Abstract
Laboratory experiments were conducted to examine the effect of charcoal addition on N2O emissions resulting from rewetting of air-dried soil. Rewetting the soil at 73% and 83% of the water-filled pore space (WFPS) caused a N2O emission peak 6 h after the rewetting, and the cumulative N2O emissions throughout the 120-h incubation period were 11 ± 1 and 13 ± 1 mg N m−2, respectively. However, rewetting at 64% WFPS did not cause detectable N2O emissions (−0.016 ± 0.082 mg N m−2), suggesting a severe sensitivity to soil moisture. When the soils were rewetted at 73% and 78% WFPS, the addition of charcoal to soil at 10 wt% supressed the N2O emissions by 89% . In contrast, the addition of the ash from the charcoal did not suppress the N2O emissions from soil rewetted at 73% WFPS. The addition of charcoal also significantly stimulated the N2O emissions from soil rewetted at 83% WFPS compared with the soil without charcoal addition (P < 0.01). Moreover, the addition of KCl and K2SO4 did not show a clear difference in the N2O emission pattern, although Cl− and SO2− 4, which were the major anions in the charcoal, had different effects on N2O-reducing activity. These results indicate that the suppression of N2O emissions by the addition of charcoal may not result in stimulation of the N2O-reducing activity in the soil because of changes in soil chemical properties.
INTRODUCTION
N2O is an important greenhouse gas produced in soil (CitationBouwman 1990). It has a destructive potential in the stratospheric ozone layer (CitationCrutzen 1981). Nitrification and denitrification are the most important biological processes in the production of N2O in soil. Denitrification is identically the sole process associated with N2O reduction (CitationZumft 1997).
In a previous study, we examined the effects of soil amendments (liming material, inorganic salts and charcoal) on the N2O-reducing activity of denitrifying communities (CitationYanai et al. ). We found that charcoal and its ash, which had a high content of alkali and inorganic salts (CitationNerome et al. 2005), seemed to promote the growth activity and N2O-reducing activity of denitrifying communities and that liming and anions affected these activities more than cations. These results suggest that N2O emissions from soil can be affected by certain soil amendments because of the modifying activity of N2O reduction, assuming that N2O emissions from soil through denitrification depend on the balance of the N2O-producing and N2O-reducing activity of denitrifying communities (CitationCavigelli and Robertson 2001). In fact, CitationInubushi et al. (1999) and CitationAzam and Müller (2003) observed stimulation of N2O emissions from soil by the addition of NaCl in a laboratory incubation study, and this result can be explained by the suppression of N2O-reducing activity by Cl− or Na+ (CitationYanai et al. ). In contrast, the effects of liming on N2O emissions from soil are inconsistent in field studies (CitationBorken and Brumme 1997; CitationButterbach-Bahl et al. 1997; CitationKlemedtsson et al. 1997; CitationMosier et al. 1998; CitationTokuda and Hayatsu 2004; CitationWang et al. 1997) and in laboratory incubation studies (CitationBorken et al. 2000; CitationClough et al. 2003; CitationClough et al. 2004; CitationKhalil et al. 2003).
Pulses of N2O emission have been observed in field studies following irrigation and precipitation events (e.g. CitationKusa et al. 2006; CitationRuser et al. 2001) and have been reproduced in laboratory incubation experiments as a rewetting of dry soil (CitationRudaz et al. 1991). CitationRudaz et al. (1991) and CitationRuser et al. (2006) investigated the contribution of nitrification and denitrification to the production of N2O emitted after rewetting using the C2H2 addition method and 15N tracing technique, respectively, and concluded that the N2O was mainly produced through denitrification. In the present study, to examine the relationship between enhancing the N2O-reducing activity of denitrifying communities and N2O emissions from soil, we examined the effects of charcoal and anion species on N2O emissions caused by the rewetting of air-dried soil in the laboratory.
MATERIALS AND METHODS
Soil samples and charcoal
The soil samples examined were the same as those used in our previous study (CitationYanai et al.). Soil sampling was conducted at the Field Museum Tsukui, the Field Science Center of Tokyo University of Agriculture and Technology, Tokyo, Japan. Soil samples were collected from a grassland field in which one side was planted with Sorghum bicolor (L.) Moench and the other with Sorghum sudanense (Piper) on April 2004 and March 2005, respectively. The soil is classified as Typic Hapludand, and the soil texture is loam to clay loam at a depth of 0–40 cm, with a granular structure (Kurokawa, pers. comm.). After collection, moist soil samples were passed through a 2-mm mesh sieve, and part of the sample was then air-dried. Selected physico-chemical properties of the soil samples are listed in . Soil pH (H2O) value was determined in a 1:2.5 air-dried soil (weight) to deionized water (volume) ratio. Total carbon and nitrogen contents of the soils were determined using the dry combustion method using a CN CORDER MT-700 (Yanaco, Kyoto, Japan). Water soluble organic C and NO− 3-N contents were determined using a TOC meter (TOC-VCSH, SHIMADZU Co. Ltd., Kyoto, Japan) and an ion chromatograph (LC-20AT, SHIMADZU Co. Ltd., Kyoto, Japan), respectively, in 1:10 extracts (air-dried soil to deionized water w/v) at 240 rpm for 30 min. The population density of denitrifiers in the air-dried soil samples was determined using the most probable number method in five replicates of 10-fold serial dilution (CitationTiedje 1994). Maximum water-holding capacity (MWHC) was determined using the Hilgard method. Particle density was determined using the pycnometer method (CitationBlake and Hartge 1986). Water-filled pore space (WFPS) was calculated as follows:
The charcoal, which was made from municipal biowaste, was provided by JFE Holdings. The physico-chemical properties of the charcoal are listed in CitationYanai et al. and its potential usefulness for cultivation was demonstrated by CitationNerome et al. (2005). Some selected physico-chemical properties of the charcoal and its ash, which was obtained by heating at 700°C for 4 h (as the test of weight loss-on-ignition; LOI), are listed in . Charcoal and ash pH (H2O) values were determined in a 1:5 air-dried material to deionized water ratio (w/v). The MWHC and particle density were determined as described above. Anion
Table 1 Selected physico-chemical properties of the air-dried soils examined in this study (oven-dry basis)
Table 2 Selected physico-chemical properties of charcoal and its ash examined in this study
Measurement of N2O emissions from soil after rewetting
To simulate the thin surface layer in arable fields, where the soil could be subjected to air-drying following continuous clear weather, 30 g of sieved air-dried soil was placed in a Petri dish (1.3 cm height and 8.5 cm diameter) without compaction. As a result, the thickness of the soil was approximately 0.8 cm. To simulate the condition of the soil during or immediately after precipitation, distilled water was added into the soil samples in the Petri dishes to more than 70% of their MWHC (equivalent to 64% of the water-filled pore space [WFPS]). Immediately after rewetting the soil sample, N2O emissions were periodically measured using the closed-chamber method (CitationHutchinson and Mosier 1981). A clear glass bell-jar (14 cm width, 26 cm height, 2.32 L) was used as a gas-tight chamber to monitor the concentration change in the headspace gas. The inlet and outlet of the bell-jar were sealed with a rubber stopper and a rubber septum, respectively, and the bottom part of the bell-jar was tightly attached with a ground glass-plate using a high vacuum-sealing compound (HIVAC-G, Shin-Etsu Chemical Co. Ltd., Tokyo, Japan). A pressure-controlling bent (CitationHutchinson and Mosier 1981) was installed at the rubber stopper in the inlet and gas samples were collected through the rubber septum installed at the outlet. After placing the chamber onto the soil sample, headspace gas was withdrawn five times at 2 or 8 min intervals, depending on the rate of concentration change. N2O concentration in the collected gas sample was analyzed using a gas chromatograph (GC-14A, SHIMADZU, Kyoto, Japan) equipped with an electron capture detector and a stainless steel column packed with Porapak-Q (80/100 mesh, 3 mm diameter, 2 m length). The column and detector temperatures were kept at 90°C and 330°C, respectively. Argon containing 5% CH4 was used as a carrier gas at a flow rate of 23 mL min−1. The N2O emission rate was calculated using the linear regression method (CitationHutchinson and Mosier 1981). After measurement of the N2O emission rate, the chamber was removed and the soil sample was left at room temperature (approximately 20–28°C) without a lid on the Petri dish. The water content was maintained during the incubation period by adding distilled water. N2O emissions were monitored until the first peak of N2O emissions disappeared.
As a preliminary experiment, we examined the effect of moisture content after rewetting on the N2O emissions from soil (TG2005). Distilled water was added into the soil samples at 17.0, 19.9 and 23.1 mL to adjust the rewetted condition to 70, 80 and 91% MWHC, equivalent to 64, 73 and 83% WFPS, respectively. This experiment was conducted in triplicate.
Effect of soil amendments on N2O emissions from soil resulting from rewetting
Based on the result of the preliminary experiment (), the moisture content after rewetting was adjusted to more than 73% WFPS in this study. First, to simulate precipitation in grassland amended with charcoal in the surface layer, 2 mm-sieved charcoal was mixed with soil (TG2004) in three of six Petri dishes at 10 wt% (equivalent to 13 vol%) before rewetting, and the N2O emissions were compared with the remaining three Petri dishes as the non-added control. The soil samples were added with distilled water to moisten the soil of the non-added control at 78% WFPS. Second, to test whether the effect of the charcoal addition on the N2O emission results from the stimulation of N2O-reducing activity by pH increase, the charcoal or its ash was mixed with soil (TG2005) in three of nine Petri dishes before rewetting, and three Petri dishes as the non-added control. The amounts of added charcoal and its ash were determined in order to set soil pH (H2O) at 6.0, and the rate of addition was 8.2 and 1.6 wt%, equivalent to 9.7 and 2.0 vol%, respectively. The soil samples were rewetted by adding distilled water, which was necessary to
Figure 1 Effect of rewetting on N2O emissions from soil (TG2005). An air-dried soil sample was rewetted using distilled water at 64 (×), 73 (⧫) and 83% (▪) of the water-filled pore space (WFPS) and incubated at room temperature. The values shown are the mean ± standard deviation of three replicates. The cumulative N2O emissions during the 120-h incubation period at a rewetting level of 64, 73 and 83% WFPS were −0.016 ± 0.082, 11 ± 1 and 13 ± 1 mg N m−2 (equivalent to −0.003 ± 0.03, 2.3 ± 0.3 and 2.8 ± 0.4 µg N g−1soil), respectively.
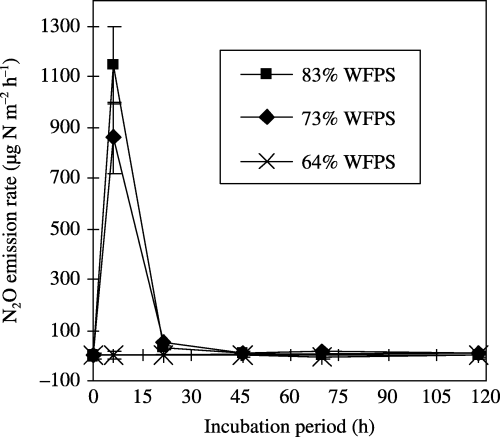
Calculation of the cumulative N2O emission and statistical analysis
The cumulative N2O emissions were estimated using the linear trapezoidal method, and the value was expressed as an arithmetic mean and standard deviation (SD). The level of significance of the treatments was examined using an unpaired t-test for TG2004 and by anova followed by Tukey's multiple comparison tests for TG2005 (P < 0.05). If one of the mean values of the triplicates appeared to lose normality (mean − 2SD < 0), the original data were log-transformed before comparison (CitationBland and Peacock 2002).
RESULTS
N2O emissions from soil after rewetting
N2O emissions were not detected after rewetting at 64% WFPS, but were detected after rewetting at 73% and 83% WFPS, and the cumulative N2O emissions throughout the 120-h incubation period at room temperature were −0.016 ± 0.082, 11 ± 1 and 13 ± 1 mg N2O-N m−2 (−0.003 ± 0.03, 2.3 ± 0.3 and 2.8 ± 0.4 µg N2O-N g−1soil), respectively (). Rewetting over 73% WFPS triggered N2O emissions, but there were no significant differences in the cumulative N2O emissions between soils rewetted at 73% and 83% WFPS (P = 0.180).
Effects of charcoal addition on N2O emissions after rewetting at 73% WFPS for TG2004
The highest N2O emission rate was observed 30 h after rewetting, and the values were 2620 ± 460 and 383 ± 74 µg N m−2 h−1 in the treatments without and with charcoal addition, respectively (). The addition of
Figure 2 Effect of charcoal addition on N2O emissions from soil (TG2004) rewetted at 78% of the water-filled pore space of the soil. The values shown are the mean ± standard deviation of three replicates. The cumulative N2O emissions during the 168-h incubation period for the non-added control and the 10 wt% charcoal addition were 105 ± 14 and 11.1 ± 2.4 mg N m−2 (equivalent to 19.9 ± 2.7 and 2.1 ± 0.5 µg N g−1 soil), respectively.
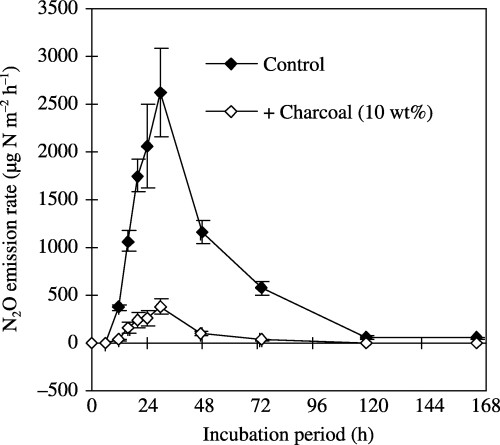
Table 3 Estimation of Cl− and load onto soil (TG2005) by the addition of charcoal, ash and K solution
Effects of liming (pH 6.0) with charcoal and its ash on N2O emissions after rewetting at 73% WFPS for TG2005
The highest N2O emission rate was observed at 12 h after rewetting in the non-added control and the ash-added soil (), but N2O emissions were kept at a low level in the charcoal-added soil throughout the observation period (72 h). The cumulative N2O emissions throughout the 72-h incubation period in the non-added control, ash-added and charcoal-added soils were 4.1 ± 1.9, 4.3 ± 1.2 and 0.8 ± 0.7 mg N m−2 (0.9 ± 0.4, 1.0 ± 0.3 and 0.2 ± 0.2 µg N g−1 soil), respectively. Charcoal addition decreased N2O emissions by 80% of the value of the non-added control (P < 0.05), whereas ash addition did not.
Effects of charcoal addition on N2O emissions after rewetting at 83% WFPS for TG2005
The N2O emission rate at 6 h after rewetting was lower in the 2 and 8.2 wt% charcoal added-soils than in the
Figure 3 Effect of liming by using charcoal and its ash on N2O emissions from soil (TG2005) rewetted at 73% of its water-filled pore space. The values shown are the mean ± standard deviation of three replicates. The cumulative N2O emissions during the 72-h incubation period for the non-added control, ash-amended soil and charcoal-amended soil were 4.1 ± 1.9, 4.3 ± 1.2 and 0.8 ± 0.7 mg N m−2 (equivalent to 0.9 ± 0.4, 1.0 ± 0.3 and 0.2 ± 0.2 µg N g−1soil), respectively.
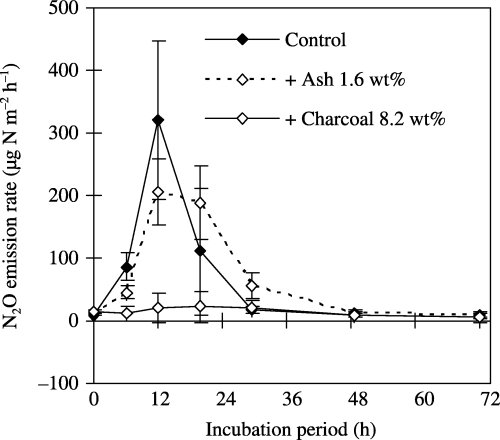
Figure 4 Effect of charcoal addition on N2O emissions from soil (TG2005) rewetted at 83% of its water-filled pore space. The values shown are the mean ± standard deviation of three replicates. The cumulative N2O emissions during the 72-h incubation period for the non-added control and the 2 and 8.2 wt% charcoal additions were 6.8 ± 0.9, 10.0 ± 0.8 and 10.3 ± 0.6 mg N m−2 (equivalent to 1.5 ± 0.2, 2.2 ± 0.2 and 2.4 ± 0.1 µg N g−1soil), respectively.
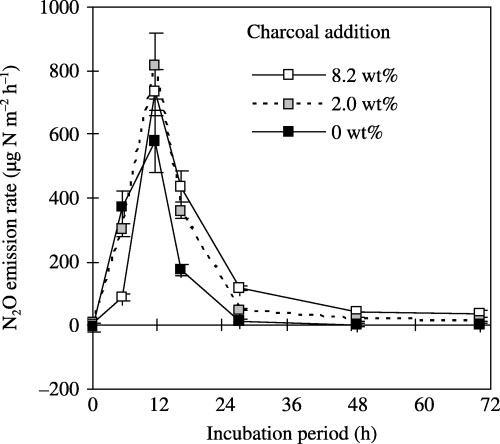
Effects of KCl and K2SO4 on N2O emissions after rewetting at 73% WFPS for TG2005
The highest N2O emission rate was observed 12 h after rewetting (). The mean N2O emission rate was higher in 10 mmol L−1 KCl than in 5 mmol L−1 K2SO4 and the non-added control, but considerable variability was observed in the KCl-added soil. The cumulative N2O emissions throughout the 72 h incubation in the non-added control, 10 mmol L−1 KCl and 5 mmol L−1 K2SO4 were 2.9 ± 0.6, 5.3 ± 6.1 and 4.4 ± 1.5 mg N m−2 (0.6 ± 0.1, 1.2 ± 1.3, 1.0 ± 0.3 µg N g−1 soil) with CV values of 21, 115 and 34% (17, 108 and 30%), respectively. There were no significant differences between the control and the 10 mmol L−1 KCl (P = 0.9986) or 5 mmol L−1 K2SO4 additions (P = 0.8559), or between the 10 mmol L−1 KCl and 5 mmol L−1 K2SO4 additions (P = 0.8794).
Figure 5 Effect of Cl− and of K salts on N2O emissions from soil (TG2005) rewetted at 73% of its water-filled pore space. An air-dried soil sample was rewetted using distilled water (control) or a K solution and incubated at room temperature. The values shown are the mean ± standard deviation of three replicates. The cumulative N2O emissions during the 72-h incubation period for the non-added control, the 10 mmol L−1 KCl-added soil and the 5 mmol L−1 K2SO4 added soil were 2.9 ± 0.6, 5.3 ± 6.1 and 4.4 ± 1.5 mg N m−2 (equivalent to 0.6 ± 0.1, 1.2 ± 1.3 and 1.0 ± 0.3 µg N g−1 soil), respectively.
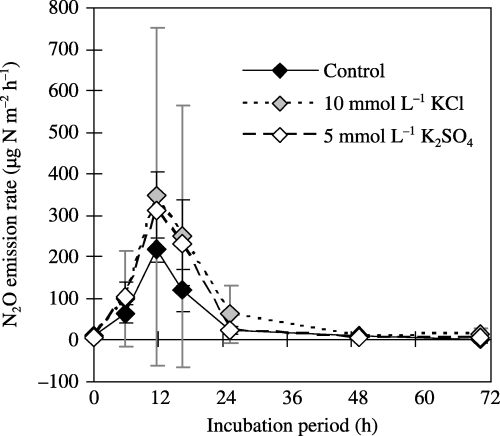
DISCUSSION
The present study demonstrated that rewetting of air-dried soil at 73% WFPS caused significant N2O emissions (), and the N2O emissions were suppressed by the addition of charcoal (,). This suppression of the N2O emissions was first considered to be a liming effect because charcoal has alkali () and it had the potential to increase the N2O-reducing activity of denitrifying communities (CitationCavigelli and Robertson 2000), which might cause a decrease in N2O emissions (CitationCavigelli and Robertson 2001). Therefore, liming resulting from the ash was expected to have a similar potential for promoting N2O-reducing activity to the charcoal itself. We checked the soil pH (1:2.5 ratio) after the observation of N2O emission from soils to which charcoal and its ash had been added and there were no significant differences between these amendments (5.7 ± 0.03 and 5.6 ± 0.01, respectively, P = 0.08), but these treatments were significantly different from the non-added control (4.9 ± 0.2, P < 0.01, n = 3). However, the addition of ash did not suppress N2O emissions (). Moreover, the suppressive effects of charcoal addition on N2O emissions were not observed when the soils were rewetted at 83% WFPS (). These results indicate that soil pH amendments, which are intended to stimulate the N2O-reducing activity, may not explain the suppression of the N2O emissions from soil rewetted at 73% WFPS (,). In addition, irrespective of the inhibitory effects of Cl− and the stimulatory effects of SO2− 4 on N2O-reducing activity of denitrifying communities (CitationYanai et al.), there were no clear differences in the N2O emissions when KCl and K2SO4 were added to the soils (). This finding could result from the use of concentrations (5 and 10 mmol L−1) that were too low to affect the denitrifying communities () because the effects of Cl− and were detected at more than 40 mmol L−1 in the liquid medium in our previous study (CitationYanai et al. ). Nevertheless, these results suggested that amelioration of the chemical properties of soil in order to stimulate the N2O-reducing activity may not be related to the suppression of the N2O emissions from soil rewetted at 73% WFPS (,).
Increases in N2O emission rates with increasing soil water contents have been reported from laboratory and field studies and have been attributed to increasing denitrifying activity induced by decreased O2 diffusion into the soil (CitationRuser et al. 2006 and references therein). In the present study, we observed a similar trend, namely, that N2O emissions increased with increases in the water content of soil by rewetting at 73% and 83% WFPS, whereas significant N2O emissions were not detected by rewetting at 64% WFPS (). This result suggests that a decrease in the moisture conditions from 83% to 73% WFPS did not affect the denitrifying communities, while a decrease from 73% to 64% WFPS may result in a significant decrease in the anoxic microsites, which results in the suppression of denitrification. Thus, undetectable N2O emissions from soil rewetted at 64% WFPS may not be the result of complete denitrification, including N2O reduction to N2, but, rather, to insufficient development of anoxic microsites in the soil to trigger denitrification. Possibly, this was caused by the soil sample TG2005, which had less denitrification activity because of a lower population density of denitrifiers, soil pH, and the amount of substrate compared with the soil sample TG2004 (). In addition, the decay of the N2O emission rate in the later incubation period after rewetting may be the completion of N2O production (stepwise reductions of NO− 3, NO− 2, and NO) rather than the kinetic equilibration of N2O production and reduction followed by N2 production. CitationRuser et al. (2006) observed few N2 emissions after rewetting, indicating a low or undetectable contribution of N2O-reducing activity in the later incubation period after rewetting. Therefore, the N2O-reducing activity of denitrifying communities may not significantly affect N2O emissions after rewetting of air-dried soil, suggesting that the suppressive effect of the charcoal addition on N2O emissions (,) might result from inhibition of N2O-producing activity of denitrifying communities.
Although there was no direct evidence to show a linkage between the addition of charcoal and the suppression of N2O emissions from soil (,), the added charcoal itself probably absorbed water and improved the aeration of the soil, leading to a suppression of N2O production (stepwise reduction of NO− 3, NO− 2 and NO) similar to the soil rewetted at 64% WFPS (). In fact, the charcoal examined was made up of porous particles, whereas the ash was nearly pulverized. Such differences in the size and structure possibly affect the water absorption capacity of these materials, and may consequently cause differences in the soil aeration, the denitrification process, and N2O emissions from soil, although the charcoal addition did not significantly affect the MWHC or the particle density (data not shown). Hence, the significant increases in N2O emissions by the addition of charcoal to soil rewetted at 83% WFPS () can be interpreted as an interaction between the insignificant improvement of the aeration of the soil and the stimulation of the N2O-producing activity resulting from neutralization (e.g. CitationCavigelli and Robertson 2000).
Charcoal was examined in this study because of its potential use for soil amendments in temperate regions (CitationNerome et al. 2005) and in the tropics (CitationGlaser et al. 2002; CitationYamato et al. 2006). Although any extrapolation of the findings from this short-term laboratory study to a long-term field scale should be conducted with caution, field applications of charcoal possibly suppress N2O emissions from arable soil, depending on the moisture or aeration conditions of the soil. In contrast, our understanding of the process of suppressing N2O emissions from soil by charcoal is still preliminary. Therefore, further studies are necessary to understand both the mechanisms and possible side-effects of charcoal addition to soil on the suppression of N2O emissions from soil, such as the activity of NO− 3 assimilation, NO− 2 accumulation in soil or NOx emissions from soil.
ACKNOWLEDGMENTS
The authors thank Dr Yuzo Kurokawa (Tokyo University of Agriculture and Technology) for providing soil samples and Mr Sumio Yamada (JFE Holdings) for providing the charcoal samples. The work described in this report was financially supported by a Sasakawa Scientific Research Grant from The Japan Science Society (16-315), the TUA&T 21 Century COE program (Evolution and Survival of Technology-based Civilization: Professor Masayuki Horio) and by the Japan Society for the Promotion of Science Research Fellowships for Young Scientists (17-6518).
REFERENCES
- Bouwman , AF . 1990 . “ Exchange of greenhouse gases between terrestrial ecosystems and the atmosphere ” . In Soils and the Greenhouse Effect , Edited by: Bouwman , AF . 61 – 127 . New York : John Wiley .
- Crutzen , PJ . 1981 . “ Atmospheric chemical processes of the oxides of nitrogen, including nitrous oxide ” . In Denitrification Nitrification and Atmospheric Nitrous Oxide , Edited by: Delwiche , CC . 17 – 44 . New York : John Wiley .
- Zumft , WG . 1997 . Cell biology and molecular basis of denitrification . MicrobiolMolBiolRev , 61 : 533 – 616 .
- YanaiY HatanoR OkazakiM ToyotaK.Chemical factors affecting the N2O-reducing activity of denitrifying communities – Analysis of the C2H2inhibition-based N2O production curve of soil
- Nerome , M , Toyota , K Islam , T-MD . 2005 . Suppression of bacterial wilt of tomato by incorporation of municipal biowaste charcoal into soil . Soil Microorganisms , 59 : 9 – 14 . (in Japanese with English summary)
- Cavigelli , MA and Robertson , GP . 2001 . Role of denitrifier diversity in rates of nitrous oxide consumption in a terrestrial ecosystem . Soil BiolBiochem , 33 : 297 – 310 .
- Inubushi , K , Barahona , MA and Yamakawa , K . 1999 . Effects of salts and moisture content on nitrous oxide emission and nitrogen dynamics in Yellow soil and Andosol in model experiments . BiolFertilSoils , 29 : 401 – 407 .
- Azam , F and Müller , C . 2003 . Effect of sodium chloride on denitrification in glucose amended soil treated with ammonium and nitrate nitrogen . JPlant NutrSoil Sci , 166 : 594 – 600 .
- Borken , W and Brumme , R . 1997 . Liming practice in temperate forest ecosystems and the effects on CO2, N2O and CH4fluxes . Soil Use Manage , 13 : 251 – 257 .
- Butterbach-Bahl , K , Gasche , R , Breuer , L and Papen , H . 1997 . Fluxes of NO and N2O from temperate forest soils: Impact of forest type, N deposition and of liming on the NO and N2O emissions . NutrCyclAgroecosys , 48 : 79 – 90 .
- Klemedtsson , L , Klemedtsson , AK , Moldan , F and Weslien , P . 1997 . Nitrous oxide emission from Swedish forest soils in relation to liming and simulated increased N-deposition . BiolFertilSoils , 25 : 290 – 295 .
- Mosier , AR , Delgado , JA and Keller , M . 1998 . Methane and nitrous oxide fluxes in an acid Oxisol in western Puerto Rico: Effects of tillage, liming and fertilization . Soil BiolBiochem , 30 : 2087 – 2098 .
- Tokuda , S and Hayatsu , M . 2004 . Nitrous oxide flux from a tea field amended with a large amount of nitrogen fertilizer and soil environmental factors controlling the flux . Soil SciPlant Nutr , 50 : 365 – 374 .
- Wang , YP , Meyer , CP , Galbally , IE and Smith , CJ . 1997 . Comparisons of field measurements of carbon dioxide and nitrous oxide fluxes with model simulations for a legume pasture in southeast Australia . JGeophysRes , 102 : 28 013 – 28 . 024
- Borken , W , Grundel , S and Beese , F . 2000 . Potential contribution of Lumbricus terrestrisL. to carbon dioxide, methane and nitrous oxide fluxes from a forest soil . BiolFertilSoils , 32 : 142 – 148 .
- Clough , TJ , Sherlock , RR and Kelliher , FM . 2003 . Can liming mitigate N2O fluxes from a urine-amended soil? . AustJSoil Res , 41 : 439 – 457 .
- Clough , TJ , Kelliher , FM , Sherlock , RR and Ford , CD . 2004 . Lime and soil moisture effects on nitrous oxide emissions from a urine patch . Soil SciSocAmJ , 68 : 1600 – 1609 .
- Khalil , MI , Van Cleemput , O , Rosenani , AB , Fauziah , CI and Shamshuddin , J . 2003 . Nitrous oxide formation potential of various humid tropic soils of Malaysia: A laboratory study . NutrCyclAgroecosys , 66 : 13 – 21 .
- Kusa , K , Hu , R , Sawamoto , T and Hatano , R . 2006 . Three years of nitrous oxide and nitric oxide emissions from silandic andosols cultivated with maize in Hokkaido, Japan . Soil SciPlant Nutr , 52 : 103 – 113 .
- Ruser , R , Flessa , H , Schilling , R , Beese , F and Munch , JC . 2001 . Effect of crop type-specific soil management and N fertilization on N2O emissions from a fine-loamy soil . NutrCyclAgroecosys , 59 : 177 – 191 .
- Rudaz , AO , Davidson , EA and Firestone , MK . 1991 . Sources of nitrous oxide production following wetting of dry soil . FEMS MicrobiolEcol , 85 : 117 – 124 .
- Ruser , R , Flessa , H , Russow , R , Schmidt , G , Buegger , F and Munch , JC . 2006 . Emission of N2O, N2and CO2from soil fertilized with nitrate: Effect of compaction, soil moisture and rewetting . Soil BiolBiochem , 38 : 263 – 274 .
- Tiedje , JM . 1994 . “ Denitrifiers ” . In Microbiological and Biochemical Properties , Edited by: Weaver , RD , Angle , JS and Bottomley , PS . 245 – 267 . Madison : Soil Science Society of America .
- Blake , GR and Hartge , KH . 1986 . “ Particle density ” . In Physical and Mineralogical Methods , Edited by: Klute , A . 377 – 382 . Madison : Soil Science Society of America .
- Hutchinson , GL and Mosier , AR . 1981 . Improved soil cover method for field measurement of nitrous oxide fluxes . Soil SciSocAmJ , 45 : 311 – 316 .
- Bland , M and Peacock , J . 2002 . “ Normal distribution ” . In Statistical Questions in Evidence-based Medicine , Edited by: Adachi , K . 79 – 85 . Tokyo : Shinoharashinsha Press . (in Japanese)
- Cavigelli , MA and Robertson , GP . 2000 . The functional significance of denitrifier community composition in a terrestrial ecosystem . Ecology , 81 : 1402 – 1414 .
- Glaser , B , Lehmann , J and Zech , W . 2002 . Ameliorating physical and chemical properties of highly weathered soils in the tropics with charcoal – A review . BiolFertilSoils , 35 : 219 – 230 .
- Yamato , M , Okimori , Y , Wibowo , IF , Anshori , S and Ogawa , M . 2006 . Effects of the application of charred bark of Acacia mangiumon the yield of maize, cowpea and peanut, and soil chemical properties in South Sumatra, Indonesia . Soil SciPlant Nutr , 52 : 489 – 495 .