metal-induced Fe deficiency. This chlorosis symptom may be caused by a Fe-translocation problem because Fe-translocation was most affected among the nutrient elements. Arsenic and Fe were mostly concentrated in or at the surface of the rice roots. Our experimental results suggested that Fe may be inactivated by As in or at the surface of the roots.
ACKNOWLEDGMENTS
The authors wish to express their appreciation to the Ministry of Education, Science, Culture and Sports, Government of Japan, for financial support. We are also grateful to Mr Shigeru Kamei, Dr Shah Alam, Mr Ryushi Kodama, Mrs Mayumi Takahashi, Mr Ladouceur Antoine, Mr Kazuaki Kudo and Mr Koji Uejo for their kind assistance during the research.
REFERENCES
- “ AliM1995 X-ray analytical studies of trace elements in food and drink: A nutritional assessment in Bangladesh ” . Dhaka : Jahangirnagar University . (PhD thesis)
- Evenson , RE , Herdt , RW and Hossain , M , eds. “ DeyMM MiahMNI MustafiBAA HossainM1996Rice production constraints in Bangladesh: Implications for further research priorities ” . In Rice Research in Asia: Progress and Priorities , 179 – 191 . Manila, , Philippines : CAB International, Wallingford, UK and International Rice Research Institute .
- 2003 . HuqSMI AlamS KawaiS2003 Arsenic in Bangladesh environment and its impact on food chain through crop transfer . Abstracts of the Annual Meeting Japanese Society of Soil Science and Plant Nutrition . August 20–22 2003 , Tokyo. Vol. 49 , Abstract No. 22–25
- Bangladesh Bureau of Statistics . 1998 . Statistical Yearbook of Bangladesh , Bangladesh Bureau of Statistics, Statistics Division, Ministry of Planning Peoples Republic of Bangladesh .
- 1998 . KhanAW AhmedSA SayedMHSUet al.1998 Arsenic contamination in ground water and its effect on human health with particular reference to Bangladesh . Presented at the International Conference on Arsenic Pollution of Groundwater in Bangladesh . February 8–12 1998 .
- DavisRD CokerEG1979 Proceedings of the International Conference on the Management and Control of Heavy Metals in the Environment 553 CEP Consultants LtdEdinburgh
- Samanta , GTR , Chowdhury , BK Mandal , BK . 1999 . Flow injection hydride generation atomic absorption spectrometry for determination of arsenic in water and biological samples from arsenic-affected districts of West Bengal, India and Bangladesh . MicrochemJ , 62 : 174 – 191 .
- Masscheleyn , PH , Delaune , RD and Patrick , WH Jr . 1991 . “ Effect of redox potential and pH on arsenic speciation and solubility in a contaminated soil ” . In EnvironSciTechnol Vol. 25 , 1414 – 1419 .
- Onken , BM and Hossner , LR . 1995 . Plant uptake and determination of arsenic species in soil solution under flooded conditions . JEnvironQual , 24 : 373 – 381 .
- Takamatsu , T , Aoki , H and Yoshida , T . 1982 . Determination of arsenate, arsenite, monomethylarsonate, and dimethylarsinate in soil polluted with arsenic . Soil Sci , 133 : 239 – 246 .
- Yamane , T . 1989 . The mechanisms and countermeasures of arsenic toxicity to rice plant . BullShimane AgricExpStat , 24 : 1 – 95 . (in Japanese with English summary)
- Ma , LQ , Komar , KM , Tu , C , Zhang , W , Cai , Y and Kennelley , ED . 2001 . A fern that hyperaccumulates arsenic . Nature , 409 : 579
- Wallace , A , Mueller , RT and Wood , RA . 1980 . Arsenic phytotoxicity and interactions in bush bean plants grown in solution culture . JPlant Nutr , 2 : 111 – 113 .
- Carbonell-Barrachina , A , Burló-Carbonell , F and Mataix-Beneyto , J . 1995 . Arsenic uptake, distribution and accumulation in tomato plants: Effect of arsenite on plant growth and yield . JPlant Nutr , 18 : 1237 – 1250 .
- Piper , CS . 1942 . Soil and Plant Analysis , Adelaide : Hassell Press .
- Loeppert , RH and Inskeep , WP . 2001 . Iron In Methods of Soil Analysis, Part 3-Chemical Methods , SSSA Book Series 5 Edited by: Sparks , DL . 639 – 664 . Madison : Soil Science Society of America and American Society of Agronomy .
- Barton , CJ . 1948 . Photometric analysis of phosphate rock . AnalChem , 20 : 1068 – 1073 .
- Jackson , ML . 1958 . “ Total elemental phosphorus ” . In Soil Chemical Analysis , Edited by: Jackson , ML . 175 – 176 . Englewood Cliffs : Prentice-Hall .
- Huang , PM and Fujii , R . 2001 . “ Selenium and Arsenic ” . In Methods of Soil Analysis, Part 3-Chemical Methods , SSSA Book Series 5 Edited by: Sparks , DL . 793 – 831 . Madison : Soil Science Society of America and American Society of Agronomy .
- Tu , S , Ma , L and Luongo , T . 2004 . Root exudates and arsenic accumulation in arsenic hyperaccumulating Pteris vittataand non-hyperaccumulating Nephrolepis exaltata . Plant Soil , 258 : 9 – 19 .
- SAS . 1988 . SAS/STAT User's guide, No1, ANOVA, Version 6 , 4th edn , Cary : Statistical Analysis System Institute .
- Mengel , K and Kirkby , EA . 2001 . “ Iron ” . In In Principles of Plant Nutrition , 5th edn , Edited by: Mengel , K and Kirkby , EA . 553 – 571 . Dordrecht, , The Netherlands : Kluwer Academic Publishers .
- Maynard , DN . 1979 . “ Nutritional disorders of vegetable crops. A review ” . In JPlant Nutr Vol. 1 , 1 – 23 .
- Batty , LC and Younger , PL . 2003 . Effects of external iron concentration upon seedling growth and uptake of Fe and phosphate by the common reed, Phragmites australis(Cav.) Trin ex. Steudel . AnnBot , 92 : 801 – 806 .
- Ohki , K . 1984 . “ Zinc nutrition related to critical deficiency and toxicity levels for sorghum ” . In AgronJ Vol. 76 , 253 – 256 .
- Abedin , MJ , Cotter-Howells , J and Meharg , AA . 2002 . Arsenic uptake and accumulation in rice (Oryza sativaL.) irrigated with contaminated water . Plant Soil , 240 : 311 – 319 .
- Speer , HL . 1973 . The effect of arsenate and other inhibitors on early events during the germination of lettuce seeds (Lactuca sativaL.) . Plant Physiol , 52 : 129 – 133 .
- Isensee , AR , Jones , GE and Turner , BC . 1971 . Root absorption and translocation of picloram by oats and soybeans . Weed Sci , 19 : 727 – 731 .
- Orwick , PL , Schrieber , MM and Hodges , TK . 1976 . Adsorption and efflux of chloro-s-triazines by Setaria roots . Weed Res , 16 : 139 – 144 .
- Abedin , MJ and Meharg , AA . 2002 . Relative toxicity of arsenite and arsenate on germination and early seedling growth of rice (Oryza sativaL.) . Plant Soil , 243 : 57 – 66 .
- Tsutsumi , M . 1980 . Intensification of arsenic toxicity to paddy rice by hydrogen sulphide and ferrous iron I. Induction of bronzing and accumulation in rice by arsenic . Soil SciPlant Nutr , 26 : 561 – 569 .
- Marin , AR , Pezeski , SR , Masscheleyn , PH and Choi , HS . 1993 . Effect of dimethylarsenic acid (DMAA) on growth, tissue arsenic, and photosynthesis of rice plants . JPlant Nutr , 16 : 865 – 880 .
- Wauchope , RD . 1983 . “ Uptake, translocation and phytotoxicity of arsenic in plants ” . In Arsenic: Industrial, Biomedical, Environmental Perspectives, Arsenic Symposium , Edited by: Lederer , WH and Fensterheim , RJ . 348 – 374 . New York : Gaithersburg, MD. Van Nostrand Reinhold Company .
- Armstrong , W . 1967 . The oxidising activity of roots in waterlogged soils . PhysiolPlant , 20 : 920 – 926 .
- Chen , CC , Dixon , JB and Turner , FT . 1980 . Iron coatings on rice roots: Morphology and models of development . Soil SciSocAmJ , 44 : 1113 – 1119 .
- Otte , ML , Dekkers , ML , Rozema , J and Broekman , RA . 1991 . Uptake of arsenic by Aster tripoliumin relation to rhizosphere oxidation . CanJBot , 69 : 2670 – 2677 .
- Liu , WJ , Zhu , YG and Smith , FA . 2005 . Effects of iron and manganese plaques on arsenic uptake by rice seedlings (Oryza sativaL.) grown in solution culture supplied with arsenate and arsenite . Plant Soil , 277 : 127 – 138 .
- Meharg , AA and Jardine , L . 2003 . Arsenic transport into paddy rice (Oryza sativa) roots . New Phytol , 157 : 39 – 44 .
- Batty , LC , Baker , AJM , Wheeler , BD and Curtis , CD . 2000 . The effect of pH and plaque on the uptake of Cu and Mn in Phragmites australis(Cav.) Trin ex Steudel . AnnBot , 86 : 647 – 653 .
- Chen , Z , Zhu , YG , Liu , WJ and Meharg , AA . 2005 . Direct evidence showing the effect of root surface iron plaque on arsenite and arsenate uptake into rice (Oryza sativaL.) roots . New Phytol , 165 : 91 – 97 .
- Sachs , RM and Michael , JL . 1971 . Comparative phytotoxicity among four arsenical herbicides . Weed Sci , 19 : 558 – 564 .
- Asher , CJ and Reay , PF . 1979 . Arsenic uptake by barley seedlings . AustJPlant Physiol , 6 : 459 – 466 .
- Meharg , AA and Macnair , MR . 1990 . An altered phosphate uptake system in arsenate-tolerant Holcus lanatusL . New Phytol , 116 : 29 – 35 .
- Carbonell-Barrachina , AA , Burló-Carbonell , F and Mataix-Beneyto , J . 1997 . Effect of sodium arsenite and sodium chloride on bean plant nutrition (Macronutrients) . JPlant Nutr , 20 : 1617 – 1633 .
- Bergmann , W . 1988 . Ernährungsstörungen bei KulturpflanzenEntstehung, visuelle und analytische Diagnose , Jena : Gustav Fischer Verlag .
- Alam , S , Kamei , S and Kawai , S . 2000 . Phytosiderophore release from manganese-induced iron deficiency in barley . JPlant Nutr , 23 : 1193 – 1207 .
- Alam , S , Kamei , S and Kawai , S . 2003 . Amelioration of manganese toxicity in young rice seedlings with potassium . JPlant Nutr , 26 : 1301 – 1314 .
- Marschner , H . 1998 . “ Functions of mineral nutrients: Micronutrients ” . In Mineral Nutrition of Higher Plants , Edited by: Marschner , H . 313 – 404 . London : Academic Press .
- Marschner , H and Schropp , A . 1977 . Comparative studies on the sensitivity of six rootstock varieties of grapevine to phosphate-induced Zn deficiency . Vitis , 16 : 79 – 88 .
- Ruano , A , Poschenrieder , Ch and Barceló , J . 1988 . Growth and biomass partitioning in zinc-toxic bush beans . JPlant Nutr , 11 : 577 – 588 .
- Robson , AD and Reuter , DJ . 1981 . “ Diagnosis of copper deficiency and toxicity ” . In Copper in Soils and Plants , Edited by: Loneragan , JF , Robson , AD and Graham , RD . 287 – 312 . London : Academic Press .
- Morrison , RS , Brooks , RD Reeves , RD . 1981 . The diverse chemical forms of heavy-metals in tissue extracts of some metallophytes from Shaba province, Zaire . Phytochem , 20 : 455 – 458 .