Abstract
Athyrium yokoscense, a type of fern that grows vigorously in mining areas in Japan, is well known as a Cd hyperaccumulator as well as a Cu, Pb and Zn tolerant plant. However, no information is available on As accumulation of A. yokoscense, although it often grows on soils containing high levels of both heavy metals and As. In this study, young ferns collected from a mine area were grown in media containing As-spiked soils or mine soil in a greenhouse for 21 weeks. Athyrium yokosense was highly tolerant to arsenate and survived in soils containing up to 500 mg As (V) kg−1. The addition of 100 mg As (V) kg−1 resulted in the highest fern biomass (1.95 g plant−1) among As-spiked soils. Although the As concentration of the fern was lower than other As hyperaccumulators, such as Pteris vittata, A. yokoscense could hyperaccumulate As in mature and old fronds. Arsenic was accumulated most efficiently in old fronds (922 mg kg−1) in the media containing 5 mg As (III) kg−1. Moreover, higher As accumulation was found in the roots of the ferns, with a range from 506 to 2,192 mg kg−1. In addition, in the mine soil with elevated concentrations of As and heavy metals, A. yokoscense not only hyperaccumulated As (242 mg As kg−1 in old fronds), but also accumulated Cd, Pb, Cu and Zn at concentrations much higher than those reported for other terrestrial plants. Athyrium yokoscense accumulated Cd mostly in fronds in high concentrations, up to 1095 mg kg−1, while it accumulated Cu, Zn and Pb mainly in the roots and the concentrations were 375, 2040 and 1165 mg kg−1, respectively.
INTRODUCTION
Arsenic and heavy metal contamination in soils has become an environmental concern worldwide. In the environment, metal contaminated soils are difficult to remediate and can adversely impact human health. Even in low doses, some metals still pose a major threat. Therefore, cost-efficient and environmentally friendly remediation techniques are urgently required. Phytoremediation can be defined as the combined use of plants, soil amendments and agronomic practices to remove pollutants from the environment or to decrease their toxicity (CitationChaney et al. 1999; CitationCunningham et al. 1995; CitationWard and Singh 2004). This technique has many advantages compared with other remediation procedures: low economic costs, the generation of recyclable residues and the possibility of being applied to soils and water with minimum environmental impact (CitationCunningham et al. 1995; CitationTu and Ma 2002; CitationWard and Singh 2004). Phytoextraction techniques involve the elimination of the pollutants from the soil by accumulating the toxic elements in the harvestable parts of the plants (CitationCaille et al. 2004; CitationFrancesconi et al. 2002; CitationLombi et al. 2001; CitationTu et al. 2002). Finding the optimum plant species for remediation of a given soil will be the most important point controlling the success of the process, as well as the selection of adequate soil amendments that allow plant survival and good growth.
A plant that takes up abnormally large quantities of contaminants from soils and sequesters them in its aboveground biomass is known as a hyperaccumulator (CitationAdriano 2001; CitationTu et al. 2002). More precisely, a Cd and As hyperaccumulator is defined as a plant that accumulates more than 100 mg kg−1 dry weight (DW), whereas a Co, Cu, Cr and Pb hyperaccumulator is defined as a plant that contains more than 1,000 mg kg−1 DW (CitationBaker and Brooks 1989). In general, the concentrations of metals in hyperaccumulator plants are approximately 100 to 1,000-fold higher than those in normal plants growing on soils with background metal concentrations, and approximately 10 to 100-fold higher than most other plants growing on metal-contaminated soils (CitationMcGrath et al. 2002). There are more than 400 plant species known to hyperaccumulate heavy metals, including Arabis gemmifera (CitationKubota and Takenaka 2003), Thlaspi caerulescens (CitationLombi et al. 2000), Berkheya coddii (CitationRobison et al. 2003), Sonchus asper L., and Corydalis pterygopetala F (CitationZu et al. 2005). Only recently, some types of ferns such as Pteris vittata (CitationMa et al. 2001), Pityrogramma calomelanos L (CitationFrancesconi et al. 2002), Pteris cretica, Pteris longifolia and Pteris umbrosa (CitationZhao et al., 2002) have been reported to be hyperaccumulators of As. However, the applicability of these plants to sites with multiple mixed contaminants is limited because these hyperaccumulators often accumulate a specific element only (CitationMcIntyre 2003). At many hazardous waste sites, where cleanup is required, the contaminated soil, groundwater and wastewater contain a mixture of several contaminants at widely varying concentrations. Especially in Japan, heavy metal contaminated soils often coincide with high levels of As in the soil (CitationTsutsumi 1981). The simultaneous cleanup of multiple contaminants using conventional methods such as landfills, soil washing, acid leaching or vapor stripping are all technically difficult and expensive; and these methods also destroy the biotic component of the soils (CitationFrancesconi et al., 2002; CitationTu and Ma, 2002). Therefore, it is desirable to find plant species that can accumulate multiple contaminated elements concurrently.
Athyrium yokoscense (Aspideaceae), a type of ferns, is distributed throughout Japan, particularly in the mine areas with metalliferous soil and is also distributed in Korea, Sakhanlin, northeastern China and eastern Siberia (CitationHiroi 1981). It is an indicator plant of heavy metals and grows vigorously in mining areas. It grows thickly on unstable slag heaps, flat damp grounds and floodplains. Through a preliminary survey of soil and vegetation around a closed old silver mine in Hyogo Prefecture, Japan, we have found that this fern propagates on soils containing high levels of As together with heavy metals (CitationKang et al. 2005). This fern has been reported to be a Cd and Pb hyperaccumulator. Morishita and Boratynski (1992); indicated that the leaflets (pinnule) of A. yokoscense could accumulate 996 mg kg−1 of Cd. CitationYoshihara et al. (2005) also reported that the tissues of this fern accumulated high levels of Cd (maximum 3.3 mg g−1 dry weight in roots). In addition, results of the studies on Pb accumulation showed that Pb concentration of this fern was more than 10 000 mg kg−1 in gametophytes (CitationKamachi et al. 2005) and more than 2000 mg kg−1 in the leaf blade (CitationSakai et al. 1991) as well as a Cu and Zn tolerant plant. Not only shows hyperaccumulation of Cd and Pb, A. yokoscense also reported as Cu and Zn tolerant plant. The root of A. yokoscense growing in areas contaminated with metals (Cu 3303 mg kg−1; Zn 9195 mg kg−1) contained maximum amount of Cu (5989 mg kg−1 dry weight) (CitationNishizono et al. 1987b). Usui et al. (1975) also indicated that the root of A. yokoscense contained 2463.6 ppm Cu in average. However, no information is available on As accumulation by A. yokoscense. Therefore, we examined the uptake of As and its interaction with heavy metals in A. yokoscense grown in mine soil and in media containing As-spiked soils.
MATERIALS AND METHODS
Field survey and sampling
The survey was conducted in the Silver Ikuno Park, which contains a closed silver mine (latitude 35ο10′0 N, longitude 134ο48′0 E), in Hyogo prefecture, Japan. More than 90% of the lkuno area is mountainous and it is approximately 300 m a.s.l. The main ores distributed in this area are chalcopyrite, sphalerite, galena, arsenopyrite, argentite, cassiterite and wolframite. These ores contain Cu, Cd, Zn, Pb, As, Ag, Sn and W (CitationAsami 1981). Intensive exploiting and smelting began in 1542 and its operation was ceased in 1973. At that time, the waste gases and the untreated wastewater from mining and smelting activities had caused long-term and severe contamination to local air, water, soil, vegetation and crops. At present, however, the contaminated areas are completely remediated and the mine area has been converted to a natural park.
In June 2004, more than 200 fern plants (with 4–5 fronds) with roots, and surface soils (0–10 cm) adjacent to the growing site of the fern were collected from the Ikuno mine area for greenhouse experiments. The size of the A. yokoscense plants was approximately 10 cm in height, and this was considered to be a young plant because the plants grow to a height of more than 20 cm in summer.
Greenhouse experiments
The mine soil (SIK) and an uncontaminated soil from a paddy field at Kochi University (FKU) were used for the greenhouse experiments. The SIK soil is classified as Dystrochrepts and the FKU soil is classified as Endoaquepts based on the USDA Soil Taxonomy classification system (CitationClassification Committee of Cultivated Soils 1996).
Experiment 1
Potassium arsenate (KH2AsO4) or sodium arsenite (NaAsO2) were added to the FKU soil at rates of 50, 100, 500 mg As (V) kg−1 or 5, 10, 50 mg As (III) kg−1, respectively. Both the FKU soil and the As-spiked FKU soil were thoroughly mixed with bark compost at a ratio of 2:1 (v:v), and kept for 2 weeks before use as the fern cultivation medium. FKU soil mixed with bark compost was used as a control. Each soil was then placed in a 1.5 L plastic pot, and one small 10 cm high fern plant with 4–5 fronds was planted in each pot. Each treatment was replicated four times. In addition, one set of pots containing media only (i.e. without a fern) was used to determine the influence of both watering and aging on the As concentration in the soils. After 21 weeks of cultivation, plants were harvested and washed with tap water to remove the soil and then quickly rinsed with 0.1 mol L−1 HCl solution to remove the metals adsorbed onto the root surface, followed by several rinses with deionized water. Then the plants were separated into aboveground and belowground parts (roots including rhizomes). The aboveground part of the plants was separated into young, mature and old fronds based on age. Biomass was measured on a DW basis (after 2 days at 55oC). Dried plant samples were ground to fine powder before analysis. Soil samples were taken from the pots both before transplanting and after harvest, air-dried and sieved with a 2-mm mesh for analytical use.
Experiment 2
Athyrium yokoscense was cultivated in the mine soil (SIK). One small live fern with 4–5 fronds and 10 cm in height was replanted in the SIK soils in a 1.5 L plastic pot and cultivated in a greenhouse at the Faculty of Agriculture, Kochi University, Kochi, Japan. This experiment was carried out with four replications. Neither chemical treatment nor fertilization was conducted to keep their original growing condition in nature. The plants were watered daily with tap water when necessary. The duration of the experiment was 21 weeks. Plant and soil samples were collected in the same way as experiment 1.
Analytical methods
General properties of the soils
The general soil properties were determined by following standard methods. Soil pH was measured using a pH meter with a combined electrode (Horiba, F-21, Kyoto, Japan). Cation exchange capacity (CEC) and the amounts of exchangeable bases (Ca, Mg, K and Na) were measured after successive extraction using 1 mol L−1 NH4-OAc
Table 1 Selected properties of the soils used in this study
Determination of As in soil and plant samples
A 0.1 g portion of the soil samples was weighed into a 120 mL Teflon digestion vessel, mixed with a mixture of concentrated HClO4, HNO3, HF and 20 g L−1 KMnO4 (2:3:5:2), and then heated on a hot plate at 100oC for 20 min. If the purple color of KMnO4 disappeared, 1 mL of 20 g L−1 KMnO4 was added and the vessel was again heated for 20 min. This procedure was repeated until the purple color did not disappear after 20 min of heating. Next, the temperature of the hot plate was set at 180–220oC until the solution in the vessel became almost dry. The hot plate was turned off and 20 mL of HCl (1 + 1) was added to dissolve the ingredients using the remaining heat of the hot plate. After cooling, the sample solution was transferred into a 50 mL polypropylene volumetric flask and filled up to 50 mL with deionized water and then filtered (Advantec No. 5C) (CitationTerashima 1984).
A 0.1 g portion of the plant samples was digested in a mixture of concentrated H2SO4 and 300 g L−1 H2O2 (3:2) in a Kieldal flask. After vigorous reaction, the solution became clear and the Kieldal flask was heated on a micro-Keildal digester at 300oC. If the solution became brown in color, the flask was removed from the digester; after several minutes of cooling, 2 mL of 300 g L−1 H2O2 was added and the flask as placed on the digester again; this procedure was repeated until the color of the solution remained colorless after 60 min consecutive heating at 300oC and white fumes of SO2 could be observed. After cooling, the solution was transferred into a 50 mL polypropylene volumetric flask filled up to 50 mL with deionized water and then filtered (Advantec No. 5C).
Available As in soils was extracted from a 5.0 g soil sample using 25 mL of 1 mol L−1 HCl by reciprocal shaking for 30 min at 30oC and then filtered (Advantec No. 5C). Water-soluble As in the soil was extracted from approximately 2.0 g of soil with 20 mL deionized water by reciprocal shaking for 30 min at 30oC. The suspension was then filtered (Advantec No. 5C).
For the measurement of As in the soil and plant digests and in the extracts of soils, 5 mL of the solution was mixed with 5 mL of concentrated HCl, 4 mL of 250 g L−1 ascorbic acid, 2.0 mL of 300 g L−1 KI and 2.0 mL of 20 g L−1 AlCl3, and then filled up with water to 20 mL immediately before measurement. Determination of As concentration was carried out using an inductively coupled plasma atomic emission spectrometer (ICP-AES, Shimadzu, ICPS-1,000 IV) combined with a hydride vapor generator (Shimadzu, HVG-1). The accuracy of the method was assessed using the certificated reference material soils (JSO-1, JSO-2) and plants (NIES CRM No. 1, NIES CRM No. 9) provided by the Geological Survey of Japan (GSJ) and the National Institute for Environmental Studies (NIES), Japan. Recoveries of the element ranged from 82 to 113%.
Distribution of As in soils
Arsenic distribution in the soil was estimated according to the sequential extraction method proposed by CitationKeon et al. (2001) with some modifications. A 1.5 g soil sample that was collected after fern cultivation was extracted sequentially with water, magnesium chlorite (1 mol L−1 at pH 7.0), sodium dihydrogenphosphate (1 mol L−1 at pH 5.0) and hydrochloric acid (1 mol L−1) and finally with a mixture of concentrated HClO4, HNO3, HF and 2% KMnO4 (2:3:5:2). The five sequential extraction steps were assumed to produce operationally defined fractions corresponding to water-soluble As (III) and As (V), ionically bound forms of As (III) and As (V), strongly bound forms of As (V) by monodentate or bidentate ligand exchange, specifically adsorbed As
(V) and occluded As by Mn oxides and amorphous Fe oxides, and As strongly bound to the residual phases, respectively.Determination of total and available heavy metals in soil and plant samples
Soil samples for measuring total heavy metals were microwave-digested in a mixture of HNO3 and HF at a ratio of 3:1 (Perkin Elmer, Multi-wave, Anton Paar, A-8054 Graz, Austria). Available heavy metals in soils were extracted by 0.1 mol L−1 HCl. Concentrations of heavy metals (Cd, Cu, Zn and Pb) in the sample solutions were determined by Atomic Absorption Spectrometer (AAS; Shimadzu, AA-6,800). The digested solutions of the plant samples previously obtained were used for heavy metal determination by AAS.
Statistical analyses
anovas were carried out using SPSS Version 11.0. Differences among treatment means were separated using Turkey's multiple range tests. Significance was tested at the 0.05% probability level. Pearson's correlations were also conducted.
RESULTS AND DISCUSSION
Both the SIK and FKU soils used in this study were acidic and had a sandy texture. As shown in , the FKU soil is an uncontaminated soil both in terms of As and heavy metals (Cd, Cu, Pb and Zn). The total As content of FKU soil was 7.6 mg kg−1, which is in the range of natural abundance of Japanese soils, 0.4–70 mg kg−1 with a mean of 11 mg kg−1, CitationIimura (1981), while SIK soil had an extremely high content of As and heavy metals.
Biomass production of A. yokoscense cultivated in a greenhouse
Growth of A. yokoscense was significantly affected by the chemical forms of As, and varied with the As levels in the soils () because of the phytotoxic effect of As (CitationKabata and Pendias 1991). The biomass of A. yokoscense with the addition of 500 mg As (V) kg−1 and 50 mg As (III) kg−1 FKU soils reduced both the aboveground and belowground biomass by 33% to 57% compared to the control (). In contrast, the biomasses obtained on the media containing 50 mg As (V) kg−1, 5 and 10 mg As (III) kg−1 FKU soils were not significantly different compared with that of the control, and the addition of 100 mg As (V) kg−1 FKU soil significantly increased the aboveground biomass. Among all treatments, the biomass (both aboveground and belowground) of the fern grown in mine soil was highest and differed significantly from the other treatments. Thus, in this experiment, the mine soil was the most favorable medium for fern growth. After 21 weeks of cultivation in mine soil, fern biomass increased markedly from 0.26 ± 0.08 to 4.3 ± 0.40 g plant−1 DW.
In the media treated at 500 mg kg−1 of As (V), and at 10, 50 mg kg−1 of As (III) FKU soils, symptoms of As toxicity appeared in the old fronds after 2 weeks, but the plants survived throughout the experiment. Thus, it is concluded that moderate As levels might promote the growth of the ferns. At much higher concentrations, As inhibited the growth of the plants and led to plant death.
Distribution of As in A. yokoscense cultivated in media containing As-spiked soils and mine soil
Arsenic concentration in fronds increased from young to old fronds grown in the media containing As-spiked soil. Arsenic concentrations in the mature and old fronds increased with As levels in the medium when As was supplied as As (V) (). In old fronds, the As concentration increased from 162 mg kg−1 (control) to 562 mg kg−1 at the 500 mg As (V) kg−1 level. However, As concentrations in young fronds were not significantly different between the control and the other treatments. These results suggested that detoxification or sequestration mechanisms may be present in mature and old fronds of A. yokoscence as described by CitationZhang et al. (2002) for P. vittata.
In the case of As (III) addition, the accumulation of As in fronds decreased with the addition of 10 and 50 mg As (III) kg−1 FKU soils. Among all treatments, the addition of 5 mg As (III) kg−1 FKU soil resulted in the highest As accumulation, with up to 922 ± 68 mg kg−1 in the old fronds. When levels of As in soils were not toxic for plant growth, a greater part of the absorbed As was probably translocated to the fronds, leading to greater concentrations in older fronds because they have been receiving As for a longer time. Most plants have relatively low As concentrations, typically < 1.5 mg kg−1 (CitationKabata and Pendias 1991) and 0.02–7 mg kg−1 DW (CitationBowen 1979). As the As concentration of A. yokoscense was much more than 100 mg kg−1 in fronds, it could be said that A. yokoscense is an As hyperaccumulator.
Figure 2 Arsenic concentration in different parts of Athyrium yokoscense cultivated in As-spiked soils and mine soils. Solid line represents the concentration of As in hyperaccumulation plants, 100-fold of central value of normal range, AS: 3.5 mg kg−1 (Bowen, 1979). SIK soil, mine soil.
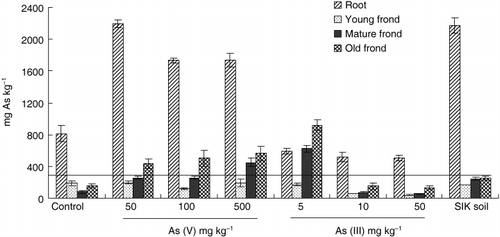
The concentration of As in the ferns planted in the mine soil was a little lower than that in the media containing As-spiked soils. This may be because the fern grown in the mine soil had a greater biomass production or there was less soluble As in the mine soil than the media containing As-spiked soils. Before transplanting, As concentrations of young and old fronds were 7.8 ± 0.3 and 57.7 ± 2.2 mg kg−1, respectively. After 21 weeks of cultivation in the mine soil, As concentration had increased to 167.6 ± 5, 242.4 ± 25 and 2,175.2 ± 97 mg kg−1, respectively ().
Contrary to the fronds, concentrations of As in the roots slightly decreased with increasing As levels in soils (). Arsenic concentration in roots with the addition of 50 mg As (V) mg−1 FKU soil was highest, followed by that in the mine soil. The addition of As (III) to the soils reduced the As concentrations in roots. As both the biomass and the As concentrations in the roots of the ferns grown in the media containing As (III) were lower than those in the control, it demonstrated that the addition of As (III) at levels greater than 10 mg kg−1 to the FKU soil inhibited normal root growth, thus, resulting in As toxicity. Compared to other As hyperaccumulators, such as P. vittata or P. calomelanos, A. yokoscense has a lower capacity to uptake As to its biomass. Athyrium yokoscense accumulated As mostly in the roots, while As is predominantly concentrated in the fronds of P. vittata and P. calomelanos. The concentrations of As in fronds of P. vittata and P. calomelanos were 7,230 and 8,000 mg kg−1, respectively (CitationFrancesconi et al. 2002; CitationTu et al. 2002). Thus, the As tolerant mechanisms in A. yokoscense might be different from those in P. vittata and P. calomelanos.
Arsenic tolerance and detoxification mechanisms in P. vittata have been reported by CitationZhang et al. (2002). Although a strong and positive correlation between As concentration and acid-soluble thiols (phytochelatin) in plant leaflets was found, they suggested that phytochelatin could play a minor detoxification role in this hyperaccumulator because only a very small part of As is complexed by phytochelatin. Although metal tolerant mechanisms have been reported in A. yokoscense, to date, As detoxification and tolerant mechanisms are unclear. Further study should be conducted to elucidate As species in plants to understand its hyperaccumulation as well as the detoxification mechanisms, and the influence of nutrients (e.g. P, Fe) on the growth and the simultaneous accumulation of As and heavy metals in A. yokoscense.
Distribution of As in media containing As-spiked soils after cultivation
Mobility, bioavailability and toxicity of trace elements depend strongly on their chemical form and type of binding. Consequently, determination of total As concentrations is insufficient to estimate potential risks of remobilization and uptake of liberated As by biota.
The sum of each fraction obtained by sequential extraction procedures agreed well with total As in soils obtained by digestion (recovery varied from 81.2 to 99.6%). The forms of As in the soil media containing As-spiked FKU soils with and without ferns (designed as soil + f and soil − f, respectively) are presented in . Despite the difference in total As concentration, the proportion of As among the five sequentially extracted fractions was similar for all soil media, except for the mine soil. Almost 10% of the total As content was extracted during the first step (water-soluble As) and less than 7.5% of As was found in the second fraction, which corresponds to ionically bound forms of As. The majority of the As was extracted in the third fraction, which included strongly adsorbed As via the ligand exchange mechanism. This result agrees with another study that examined soils in Cornwall in southwest England (CitationCaille et al. 2004). They found that more than 60% of As was extracted in the fraction that corresponds to As adsorbed to amorphous and poorly crystalline hydrous oxides of Fe and Al. A considerable amount of As was also associated with the amorphous Fe hydroxides fraction. The residual fraction accounted for approximately 10% of the total As in the media containing As (V) spiked soils, and 10–14% of the total As in the media spiked with As (III). The fractional distribution of As in the mine soil (SIK) indicated that only a small proportion (less than 3%) of total As was present in water-soluble and MgCl2 fractions. The data also indicated that mine soil contained higher proportions of As in the residual fraction (accounting for 62%) compared with the media containing As-spiked FKU soils.
Heavy metal accumulation in A. yokoscense cultivated in mine soil
Athyrium yokoscense could accumulate not only As but also heavy metals (Cd, Cu, Pb and Zn) when cultivated in the mine soil. The concentrations of heavy metals in A. yokoscense were significantly higher than the values reported for terrestrial plants (Cd 0.1–2.4 mg kg−1; Pb 1–13 mg kg−1; Cu 1–30 mg kg−1; Zn 20–400 mg kg−1; CitationBowen 1979). The fern accumulated Cd mainly in the fronds, with concentrations of 335 ± 84 (young), 959 ± 96 (mature) and 1,095 ± 120 (old) mg kg−1, respectively (). The concentration of Cd in the tissue of A. yokoscense was in the following order: roots < young frond < mature frond < old frond, which is typical of an accumulator species. Morishita and Boratynski (1992) detected that at polluted areas in Bandai, Fukushima, A. yokoscense exhibited high levels of Cd up to 996 mg kg−1 in leaflets, while
Figure 3 Arsenic distribution in the As-spiked soils and mine soils after cultivation. f, fern; SIK soil, mine soil; W-s: water soluble-As; Res: residual.
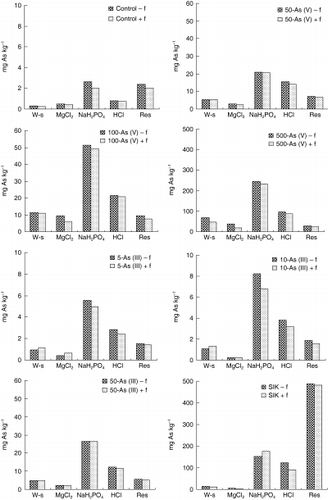
Figure 4 Heavy metal accumulation in Athyrium yokoscense cultivated in mine soil. Solid lines represents the concentration of As in hyperaccumulation plants, 100-fold of central value of normal range, Cd: 1.2 mg kg−1 (Bowen, 1979).
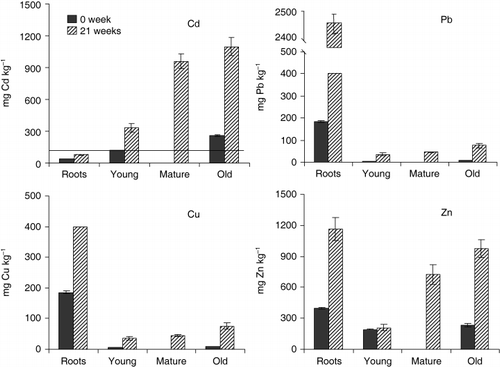
CitationCaille et al. (2004) reported that P. vittata could hyperaccumulate As from naturally contaminated soil, but grew poorly on soil that had extremely high contents of Zn and Cu (1221 and 5,500 mg kg−1, respectively). These authors reported that the As and Cu concentration in P. vittata was 3,510 and 7.0 mg kg−1 when the fern was grown on soil containing 475 mg kg−1 As and 370 mg kg−1 Cu (Roscroggan soil), respectively. In the present study, A. yokoscense accumulated 242 mg kg−1 As and 88 mg kg−1 Cu from soil containing 814 mg kg−1 As and 343 mg kg−1 Cu. Thus, at a similar Cu concentration in soil, P. vittata can accumulate much more As than A. yokoscense, but Cu accumulation was lower than that by A. yokoscense. In contrast, when P. vittata was cultivated in a soil containing 4,550 mg kg−1 As, 3,903 mg kg−1 Pb and 1,242 mg kg−1 Zn, the accumulation of As in P. vittata (362 mg kg−1 in fronds) was almost the same as A. yokoscense that was grown in the mine soil (814 mg kg−1 As, 3,464 mg kg−1 Pb and 2,422 mg kg−1 Zn) (CitationCaille et al. 2004). CitationFayiga et al. (2004) also indicated that, although effective in taking up As (up to 4,100 mg kg−1), P. vittata had a limited capacity to take up other metals. Compared to P. vittata, it is proposed that the potential for heavy metal accumulation from As and heavy metal contaminated soils is higher for A. yokoscense, particularly when the As level in the soil is not extremely high.
Potential of A. yokoscense for As and heavy metal phytoremediation
For the practical application of phytoremediation, the total amount of metal accumulation by A. yokoscense must be considered to measure the potential effectiveness of a plant for phytoextraction (CitationFrancesconi et al. 2002; CitationTu et al. 2002). The original soil metal removed by one crop is expressed as a percentage of metal accumulation by the plant (plant metal concentration × biomass) to the metal in the soil (soil metal concentration × soil mass) (CitationTu et al. 2002; CitationZhao et al. 2003). Approximately 0.2–8.8% of the initial soil As was removed by A. yokoscense after 21 weeks of cultivation. Among the six As levels tested, the highest removal of As was in the media containing 50 mg As (V) kg−1 FKU soil, accounting for 8.84%. The removals of the other treatments were 0.89, 6.16, 2.27, 0.26, 5.15, 2.37 and 0.82% for the mine soil, control-FKU soil, the media containing 100 mg As (V) kg−1, 500 mg As (V) kg−1, 5 mg As (III) kg−1, 10 mg As (III) kg−1 and 50 mg As (III) kg−1, respectively. Assuming a constant rate of As extraction as above, it would take 12–15 harvests (250–300 weeks) to remediate soil contaminated with 50 mg As kg−1. For P. vittata, the accumulation of As in its biomass accounted for up to approximately 26% of the initial As in the soil spiked with As at a level of 100 mg kg−1 (CitationTu et al. 2002). Thus, P. vittata would take less time than A. yokoscense, only 4 or 5 harvests (80–100 weeks), to phytoremediate a soil contaminated with 100 mg As kg−1. In addition, 13.7, 0.37, 0.24 and 0.20% of Cd, Cu, Pb and Zn, respectively, in the mine soil were removed by A. yokoscense in the greenhouse cultivation.
Although the direct application of A. yokoscense to As and heavy metal phytoremediation is not a promising approach practically, soil amendments or the application of fertilizers might assist A. yokoscense to increase its As and heavy metal removal from contaminated soils.
ACKNOWLEDGMENTS
We thank Mitsubishi Material for providing soils and fern samples. This research was supported by a Sasakawa Scientific Research Grant (No. 16–317) from The Japan Science Society to T. K. Van and by the Grant-in-Aid for Scientific Research (B, No. 15380223) from Ministry of Education, Culture, Sports, Science and Technology (MEXT) of Japan to K. Iwasaki.
REFERENCES
- Chaney , RL , Malik , M Li , YM . 1997 . Phytoremediation of soil metals . CurrOpinBiotech , 8 : 279 – 284 .
- Cunningham , SD , Berti , WR and Huang , JW . 1995 . Phytoremediation of contaminated soils . Trends Biotechnol , 13 : 393 – 397 .
- Ward , OP and Singh , A . 2004 . “ Soil bioremediation and phytoremediation—An overview ” . In Applied Bioremediation and Phytoremediation , Edited by: Singh , A and Ward , OP . 7 Heidelberg : Springer-Verlag .
- Tu , C and Ma , LQ . 2002 . Effects of arsenic concentrations and forms on arsenic uptake by the hyperaccumulator Ladder brake . JEnvironQual , 31 : 642 – 647 .
- Caille , N , Swanwick , S , Zhao , FJ and McGrath , SP . 2004 . Arsenic hyperaccumulation by Pteris vittatafrom arsenic contaminated soils and the effect of liming and phosphate fertilization . EnvironPollut , 131 : 113 – 120 .
- Francesconi , K , Visootiviseth , P , Sridokchan , W and Goessler , W . 2002 . Arsenic species in an arsenic hyperaccumulating fern, Pityrogramma calomelanos: a potential phytoremediator of arsenic-contaminated soils . SciTotal Environ , 284 : 27 – 35 .
- Lombi , E , Zhao , FJ , Dunham , SJ and McGrath , SP . 2001 . Phytoremediation of heavy metal-contaminated soils: Natural hyperaccumulation versus chemically enhanced phytoextraction . JEnvironQual , 30 : 1919 – 1926 .
- Tu , C , Ma , LQ and Bondada , B . 2002 . Arsenic accumulation in the hyperaccumulator Chinese brake and its utilization potential for phytoremediation . JEnvironQual , 31 : 1671 – 1675 .
- Adriano , D . 2001 . “ Arsenic ” . In Trace Elements in Terrestrial Environments: Biochemistry, Bioavailability, and Risks of Metals , Edited by: Adriano , DC . 219 – 263 . New York : Springer-Verlag .
- Baker , AJM and Brooks , RR . 1989 . Terrestrial higher plants which hyperaccumulate metallic elements—a review of their distribution, ecology and photochemistry . Biorecovery , 1 : 81 – 126 .
- McGrath , SP , Zhao , FJ and Lombi , E . 2002 . Phytoremediation of metals, metalloids, and radionuclides . AdvAgron , 75 : 1 – 56 .
- Kubota , H and Takenaka , C . 2003 . Arabis gemmiferais a hyperaccumulator of Cd and Zn . IntJPhytoremediat , 5 ( 3 ) : 197 – 201 .
- Lombi , E , Zhao , FJ , Dunham , SJ and McGrath , SP . 2000 . Cadmium accumulation in populations of Thlaspi caerulescens and Thlaspi goesingense . New Phytol , 145 : 11 – 20 .
- Robison , BH , Lombi , E , Zhao , FJ and McGrath , SP . 2003 . Uptake and distribution of nickel and other metals in hyperaccumulator Berkheya coddii . New Phytol , 158 ( 2 ) : 279 – 285 .
- Zu , Y , Li , Y , Chen , J , Chen , H , Qin , L and Schvartz , C . 2005 . Hyperaccumulator of Pb, Zn, and Cd in herbaceous grown on lead–zinc mining area in Yunnan, China . EnvironInt , 31 ( 5 ) : 755 – 762 .
- Ma , LQ , Komar , KM , Tu , C , Zhang , W , Cai , Y and Kennelley , DE . 2001 . A fern that hyperaccumulates arsenic . Nature , 409 : 579
- Zhao , FJ , Dunham , SJ and McGrath , SP . 2002 . Arsenic hyperaccumulation by different fern species . New Phytol , 156 : 27 – 31 .
- McIntyre , T . 2003 . “ Phytoremediation of heavy metals from soils ” . In Advances in Biochemical Engineering/Biotechnology , Edited by: Scheper , T and Tsao , DT . Vol. 78 , 97 – 123 . Berlin : Springer-Verlag . Phytoremediation
- Tsutsumi , M . 1981 . “ Arsenic pollution in arable land ” . In Heavy Metal Pollution in Soils of Japan , Edited by: Kitagishi , K and Yamane , I . 181 – 192 . Tokyo : Japan Scientific Societies Press .
- Hiroi , T . 1981 . “ Vegetation in mining areas ” . In Heavy Metal Pollution in Soils of Japan , Edited by: Kitagishi , K and Yamane , I . 219 – 229 . Tokyo : Japan Scientific Societies Press .
- 2005 . KangY VanTK FukuiT IwasakiK SakuraiK2005The accumulation of As in Athyrium yokoscensewhich grows in As-spiked soils and mine soil . Abstracts of the Annual Meeting, Japanese Society of Soil Science and Plant Nutrition . September 7 2005 , Shimane, Japan. Vol. 51 , pp. 184 (in Japanese)
- Kamachi , H , Komori , I Tamura , H . 2005 . Lead tolerance and accumulation in the gametophytes of the fern Athyrium yokoscense . JPlant Res , 118 : 137 – 145 .
- Nishizono , H , Ichikawa , H , Suzuki , S and Ishii , F . 1987a . The role of the root cell wall in the heavy metal tolerance of Athyrium yokoscense . Plant Soil , 101 : 15 – 20 .
- Sakai , Y , Fukuoka , T and Honjo , T . 1991 . Characterization of lead in the tissues of heavy metal tolerant plant, Athyrium yokoscense(Fr. Et Sav.) . ChristChemSocJapan , 5 : 416 – 421 . (in Japanese with English abstract)
- Yoshihara , T , Tsunokawa , K Miyano , Y . 2005 . Induction of callus from a metal hypertolerant fern, Athyrium yokoscense, and evaluation of its cadmium tolerance and accumulation capacity . Plant Cell Rep , 23 : 579 – 585 .
- Nishizono , H , Suzuki , S and Ishii , F . 1987b . Accumulation of heavy metals in the metal-tolerant fern, Athyrium yokoscense, growing on various environments . Plant Soil , 102 : 65 – 70 .
- Asami , T . 1981 . “ The Ichi and Maruyama River Basins: Soil pollution by cadmium, zinc, lead and copper discharged from Ikuno mine ” . In Heavy Metal Pollution in Soils of Japan , Edited by: Kitagishi , K and Yamane , I . 125 – 136 . Tokyo : Japan Scientific Societies Press .
- Classification Committee of Cultivated Soils . 1996 . Classification of Cultivated Soils in Japan , Tsukuba : National Institute of Agro-Environmental Sciences . Available from URL: http://soilgc.job.affrc.go.jp/Document/Classification.pdf
- Terashima , S . 1984 . Determination of arsenic and antimony in geological materials by automated hydride generation and electrothermal atomic absorption spectrometry . Bunseki Kagaku , 33 : 561 – 563 . (in Japanese with English summary)
- Keon , NE , Swartz , CH , Brabander , DJ , Harvey , C and Hemond , HF . 2001 . Validation of an arsenic sequential extraction method for evaluating mobility in sediments . EnvironSciTech , 35 : 2778 – 2784 .
- Iimura , K . 1981 . “ Background contents of heavy metals in Japanese soils ” . In Heavy Metal Pollution in Soils of Japan , Edited by: Kitagishi , K and Yamane , I . 19 – 26 . Tokyo : Japan Scientific Societies Press .
- Kabata-Pendias , A and Pendias , H . 1991 . “ Arsenic ” . In Trace Elements in Soils and Plants , Edited by: Kabata-Pendias , A . 203 – 209 . Boca Raton : CRC Press .
- Zhang , W , Cai , Y , Tu , C and Ma , LQ . 2002 . Arsenic speciation and distribution in an arsenic hyperaccumulating plant . SciTotal Environ , 300 : 167 – 177 .
- Bowen , HJM . 1979 . Environmental Chemistry of the Elements , London : Academic Press .
- Nishizono , H , Minemure , H , Suzuki , S and Ishii , F . 1988 . An inducible copper–thiolate complex in the fern Athyrium yokoscense: Involvement in copper-tolerance of the fern . Plant Cell Physiol , 29 ( 8 ) : 1345 – 1351 .
- Xian , X . 1996 . Relationship between the distribution of Cd and Zn in root tissue and the heavy metal tolerance of mulberry and Athyrium yokoscense . JSericSciJpn , 64 ( 4 ) : 286 – 291 .
- Fayiga , OA , Ma , LQ , Cao , X and Rathinasabapathi , B . 2004 . Effects of heavy metals on growth and arsenic accumulation in the arsenic hyperaccumulator Pteris vittataL . EnvironPollut , 132 : 289 – 296 .
- Zhao , FJ , Lombi , E and McGrath , SP . 2003 . Assessing the potential for zinc and cadmium phytoremediation with the hyperacumulator Thlaspi caerulescens . Plant Soil , 249 : 37 – 43 .