Abstract
Aluminum (Al)–humus complexes are abundant in the A horizons of non-allophanic Andosols and contribute to the unique properties of volcanic ash soils, such as high reactivity with phosphate ions and a low bulk density. Natural non-allophanic Andosols commonly show Al toxicity to plant roots. There have been very few studies examining the contribution of Al–humus complexes to the Al toxicity of plant roots, although the complexes are the probable source of the toxic Al. We extracted humic substances from the A horizon of a non-allophanic Andosol using NaOH solution and reacted the humic substances and partially neutralized the AlCl3 solution at three pH conditions (pH 4.0, 4.5 and 5.5) to prepare pure Al–humic substance complexes. The Al solubility study (equilibrium study in 10−2 mol L−1 CaCl2) and the Al release study (a stirred-flow method using 10−3 mol L−1 acetate buffer solution adjusted to pH 3.5) indicated that all the synthetic complexes easily and rapidly release monomeric Al into the liquid phase with slight changes in pH and ion strength, although the Al contents and their extent of polymerization are considerably different among the complexes. A plant growth test was conducted using a medium containing the Al–humic substance complexes and perlite mixture. Root growth in burdock (Arctium lappa) and barley (Hordeum vulgare L.) was reduced equally by all three complex media, and the roots showed the typical injury symptoms of Al toxicity. These results indicate that in soils dominated by Al–humus complexes the Al released from the Al–humus complexes, as well as the exchangeable Al adsorbed by soil minerals, is definitely toxic to plant roots.
INTRODUCTION
Andosols or Andisols, the typical soils developed from volcaniclastic materials, are divided into two major groups on the basis of their colloidal compositions (CitationFood and Agriculture Organization et al. 1998; CitationNanzyo et al. 1993; CitationThe Fourth Committee for Soil Classification and Nomenclature 2002). One group is referred to as “allophanic” Andosols, whose colloidal fraction is dominated by allophanic clay materials. The other group is “non-allophanic” Andosols, which are dominated by aluminum (Al)–humus complexes and 2:1 type aluminosilicates. Both groups of Andosols show the unique properties of volcanic ash derived soils, such as high reactivity with phosphate and fluoride ions and a low bulk density. However, there are large differences in the soil acidity between the two groups of Andosols (CitationNanzyo et al. 1993). Allophanic Andosols are moderate to slightly acidic, even when the base saturation is very low, and rarely contain a toxic level of KCl-extractable Al. In contrast, non-allophanic Andosols are strongly acidic when the base saturation is low and possess a high KCl-extractable Al that is toxic to plant roots (CitationSaigusa et al. 1980; CitationShoji et al. 1980). Unbuffered salt solutions, such as KCl solution, are generally believed to remove exchangeable Al ions electrostatically bonded to permanently charged sites (CitationJardine and Zelazny 1996). In the case of acidic Andosols, the origin of KCl-extractable Al has also been attributed primarily to Al3+ adsorbed to 2:1 minerals (CitationDahlgren and Saigusa 1994; CitationSaigusa et al. 1980; CitationShoji et al. 1980).
Humus-rich A horizons of non-allophanic Andosols generally contain abundant amounts of organic matter and organically complexed Al. The Al solubility of the soil horizons was significantly influenced by the ion exchange reaction of the H+ and Al ions on the negatively charged sites of the organic matter (CitationTakahashi et al. 1995). Part of the Al complexed with humus was easily released using acidic buffer solutions (CitationTakahashi et al. 1995). Thus, Al–humus complexes are considered to control the Al solubility of non-allophanic Andosols. In some soil samples dominated by Al–humus complexes, a large amount of Al other than the exchangeable Al, including the organically complexed Al, is assumed to react during the 1 mol L−1 KCl extraction for determination of the exchangeable Al (CitationTakahashi and Dahlgren 1998). Furthermore, there is a close relationship between the exchangeable Al (1 mol L−1 KCl extractable Al) and the organically complexed Al (pyrophosphate extractable Al) of the humus horizons of non-allophanic Andosols from the extensive area of eastern Japan (CitationTakahashi et al. 2003). These observations suggest that the exchangeable Al is equilibrated with organically complexed Al through aqueous Al, and that the organically complexed Al may be one of the sources of phytotoxic Al. Furthermore, the probability that part of the organically complexed Al may be toxic to plant roots cannot be definitively ruled out, although this assumption is contradictory to the fact that organic matter tends to ameliorate Al toxicity (e.g. CitationWong and Swift 1995). Clarifying the bioavailability of Al in Al–humus complexes will lead to the effective improvement of acid soils dominated by Al–humus complexes and to the beneficial use of these types of acid soils. Andosols dominated by Al–humus complexes reduce some soil-born diseases that are sensitive to Al toxicity (CitationFuruya et al. 1999; CitationMizuno et al. 1998). Application of gypsum or phosphogypsum, substitutes for lime materials, was not effective in the amelioration of Al toxicity of humus-rich Andosols (CitationSaigusa et al. 1996; CitationTakahashi et al. 2006b). This may relate to the nature of Al–humus complexes.
To verify the possibility of the potential toxicity of organically complexed Al to plant roots, we have to establish the conditions without the influence of soil minerals for the plant toxicity test. We can either dissolve soil minerals using HCl-HF treatment without any influence to the soil humus (CitationSunehez-Monedero et al. 2002) or effectively extract the soil humic substances using alkaline solutions (CitationAmerican Society of Agronomy and Soil Science Society of America 1982). In the present study we chose the latter method: the humic substances were extracted using alkaline solution (NaOH solution) and reacted with hydroxy Al solution to synthesize the Al–humic substance complexes according to CitationHigashi (1983). We examined the chemical structural properties and the Al solubility of the synthetic Al–humic substance complexes, and their ability to induce Al toxicity in plant roots.
MATERIALS AND METHODS
Synthesis of Al–humic substance complexes
The soil sample used in this study was derived from the Field Science Center (Kawatabi), Tohoku University, Miyagi Prefecture, Japan (38°44′53″ N, 140°45′08″ E). The Kawatabi soil is a typical non-allophanic Andosol and is classified as Pachic Melanudant (CitationIto and Saigusa 1996) according to Soil Taxonomy (CitationSoil Survey Staff 1999).
The Al–humic substance complexes were synthesized according to the method of CitationHigashi (1983), which includes the following three steps: (1) preparation of humic substance solution, (2) preparation of hydroxyl Al solution, (3) synthesis of the complexes.
Extraction and preparation of a humic substance
A humic substance was obtained by the extraction of the A horizon sample from the Kawatabi soil with 0.125 mol L−1 NaOH solution (soil:solution = 1:50 w/v). After a 16-h extraction, the suspended fine soil particles were removed by centrifugation (38,900 g, 30 min) after the addition of Superfloc (Cytec Industries, West Paterson, NJ, USA). The humic substance in 0.125 mol L−1 NaOH was dialyzed against deionized water until the pH of the extractant became neutral (approximately 72 h). The resulting humic substance was then treated with a cation exchange resin (Amberlite IR-120) of the H+ form. The ash content of the humic substance was 2.6%.
Preparation of hydroxy Al solutions
Three ionic solutions of hydroxy Al were formed with pH values of 4.0, 4.5 and 5.5 by the slow addition of 0.1 mol L−1 NaOH (0.3 mL min−1) to a continuously stirred 0.1 mol L−1 AlCl3. The resulting solutions were diluted to 0.02 mol L−1 Al with deionized water. The OH/Al mole-ratios of the hydroxy Al solution were 1.35, 2.35 and 2.51 for the pH 4.0, pH 4.5 and pH 5.5 solutions, respectively.
Synthesis of Al–humic substance complexes
The pH of the solution of the humic substances was adjusted with dilute NaOH to match those of the hydroxy Al solutions. The hydroxy Al solutions were added to the solution of humic substances until they were completely flocculated. The flocculated Al–humic substance complexes were centrifuged and washed with 80% ethanol until free of Cl− in the supernatants, dried at room temperature, and then ground into fine powders. The synthetic Al–humic substance complexes at pH 4.0, 4.5 and 5.5 were then labeled as Complex (4.0), Complex (4.5) and Complex (5.5), respectively.
Analytical methods
Aluminum and carbon contents in extracts of synthetic complexes (CitationHigashi 1983)
Twenty milliliters of 0.1 mol L−1 sodium hydroxide in a 0.05 mol L−1 sodium tetraborate solution or 0.1 mol L−1 sodium pyrophosphate solution and 20 mg of a synthetic complex were shaken in a polyethylene centrifuge tube for 16 h. Superfloc was added and the suspension was obtained after centrifugation for 30 min at 38,900 g. The Al and C contents in the supernatant were determined using atomic absorption spectrometry (A2000, Hitachi, Tokyo, Japan) and a TOC analyzer (TOC-VCHS, Shimadzu, Kyoto, Japan), respectively.
Visible photoabsorption spectrum of NaOH extracts and melanic index
Approximately 20 mg of the complexes was shaken with 25 mL of 0.125 mol L−1 NaOH solution for 1 h. One drop of Superfloc was added and the mixture was centrifuged for 10 min at 3,000 g. The supernatant was appropriately diluted with 0.025 mol L−1 NaOH and the absorbance was scanned from 400 nm to 700 nm. The melanic index was obtained from the calculation of the ratio of the absorbance at 450 nm over that at 520 nm (CitationHonna et al. 1988; CitationSoil Survey Staff 1999). As a comparison, the analysis was also done using the original soil sample and its soil humic substance.
Speciation of C and Al using solid-state magic angle spinning nuclear magnetic resonance
The chemical speciation of C in the original soil sample and the extracted humic substance and that of Al in the Al–humic substance complexes was carried out using the JNM-α300 Fourier transform (FT)-nuclear magnetic resonance (FT-NMR) system (JEOL, Tokyo, Japan). The powdered samples were transferred to a KEL-F spinning tube (6 mm ø, JEOL). The 13C signals were recorded using the cross polarization magic angle spinning (CPMAS) technique and observed at 75.45 MHz with 6–8 kHz of MAS, 1 msec of contact time and a 3-s pulse interval. A broadening factor of 100 Hz was used in the Fourier transformation procedure. A standard chemical shift (0 p.p.m. tetramethylsilane) adjustment was carried out using adamantane (29.50 p.p.m.). The signals of 27Al were recorded at 78.2 MHz in a single-pulse experiment without decoupling, with 6–8 kHz of MAS, a flip angle of π/2 for 27Al (0.9 µs as a pulse width), observation band of 80 kHz, and an observation point of 4,096 (resolution: 19.53 Hz). A broadening factor of 20 Hz was used in the Fourier transformation procedure. The standard chemical shift (0 p.p.m.) was adjusted using a 1 mol L−1 AlCl3 solution.
Aluminum release rates from Al–humic substance complexes
The rates of Al release from the Al–humic substance complexes were determined using a stirred flow cell as described by CitationDahlgren and Walker (1993) and CitationTakahashi et al. (1995). A reaction solution consisting of 10−3 mol L−1 sodium acetate/hydrochloric acid adjusted to pH 3.5 was used for the Al release experiment. The reacted solution was continuously injected into an autoanalyzer using the pyrocatechol violet method for quantification of the monomeric Al (CitationRogeberg and Henriksen 1985).
Aluminum solubility of Al–humic substance complexes
An equilibrium study was conducted in a 0.01 mol L−1 CaCl2 solution system (CitationDahlgren and Walker 1993) to determine the solubility of Al as a function of pH in the Al–humic substance complexes. Thirty milliliters of a 0.01 mol L−1 CaCl2 solution was added to approximately 30 mg of sample, and HCl or NaOH was added to provide a pH range of 3.5–5.5. The suspensions were incubated for 7 days at 25°C and the supernatant was obtained after centrifugation at 27,700 g for 10 min and filtration through a 0.45 µm membrane filter. The monomeric Al concentrations were determined using the ferron-o-phenanthroline method (CitationAmerican Society of Agronomy and Soil Science Society of America 1982). The activity of Al3+ was estimated using Visual MINTEQ (downloaded from http://www.lwr.kth/se/English/OurSoftware/vminteq). As dissolved organic carbon was not taken into consideration, the calculated Al3+ activity should be considered a maximum value.
Plant culture
Plant culture was carried out in a mixture of the Al–humic substance complexes and perlite. A commercial horticultural perlite was crushed, passed through a 1-mm mesh, washed thoroughly with dilute HCl and then rinsed with pure water until the chloride ion was not detected by AgNO3 solution. A dry weight of 2.5 g perlite was mixed with 125 mg of the Al–humic substance complexes and the mixture was added to a tube (20 mm diameter and 30 mm height). The water content was adjusted and kept at 70% of the maximum water holding capacity. Three seedlings of burdock (Arctium lappa) or barley (Hordeum vulgare L.) per one tube were sown and cultured at room temperature (25–27°C). As controls, we used a mixture of perlite and Ca–humic substance complexes (prepared from 0.1 mol L−1 CaCl2 solution and the humic substance solution at pH 5) and perlite by itself (without any complexes). All the cultural experiments were done without any nutrient element. After a 14-day culture for burdock and a 7-day culture for barley, plants were harvested and the length of the roots was measured. Analysis of the significant difference among the means was conducted using a t-test. Just after harvest, the pH values of the culture medium solution were measured using a compact pH meter (Twin pH B-212, Horiba, Kyoto, Japan) that is able to measure pH values from a single drop of sample.
RESULTS
Characteristics of synthetic Al–humic substance complexes
The solid-state CPMAS 13C-NMR spectrum of Kawatabi A horizon () showed the presence of aliphatic C (resonated at 32 p.p.m.), O-alkyl C (103 and 72 p.p.m.), aromatic C (131 p.p.m.) and carbonyl C (175 p.p.m.). Among them, the aromatic C and carbonyl C were concentrated in the extracted humic substances ().
Figure 1 Solid-state cross polarization magic angle spinning (CPMAS) 13C-nuclear magnetic resonance (NMR) spectra of (A) Kawatabi A horizon soil and (B) its extracted humic substance used in the present study. *, Spinning side band.
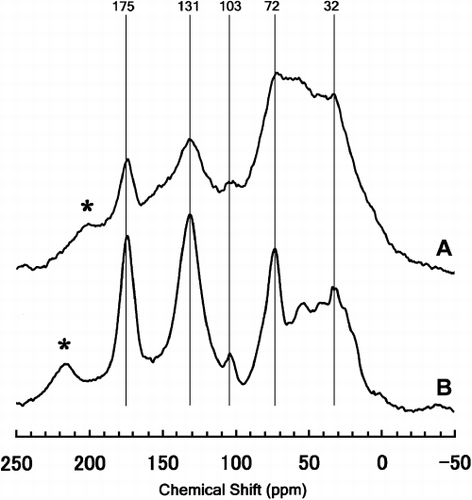
Figure 2 Visible photoabsorption spectra of extracts with NaOH solution from Kawatabi A horizon soil, its extracted humic substance and synthetic Al–humic substance complexes.
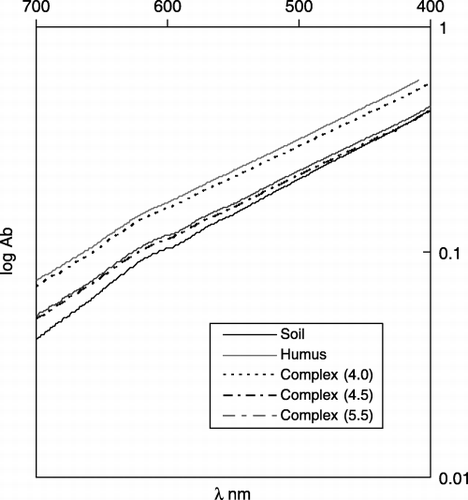
Table 1 Melanic indices (MI) of original Kawatabi A horizon soil, humic substance extracted the soil samples and synthetic Al–humus complexes
shows the optical absorption spectra of the NaOH extracts from the Al–humic substance complexes, the humic substance and the original soil. All spectra possessed similar absorption patterns, which show the presence of an A-type humic acid with a high degree of humification (CitationIto and Saigusa 1996). Although the melanic indices of the Al–humic substance complexes were slightly lower than that of the original soil, they satisfied the criterion for melanic horizon (melanic index < 1.70) (CitationHonna et al. 1988; CitationSoil Survey Staff 1999) ().
The Al and C contents of the Al–humic substance complexes and the extractability of Al and C from the complexes by sodium pyrophosphate and sodium hydroxide-tetraborate solutions are shown in . When the pH increased from 4 to 5.5 during the
Table 2 Total contents of Al and C in synthetic Al–humic substance complexes and extractable Al and C with sodium pyrophosphate and sodium hydroxide-tetraborate
The solid-state MAS 27Al-NMR spectrum of Complex (4.0) showed only one peak at approximately 0 p.p.m., which is attributed to the octahedral Al (). On the contrary, the spectrum of Complex (4.5) possessed a distinct peak at 63 p.p.m. in addition to the 0 p.p.m. peak, and a stronger peak at 63 p.p.m. was observed for the spectrum of Complex (5.5). The 63 p.p.m. peak is attributed to the tetrahedral Al situated at the center of the Al tridecamer (Al13) (CitationHiradate and Yamaguchi 2003). These NMR analysis results agreed with the selective extraction of Al; the amounts of Al13 from the NMR analysis corresponded to the differences between the pyrophosphate-extractable Al and sodium hydroxide-tetraborate-extractable Al (Alp–Alna, ).
Aluminum release rates and Al solubility of synthetic Al–humic substance complexes
As shown in , monomeric Al was easily released from the synthetic Al–humic substance complexes using the 10−3 mol L−1 acetic buffer solution (pH 3.5). The Al release rates from the Al–humic substance complexes are in the following order: Complex (4.5) > Complex (4.0) = Complex (5.5). This trend is similar to the amount
Figure 3 Solid-state magic angle spinning (MAS) 27Al-nuclear magnetic resonance (NMR) spectra of humic substance extracted from Kawatabi A horizon and synthetic Al–humic substance complexes.
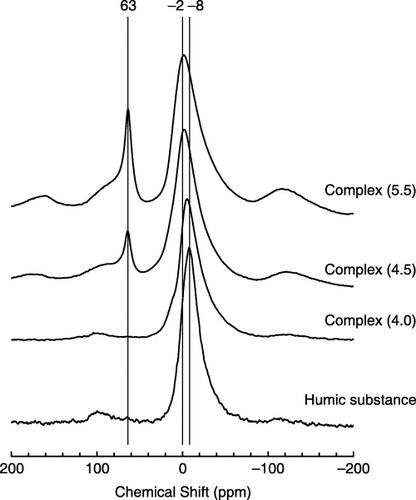
Figure 4 Release rates of Al from synthetic Al–humic substance complexes with 10−3 mol L−1 acetate buffer adjusted to pH 3.5.
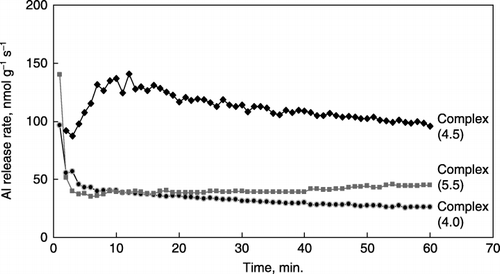
Figure 5 Plot of equilibrium Al solubility versus pH for synthetic Al–humic substance complexes. The solubility of synthetic gibbsite is indicated by the dotted line for comparison. Solubility expressions were pAl = 0.96 pH + 1.09 for Complex (4.0), pAl = 1.43 pH + 1.36 for Complex (4.5), and pAl = 1.49 pH + 1.86 for Complex (5.5).
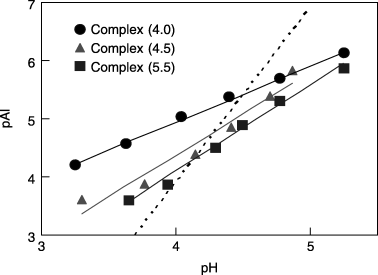
The results of the equilibration study conducted to examine the Al solubility are shown in . The dotted line in indicates the solubility of synthetic gibbsite with a slope of 3.0 and log K*so of 8.1 for the following reaction:
The values of the slopes of the A horizon soils dominated by the Al–humus complexes were 2.2–2.5, significantly lower than the 3.0 recorded for the Al(OH)3 minerals, thus, the Al solubility of these soils was considered to be largely controlled by the exchange reaction of the H+ and Al ions on the negatively charged sites of the organic matter (CitationTakahashi et al. 1995; CitationTakahashi et al. 2003). As for the organic horizons of Spodosols (CitationCronan et al. 1986), slopes of 0.4–1.3 were observed. The slopes of the solubility line for the synthetic Al–humic substance complexes ranged from 0.96 to 1.49 () and were closer to those of the organic horizons than to those of the A horizon soils. With an increase in pH during synthesis, Al solubility of the Al–humic substance complexes increased and the slope was closer to that of gibbsite. However, it is noteworthy that the Al solubility of all Al–humic substance complexes exceeded that of gibbsite when the pH was higher than 4.2–4.6, indicating that Al is easily released into the soil solution even in the higher pH ranges ().
Plant root growth in the presence of synthetic Al–humic substance complexes
Results of the root lengths of burdock and barley grown in the presence of Al–humic substance complexes, Ca–humic substance complex and controls are shown in . Root length is shown as the maximum length of the primary root for burdock and as the total root length per one plant for barley. Root growth in the presence of the Al–humic substance complexes was evidently restricted compared with growth in the presence of the Ca–humic substance complex and the controls (P < 0.001). There was no significant difference in root growth among the three Al–humic substance complexes. As represented in , roots grown in the presence of the synthetic Al–humic substance complexes showed the typical morphology of roots injured by Al toxicity, for example, stubby appearance and brown tips (CitationFoy 1974). The root growth of barley was also restricted in the presence of the Al–humic substance complexes.
Figure 6 Root growth of burdock and barley cultured in perlite media containing synthetic Al–humic substance complexes (Al(4.0), Al(4.5) and Al(5.5)), Ca–humic substance complex (Ca) and perlite medium only (Cont.). Bars indicate standard deviation.
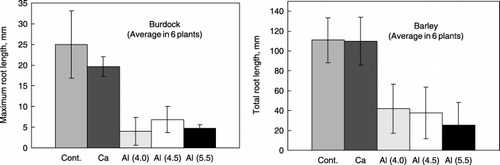
shows the pH values of the medium solution just after sampling of the plants. The pH values in the presence of the complexes ranged from 5.1 to 6.9, generally indicating low Al concentrations in the bulk solution. In the Al–humic substance systems, however, the level of Al in solution would be higher at the higher pH range compared with the system controlled by Al(OH)3 (). Thus, the concentrations of monomeric Al could be 10−6−10−5 mol L−1 at pH 5–5.5 in equilibrium condition; this level of Al is toxic to Al sensitive plants such as barley (CitationCameron et al. 1986). The unexpectedly high pH values (up to pH 6.9) observed in are attributable to the low buffering capacity resulting from the low concentration of the Al–humic substances (< 5%) in the culture medium.
Table 3 pH values of a solution of culture media just after plant sampling
DISCUSSION
The solid-state CPMAS 13C-NMR spectra () showed that the humic substances prepared in the present study concentrated aromatic C and carbonyl C from the original soil (Kawatabi A horizon). CitationHiradate et al. (2004) indicates that highly humified humic acids are rich in carbonyl C and aromatic C as indicated by the CPMAS 13C-NMR spectra. Therefore, it is likely that the humified fractions of the soil organic C were extracted and concentrated in the humic substances. The visible photoabsorption spectra () and melanic indices () also showed the presence of the A-type of humic acid with a high degree of humification in all synthetic Al–humic substance complexes. In contrast to the similarity of the C status among the complexes, the amounts and status of Al varied according to the pH during synthesis, as shown in the results of the selective dissolution analyses (extractions with pyrophosphate and sodium hydroxide-tetraborate) and the Al release rates using the acetate buffer solution (10−3 mol L−1, pH 3.5).
The solid-state 27Al-NMR analysis revealed the existence of the Al13 polymer in Complex (4.5) and Complex (5.5). CitationHiradate and Yamaguchi (2003) and CitationYamaguchi et al. (2004) reported that when Al13 came into contact with humic acids, it formed Al13–humic acid complexes in the solid phase and Al13 was gradually decomposed and converted to octahedral Al. Therefore, Al13 would not occur under natural soil conditions except in special cases, such as those observed in the organic horizons of a Spodosol (CitationHunter and Ross 1991). Because Al13 has a high positive charge (+7), Al13 precipitated with humus or negatively charged clay minerals is strongly fixed in the solid phase of the soils, and is hardly released (CitationHiradate and Yamaguchi 2003). In fact, Al13 was not detected in the soil solution and salt solution extracts of soils (CitationHiradate et al. 1998; CitationToma et al. 1999).
As observed in the Al release rate results (), monomeric Al ions were easily released from the Al–humic substance complexes using the dilute acetic buffer solution (10−3 mol L−1) adjusted to pH 3.5. As already noted, the amounts of Al released during the 60-min experiment corresponded to 3.2–13.7% of the total Al content of the Al–humic substance complexes, which is comparable to the rate of Al released to the total organically complexed Al of Kawatabi soil (approximately 10% of Alp). The trend in the Al release rates among the complexes is similar to the amounts of Al extracted using sodium hydroxide-tetraborate. The slow Al release rates from Complex (5.5) in spite of the high Al content and re-increase of the rates after 10 min () is attributable to the high content of polymer Al and Al13. Thus, monomeric Al and Al in the lower degree of polymerization are more important for the release of Al than the polymer Al and Al13.
The Al solubility of the Al–humic substance complexes derived from the equilibrium study were different from that of gibbsite, as observed for soils dominated by Al–humus complexes (CitationDahlgren and Ugolini 1989; CitationTakahashi et al. 1995; CitationTakahashi et al. 2003). This strongly indicates that Al solubility is largely controlled by the exchange reaction of the H+ and Al ions on the negatively charged sites on the humic substance. Thus, it is considered that Al is easily and rapidly released from the synthetic Al–humic substance complexes even when the pH slightly decreases and the ionic strength slightly increases. The average concentration of Al in the solutions of the Al–humic substance complexes/perlite mixture may be higher than those of soil minerals in the higher pH ranges (greater than pH values of 4.2–4.6).
The plant culture experiment showed that all synthetic Al–humic substance complexes similarly injured plant roots (). This injury resulted from Al toxicity as attested by the morphology of the roots (). These results are contradictory to the fact that the application of organic matter generally reduces Al toxicity. These complete opposite effects are likely to be because of the solubility of Al; Al–humus complexes of non-allophanic Andosols tend to easily and rapidly release Al. This instability of a portion of the Al–humus complexes was confirmed by the measurable reduction in sodium pyrophosphate-extractable Al (Alp) with a rather simple treatment, the liming of soils (CitationTakahashi et al. 2006a). In contrast, the application of organic matter to acid soils would generally reduce Al solubility because of the formation of more stable Al–organic matter complexes.
Complex (4.5) and Complex (5.5) contained Al13 (), which has been revealed to show a much stronger toxicity than monomeric Al to plant roots in solution cultures (CitationParker et al. 1989; CitationWagatsuma and Ezoe 1985; CitationWagatsuma and Kaneko 1987). However, plant roots grown in the presence of Complex (4.0), in which Al13 was not detected using the 27Al NMR analysis (), were similarly injured to those of the other Complexes (4.5 and 5.5). As mentioned earlier, the Al13 precipitated with humic materials is considered to be strongly fixed in the solid phase and seldom released into the solution phase (CitationHiradate and Yamaguchi 2003; CitationYamaguchi et al. 2004). Therefore, it is highly likely that Al13 did not participate in the toxicity observed in the present study.
The solution pH in the culture medium of all the synthetic Al–humic substance complexes was observed to be above 5.0 (pH 5.1–6.9, ). At pH 5–5.5, monomeric Al in the solution could be at levels of 10−5−10−6 mol L−1, which may show toxicity to plant roots. However, in the higher pH range (pH 6–7), the Al concentration would be very low in the bulk medium solutions. Therefore, it is likely that high Al dissolutions to toxic levels only occurred in the rhizosphere of this study. CitationHeim et al. (2003) found that Norway spruce (Picea abies L.) received Al injury in solid media that possessed a sufficiently high pH (>5) and no detectable soluble Al (CitationHeim et al. 2003). CitationGottlein et al. (1999) showed that the concentration of Al3+ significantly increased in the soil solution of the rhizosphere of oak seedlings (Quercus robur L.) using microlysimeters. These previous studies indicate that analyses of bulk soil and bulk soil solutions are only limited tools for estimating Al toxicity. We need to observe the mechanism of Al toxicity to plant roots on a microscale, for instance, by using microlysimeters, although we were not able to measure Al concentration in culture solutions because of insufficient amounts of the solutions. Because the Al–humic substance complexes show a higher Al solubility in the high pH range compared with that of gibbsite () and rapid Al release rates with the dilute acidic solution (), lowering pH and increasing ion strength in the rhizosphere will easily lead to dissolution of large amounts of Al.
Synthetic Al–humic substance complexes possessed very similar compositions of C and Al, optical spectra, and Al release and Al solubility properties to those of the soil horizons dominated by Al–humus complexes (CitationTakahashi et al. 1995). The Al–humic substance complexes synthesized at higher pHs (pH 4.5 and 5.5) contained Al13, whereas Al13 was not detected in the complex synthesized at pH 4.0. All Al–humic substance complexes equally injured burdock or barley roots. The results of this study indicate that, in soils dominated by Al–humus complexes, Al released from Al–humus is definitely toxic to plant roots as well as exchangeable Al adsorbed by the negatively charged sites on the minerals, although the contribution of Al13 to the Al toxicity would be negligible.
ACKNOWLEDGMENTS
This study was partly supported by a Grant for Environmental Research from the Sumitomo Foundation (No. 63046).
REFERENCES
- Food and Agriculture Organization, International Soil Reference and Information Centre (ISRIC), International Society of Soil Science (ISSS) . 1998 . World Reference Base for Soil Resources , Rome : Food and Agriculture Organization . 84, World Soil Resources Reports
- Nanzyo , M , Dahlgren , R and Shoji , S . 1993 . “ Chemical characteristics of volcanic ash soils ” . In Volcanic Ash Soils – Genesis, Properties and Utilization , Edited by: Shoji , S , Nanzyo , M and Dahlgren , RA . 145 – 187 . Amsterdam : Elsevier .
- The Fourth Committee for Soil Classification and Nomenclature, the Japanese Society of Pedology . 2002 . Unified Soil Classification System of Japan 2nd Approximation , Tokyo : Hakuyusha . (in Japanese)
- Saigusa , M , Shoji , S and Takahashi , T . 1980 . Plant root growth in acid Andosols from northeastern Japan: 2. Exchange acidity Y1as a realistic measure of aluminum toxicity potential . Soil Sci , 130 : 242 – 250 .
- Shoji , S , Saigusa , M and Takahashi , T . 1980 . Plant root growth in acid Andosols from northeastern Japan: 1. Soil properties and root growth of burdock, barley, and orchard grass . Soil Sci , 130 : 124 – 131 .
- Jardine , PM and Zelazny , LW . 1996 . “ Surface reactions of aqueous aluminum species ” . In The Environmental Chemistry of Aluminum , 2nd edn , Edited by: Sposito , G . 221 – 270 . Boca Raton : Lewis Publishers .
- Dahlgren , RA and Saigusa , M . 1994 . Aluminum release rates from allophanic and nonallophanic Andosols . Soil SciPlant Nutr , 40 : 125 – 136 .
- Takahashi , T , Fukuoka , T and Dahlgren , RA . 1995 . Aluminum solubility and release rates from soil horizons dominated by aluminum–humus complexes . Soil SciPlant Nutr , 41 : 119 – 131 .
- Takahashi , T and Dahlgren , RA . 1998 . Possible control of aluminum solubility by 1 M KCl treatment in some soils dominated by aluminum–humus complexes . Soil SciPlant Nutr , 44 : 43 – 51 .
- Takahashi , T , Sato , T , Sato , A and Nanzyo , M . 2003 . Relationship between 1 M KCl-extractable aluminum and pyrophosphate-extractable aluminum in A horizons of non-allophanic Andosols . Soil SciPlant Nutr , 49 : 729 – 733 .
- Wong , MTF and Swift , RS . 1995 . “ Amelioration of aluminum phytotoxicity with organic matter ” . In Plant Soil Interactions at Low pH , Edited by: Date , RA , Grundon , NJ , Rayment , GE and Probert , ME . 41 – 45 . Boston : Kluwer Academic Publishers .
- Furuya , H , Takahashi , T and Matsumoto , T . 1999 . Suppression of Fusarium solanif. sp. phaseoli on bean by aluminum in acid soils . Phytopathology , 89 : 47 – 52 .
- Mizuno , N , Yoshida , H , Nanzyo , M and Tadano , T . 1998 . Chemical characterization of conducive and suppressive soils for potato scab in Hokkaido, Japan . Soil SciPlant Nutr , 44 : 289 – 295 .
- Saigusa , M , Toma , M and Nanzyo , M . 1996 . Alleviation of subsoil acidity in nonallophanic Andosols by phosphogypsum application in topsoil . Soil SciPlant Nutr , 42 : 221 – 227 .
- Takahashi , T , Ikeda , Y , Nakamura , H and Nanzyo , M . 2006b . Efficiency of gypsum application to acid Andosols estimated using aluminum release rates and plant root growth . Soil SciPlant Nutr , 52 : 584 – 592 .
- Sunehez-Monedero , MA , Roig , A and Cegarra , J . 2002 . Effects of HCl-HF purification treatment on chemical composition and structure of humic acids . EurJSoil Sci , 53 : 375 – 381 .
- American Society of Agronomy and Soil Science Society of America . 1982 . Methods of Soil Analysis, Part 2Chemical and Microbiological Properties , 2nd edn , Madison : American Society of Agronomy, Inc. and Soil Science Society of America, Inc. .
- Higashi , T . 1983 . Characterization of Al/Fe–humus complexes in Dystrandepts through comparison with synthetic forms . Geoderma , 31 : 277 – 288 .
- Ito , T and Saigusa , M . 1996 . Characteristics of nonallophanic Andisols at Tohoku University Farm . BullExpFarm Tohoku Uni , 12 : 91 – 103 .
- Soil Survey Staff1999 Soil Taxonomy2nd edn Agriculture Handbook No.436 USDA-NRCS, Washington DC
- Honna , T , Yamamoto , S and Matsui , K . 1988 . A simple procedure to determine melanic index useful to separation of melanic and fulvic Andisols . Pedologist , 32 : 69 – 78 .
- Dahlgren , RA and Walker , WJ . 1993 . Aluminum release rates from selected Spodosol Bs horizons: Effect of pH and solid-phase aluminum pools . GeochimCosmochimActa , 57 : 57 – 66 .
- Rogeberg , EJS and Henriksen , A . 1985 . An automatic method for fractionation and determination of aluminum species in fresh-waters . Vatten , 41 : 48 – 53 .
- Hiradate , S and Yamaguchi , NU . 2003 . Chemical species of Al reacting with soil humic acids . JInorgBiochem , 97 : 26 – 31 .
- Cronan , CS , Walker , WJ and Bloom , PR . 1986 . Predicting aqueous aluminum concentrations in natural waters . Nature , 324 : 140 – 143 .
- Foy , CD . 1974 . “ Effects of aluminum on plant growth ” . In The Plant Root and Its Environment , Edited by: Foy , CD . 601 – 642 . Charlottesville : University Press of Virginia .
- Cameron , RC , Ritchie , GSP and Robson , AD . 1986 . Relative toxicities of inorganic aluminum complexes to barley . Soil SciSocAmJ , 50 : 1231 – 1236 .
- Hiradate , S , Nakadai , T , Shindo , H and Yoneyama , T . 2004 . Carbon source of humic substances in some Japanese volcanic ash soils determined by carbon stable isotopic ratio, δ13C . Geoderma , 119 : 133 – 141 .
- Yamaguchi , N , Hiradate , S , Mizoguchi , M and Miyazaki , T . 2004 . Disappearance of aluminum tridecamer from hydroxyaluminum solution in the presence of humic acid . Soil SciSocAmJ , 68 : 1838 – 1843 .
- Hunter , D and Ross , DS . 1991 . Evidence for a phytotoxic hydroxyl-aluminum polymer in organic horizons . Science , 251 : 1056 – 1058 .
- Hiradate , S , Taniguchi , S and Sakurai , K . 1998 . Aluminum speciation in aluminum silica solutions and potassium chloride extracts of acidic soils . Soil SciSocAmJ , 62 : 630 – 636 .
- Toma , M , Hiradate , S and Saigusa , M . 1999 . Chemical species of Al in a gypsum-treated Kitakami Andosol . Soil SciPlant Nutr , 45 : 279 – 285 .
- Dahlgren , RA and Ugolini , FC . 1989 . Formation and stability of imogolite in a tephritic Spodosol, Cascade Range, Washington, USA . GeochimCosmochimActa , 53 : 1897 – 1904 .
- Takahashi , T , Ikeda , Y , Fujita , K and Nanzyo , M . 2006a . Effect of liming on organically complexed aluminum of nonallophanic Andosols from northeastern Japan . Geoderma , 130 : 26 – 34 .
- Parker , DR , Kinraide , TB and Zelazny , LW . 1989 . On the phytotoxicity of polynuclear hydroxy–aluminum complexes . Soil SciSocAmJ , 53 : 789 – 796 .
- Wagatsuma , T and Ezoe , Y . 1985 . Effect of pH on ionic species of aluminum in medium and on aluminum toxicity under solution culture . Soil SciPlant Nutr , 31 : 547 – 561 .
- Wagatsuma , T and Kaneko , M . 1987 . High toxicity of hydroxyl-aluminum polymer ions to plant roots . Soil SciPlant Nutr , 33 : 57 – 67 .
- Heim , A , Brunner , I , Frossard , E and Luster , J . 2003 . Aluminum effects on Picea abiesat low solution concentrations . Soil SciSocAmJ , 67 : 895 – 898 .
- Göttlein , A , Heim , A and Matzner , E . 1999 . Mobilization of aluminium in the rhizosphere soil solution of growing tree roots in an acidic soil . Plant Soil , 211 : 41 – 49 .