Abstract
To investigate a soil's decolorization capacity for wastewater treatment, three types of soil (an Andisol, a sandy soil and a red soil) were compared with activated carbon and charcoal in their respective adsorption and recovery capacities. Sandy soil and red soil showed negative decolorization rates for sewage plant effluent. When 10 g of Andisol was applied to decolorize 30 mL of sewage plant effluent or livestock wastewater diluted fivefold and 20-fold, its decolorization rates reached 51.8%, 59.9% and 66.6%, respectively. The decolorization capacity of activated carbon and charcoal varied greatly because of their different original sources and production processes. An analysis of pore size distribution of activated carbons and charcoal showed that their decolorization capacity was positively related to their respective outside pore surface area and total mesopore volume. The result of a recovery experiment showed that activated carbon had a quick desorption for the adsorbed colored substances, which was hardly influenced when incubation time was increased from 7 days to 14 days. In contrast, the recovery rates for Andisol, zeolite and charcoal could be enhanced by increasing the incubation time from 7 days to 14 days or by decreasing the concentration of colored substances. During the continuous operation of Andisol-based decolorization systems, a column (100 cm3) filled with Andisol (84 g) and activated carbon (6 g) maintained a decolorization rate of more than 60% for 20 days for 20-fold diluted livestock wastewater with an absorbance of 0.8 at a wavelength of 406 nm at a hydraulic loading rate of 50 mL soil 100 cm−3 day−1.
INTRODUCTION
Many countries have strict regulations concerning the release of wastewater, which require that wastewater discharges should not only avoid environmental impacts but should also, where possible, be treated and recycled. In Japan, owing to the many effective wastewater treatment technologies available, most of the pollutants in the wastewater, especially biodegradable pollutants, can be controlled in accordance with government regulations. However, most of the non-biodegradable components of wastewater, such as the colored substances found in sewage and livestock wastewater, cannot be treated in an effective way and have caused visual pollution and hindered the recycling of treated water (CitationMori et al. 1997). There is an urgent need to develop new technologies to decolorize wastewater in an effective and economical way to promote environmental health and ensure efficient water recycling.
Adsorption is one technique that has been successfully used for the effective removal of non-biodegradable pollutants. The first step in establishing an efficient adsorption process is to find adsorbents with high selectivity, high capacity and long life. These need to be available in large quantities and have to be affordable (CitationFigueiredo et al. 2000). Activated carbon is one of the most well-known adsorbents, but because of its high price, research has also been directed towards alternative adsorbents, including the use of natural materials, such as soil, charcoal and bamboo charcoal (CitationDiez et al. 1999; CitationYatagai et al. 1995). It has been reported that, when applied to livestock wastewater with an absorbance of 0.201 at a wavelength of 410 nm, Andisol showed a decolorization rate of more than 50% over 60 days (CitationMori and Sakimoto 1998). The main mechanism for an Andisol to act as an adsorbent appears to be its high content of organic matter, which has a great affinity with pollutants, such as humic and fulvic acids. Furthermore, its clay-reactive sites, such as the Al and Fe hydroxide groups, can also be involved in fixing organic pollutants onto the soil matrix (CitationMora and Canales 1995).
In practical wastewater treatment systems, aside from the above-mentioned characteristics of the adsorbents, other factors, such as adsorbate–adsorbent chemical relations and treatment conditions, might also influence the adsorption kinetics (CitationFigueiredo et al. 2000), and we need to establish a long-life decolorization technique without the necessity for frequent changes of the adsorbents. In this study, we conducted a series of experiments to compare the characteristics of soils and other adsorbents in decolorizing wastewater, to examine the adsorption and recovery balance for the different adsorbents, and to investigate the influence of other factors on the treatment performance of actual soil-based decolorization systems.
MATERIALS AND METHODS
Wastewater and adsorbents
In this study, two types of wastewater with different colored substance concentrations were used. The first type of wastewater was effluent from a local sewage plant. When measured with a spectrophotometer (JASCO, V-530, Tokyo, Japan) at a wavelength of 406 nm, it showed an absorbance of 0.02. The other type was livestock wastewater from a local dairy farm without any pretreatment, which showed an absorbance of approximately 2.85. When diluted fivefold, 20-fold and 50-fold, it showed absorbances of 1.91, 0.80 and 0.45, respectively. Various wavelengths have been proposed for measuring the color of humic water using a spectrophotometer
Table 1 Soil classification, pH, particle size distribution, total carbon (TC), total nitrogen (TN), effective cation exchange capacity (eCEC) of Andisol, sandy soil and red soil used in the experiment
Three types of soil (Andisol, sandy soil and red soil), zeolite (1–3 mm), nine types of activated carbon (AC) and wood charcoal were tested. Andisol was collected from the top surface soil of Mount Sanbe of Shimane prefecture, Japan. Sandy soil (Inceptisol), a decomposed granite soil, was collected from the sub-surface mountain soil of Inbe, Shimane Prefecture, and is commercially available in Japan (Araya, Shimane, Japan). Red soil (Ultisol) was collected from sub-surface mountain soil in Matsue city, Japan. The physical and chemical properties of the tested soils are shown in . The nine ACs were powdered activated carbon with a peak pore diameter of 20 Å. They were obtained from two companies (EnviroChemicals, Osaka, Japan and Ajinomoto Fine-techno, Tokyo, Japan) with commercial names, Shirasagi M, MD5, E-200 and F-17W, H-10, HJA, F-17W (pH adjusted), H-10 (pH adjusted) and HJA (pH adjusted). The wood charcoal was in the shape of chips and was obtained from Sumihachi Izumo-doken, Izumo, Japan. The respective surface area pore size distribution characteristics are shown in .
Adsorption experiment
Different amounts of adsorbent samples based on dry weight (5, 20, 50, 100, 150 and 200 mg for AC and charcoal, and 50, 100, 500, 1,000, 5,000 and 10,000 mg for soils and zeolite) were put into 50-mL centrifuge tubes and 30 mL of wastewater was added to the tubes, which were shaken for 1 h on a horizontal shaker at 150 rpm at a temperature of 20°C. The tubes were
Table 2 pH value, original source, total surface area of micropores (A1), total outside surface area of micropores (A2), total surface area of mesopores (Ap), total volume of micropores (Vp) and mesopores (Vp), peak of the diameter of micropore distribution (Dp) and peak of the radius of mesopore distribution (Rp) of some of the activated carbon and charcoal used in the experiment
Table 3 Names, composition material, mixing percentage and bulk density of the columns in the recovery experiment
Recovery experiment
Seven columns with a volume of 100 cm3 were filled with mixtures of different adsorbents (). The experiment was conducted over three recovery periods (7-day, 3-day and 14-day). For the 7-day and 14-day recovery periods, Process A, including three stages, was adopted (). First, the columns were filled with 10-fold-diluted livestock wastewater at a hydraulic loading rate (HLR) of 100 mL column−1 day−1 for 48 h. Second, the columns were filled with fivefold-diluted wastewater and kept at room temperature for 2 days until they were saturated with colored substances. Third, they were incubated at a temperature of 30°C for 7 days or 14 days. For the 3-day recovery period, Process B was adopted, which omitted the saturation stage (). Sampling of water was conducted twice (after 24 h and 48 h) at the first stage and once after being saturated with colored substances.
The recovery rate of the adsorbents was calculated as:
Continuous column experiment
Eight columns with a volume of 100 cm3 were filled with Andisol or zeolite as the main adsorbents and mixed with AC or charcoal in different ratios (). Two of these columns were filled with hexadecyltrimethyl ammonium (HDTMA) treated Andisol and zeolite at concentrations similar to their respective cation exchange capacity (CEC) for 24 h. The concentration of HDTMA for the Andisol treatment was 10 cmol kg−1 and for zeolite was 100 cmol kg−1, as HDTMA was expected to increase the sorption of non-ionic organic compounds in the adsorbents by partition interactions with the HDTMA-derived organic phase (CitationJaynes and Boyd 1991). Column AC30′ was always applied at twice the HLR of the other seven columns. To check the aeration effect on the systems’ decolorization performance, aeration at an amount of 1,000 mL min−1 column−1 was applied to Char15 and Char30 from 21 September to 4 October
2005. The experimental period and treatment conditions are listed in .Laboratory analysis
The air-dried soil samples were ground and passed through a 2-mm sieve. Soil pH was measured using the glass-electrode method (CitationJapan Soil Standard Analysis and Measurement Method Committee 2003a);
Table 4 Names, composition material, mixing percentage and bulk density of the columns in the continuous column experiment
RESULTS AND DISCUSSION
Decolorization capacity of the tested adsorbents in the adsorption experiment
shows the decolorization rates of the different adsorbents when added to sewage plant effluent. At an amount of 50 mg per 30 mL, the decolorization rate of the Andisol was 11.7%, while the sandy soil and red soil showed a negative effect. In contrast, different ACs showed considerable variation when applied at an amount of 5 mg per 30 mL. Shirasagi M attained a decolorization rate as high as 78.8%, while E-200 only removed 4.7% of the colored substances in the wastewater. When the amount of Andisol was increased to 10,000 mg per 30 mL, its decolorization rate increased to 51.8%. At 200 mg per 30 mL, five types of AC, Shirasagi M, MD5, E-200, F-17W and F-17W (pH-adjusted), achieved decolorization rates of more than 90%, but the other four ACs only removed 40% of the colored substances. At the same treatment amount, the decolorization rate for charcoal reached 46.1%, which was comparable with some of the ACs.
Table 5 Experimental period, time, applied wastewater, hydraulic loading rate (HLR) and aeration conditions during the continuous column experiment period
Figure 2 Effect of the amount of adsorbent on the decolorization rates of sewage plant effluent. (a) Decolorization rates by soil and (b) decolorization rates by activated carbon and charcoal.
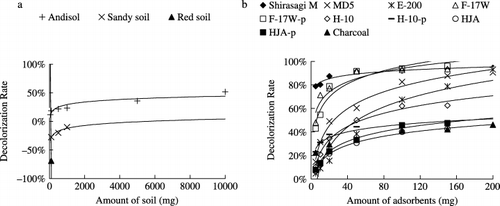
For the decolorization of livestock wastewater, at an amount of 50 mg per 30 mL, the Andisol had removal rates of 3.8, 22.4 and 19.7%, respectively, for fivefold, 20-fold and 50-fold diluted wastewater. When the amount increased to 10,000 mg per 30 mL, the decolorization rates increased to 59.9, 66.6 and 57.8%, respectively (). Further dilution of wastewater from 20-fold to 50-fold did not increase the decolorization rates of the Andisol, which suggests that the concentration of colored substances at 20-fold dilution was almost at the threshold for Andisol. In this study, the decolorization rate was 27.7% for Andisol at an amount of 500 mg per 30 mL to treat wastewater with an absorbance of 0.45 at 406 nm. CitationMori and Sakimoto (1998) have reported that some Andisols are capable of a decolorization rate as high as 76.8% at 500 mg per 10 mL for livestock wastewater with an absorbance of 0.202 at 410 nm. Zeolite showed decolorization rates of 5.3%, 11.4% and 13.7%, respectively for fivefold, 20-fold and 50-fold diluted livestock wastewater at an amount of 50 mg. When the amount of zeolite increased to 10 g, the decolorization rates also increased to 34.5%, 46.8% and 49.7%, respectively. The colored substances removal capacity of zeolite might come from its unique structure because zeolite are naturally occurring aluminosilicate minerals with 3-D structures based on SiO4− 4 and AlO4− 4 polyhedra. These polyhedra are linked by their corners to form internal cavities in which molecules of various sizes can be trapped. Although the surface of zeolite is negatively charged in the entire pH region, the porous zeolite is host to water molecules and ions of potassium and calcium as well as other positively charged ions that can attract anionic humic molecules from the solution (http://www.lenntech.com/zeolites-structure-types.htm).
For the decolorization of fivefold-diluted livestock wastewater, all the ACs and charcoal showed low decolorization rates ranging from 1.8% to 6.9% at a low amount of 5 mg per 30 mL. With an increase in the adsorbent amount, the decolorization rates increased, although there were big differences between them (). The rate for Shirasagi M increased the fastest and reached 78.1% at 200 mg per 30 mL, followed by MD5 (58.9%), E-200 (31.5%) and charcoal (11.8%). The further dilution of wastewater to 20-fold increased the decolorization rates of all the adsorbents, even at the small amount of 5 mg per 30 mL (). E-200 and
Figure 3 The effect of the amount of adsorbent on the decolorization rate of livestock wastewater diluted by different amounts. (a) Decolorization rates by Andisol and zeolite for fivefold, 20-fold and 50-fold diluted wastewater, (b) decolorization rates by activated carbons (ACs) and charcoal for 5-fold diluted wastewater, (c) decolorization rates by ACs and charcoal for 20-fold diluted wastewater and (d) decolorization rates by ACs and charcoal for 50-fold diluted wastewater. Andi, andisol; zeo, zeolite.
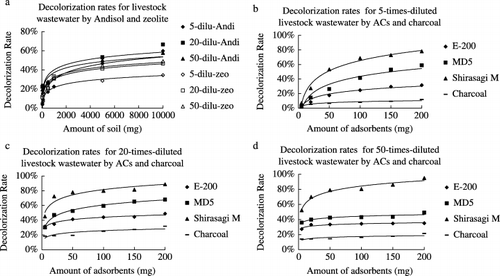
Decolorization capacities of these four types of adsorbents for sewage and livestock wastewater treatment were in the order Shirasagi M > MD5 > E-200 > charcoal. The analysis of pore size distribution () showed that this was positively correlated with the total mesopore volume and specific micropore outside surface area of the adsorbents, which was in agreement with the results reported by CitationMitsuyoshi et al. (1995). As the colored substances in the wastewater are mainly composed of large and non-degradable compounds (CitationNavia et al. 2005), the macropores and mesopores can serve as avenues for the rapid diffusion into the micropores where they are adsorbed. Shirasagi M, F-17W and F-17W (pH-adjusted) are made from wood, so they have well-developed pore size systems and achieved much higher decolorization rates than the other ACs. Andisol could also be a competitive adsorption material for retaining large non-biodegradable molecules because of its high specific area between 310 and 672 m2 g−1 (CitationDiez et al. 1999) and its high organic carbon content, which is able to act as a nanoporous sorbent of organic pollutants (CitationPignatello 1998).
Recovery capacity of the tested adsorbents in the recovery experiment
,c shows that after 7 days incubation, the decolorization capacities of the Andisol, Andisol plus charcoal (Andi+Char) and Zeolite columns decreased to less than 10% after three cycles of saturation and incubation, whereas the sand plus Shirasagi M (San+Shira-M) and Andisol plus Shirasagi M (Andi+Shira-M) columns were able to maintain stable decolorization rates of approximately 40% even after three experimental runs. Shirasagi M might have a high and quick decomposition capacity for the adsorbed colored substances on the surface as well as in the pores. The columns of red soil plus Shirasagi M (Red+Shira-M) and Andisol plus MD5 (Andi+MD5) decreased their decolorization rates to approximately 20% after three running cycles. The recovery rates of the columns defined by Eq. Equation2 also showed
Figure 4 Effect of incubation time on the recovery rates of Andisol-based and zeolite-based systems during the recovery experimental period. (a) Decolorization rates by andisol (Andi), andisol plus charcoal (Andi+Char) and Zeolite, (b) recovery rates by Andi, Andi+Char and Zeolite, (c) decolorization rates by sandy soil plus Shirasagi M (San+Shira-M), red soil plus Shirasagi M (Red+Shira-M), andisol plus Shirasagi M (Andi+Shira-M) and Andisol+MD5 and (d) recovery rates by San+Shira-M, Red+Shira-M, Andi+Shira-M and Andisol+MD5.
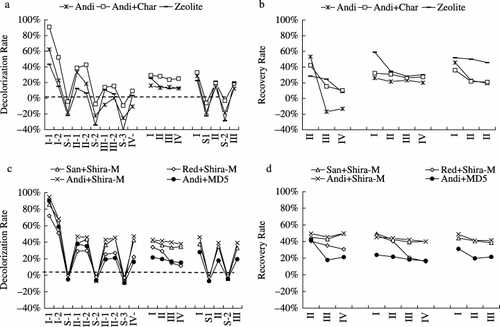
After 3 days of incubation without saturation, the columns of Andisol, Andi+Char and Zeolite showed higher recovery rates than after the 7-day incubation. However, for columns filled up with soil and AC, the shortening of incubation time and the omission of the saturation stage did not have much influence on their recovery rate, which confirmed that AC had a relatively faster decomposition speed for the entrapped colored substances and could maintain a stable decolorization capacity. Red soil was clogged during this period, which probably resulted from the clay dispersion caused by the high sodium content of the wastewater (approximately 105 mg L−1 for 10-fold-diluted water), as the clay content in red soil was as high as 40% ().
The increase in incubation time to 14 days also increased the recovery rates of the Andisol, Andi+Char and Zeolite columns. CitationTanner et al. (1995) reported that in a wetland designed for livestock wastewater treatment, the colored substances concentration in the outflow showed a gradual decline with increasing hydraulic retention time. This suggests that we can either increase incubation time or decrease colored substances concentration to maintain the sustainability of the decolorization capacity of these three columns. However, an increase in incubation time did not have much influence on the decomposition rates of the ACs, which seemed to have a limit to their decolorization capacity and recovery rate that was correlated with their own characteristics, such as surface area, pore volume and pore size distribution. The results of the recovery experiment indicate that the decolorization capacity of AC could be recovered within 7 days with saturation or 3 days without saturation. As a proportion of the adsorbed colored or other substances from wastewater was probably fixed into the pores of the adsorbents and hardly ever released, the recovery rate of the adsorbents could not fully return to the original level ().
Figure 5 Effect of colored substance concentration, hydraulic loading rate (HLR) and aeration on the decolorization rates of Andisol-based and zeolite-based systems during the continuous column experiment period. Column AC30′ was at double the HLR during Period 1 and 2. AC, activated carbon; Andi, andisol; Char, charcoal; HDTMA, hexadecyltrimethyl ammonium; Zeo, zeolite.
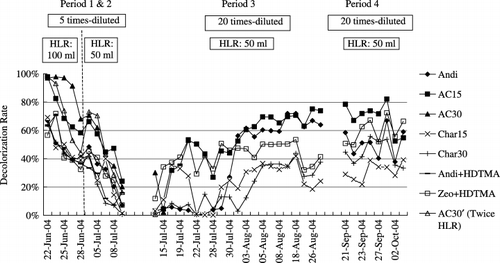
Performance of soil-based decolorization systems in the continuous column experiment
The column experiment started with fivefold-diluted livestock wastewater from 22 to 28 June 2005 at a HLR of 100 mL column−1 day−1. Decolorization rates for all the columns decreased by 26.2–53.8% after 1 week, except for Column AC30, which still maintained a decolorization rate as high as 70% after 1 week. This could have resulted from the high content of Shirasagi M (). However, after 2 weeks’ operation, AC30 was gradually saturated with the adsorbed colored substances and showed almost the same decolorization rate as that of AC15. As column AC30′ was applied at twice the HLR of the other columns, its decolorization rate decreased by 81.6% after 1 week of running. The columns of Char30 and Char15 were nearly 30% lower than AC30 during this period. An increase in the amount of charcoal did not show much difference to the decolorization effect. The HDTMA treatment showed no clear effect with Andisol, but Zeo+HDTMA showed a higher decolorization rate than Char30 and Char15. This suggests that treatment with a high concentration of HDTMA might enhance colored substances adsorption. In period 2, from 1 to 12 July 2005, HLR was adjusted to 50 mL column−1 day−1, and all the columns’ decolorization rates still kept decreasing to 0.6–24.4%. It seems that the systems were saturated with the high concentration of colored substances and could not recover their adsorption capacity under the treatment conditions of periods 1 and 2, even for AC30 filled with twice the amount of Shirasagi M.
Livestock wastewater diluted 20-fold was applied to the systems after 14 July 2005, and columns of AC30, Andi+HDTMA and AC30′ were stopped during period 3. The low HDTMA concentration treatment to Andisol showed no clear effect during Periods 1 and 2. In the case of AC30 and AC30′, twice the amount of Shirasagi did not have much effect on colored substances removal when the system was supplied with high-concentrated wastewater or at a high HLR. The other five columns all showed increasing decolorization rates at the beginning of this period and then remained stable from 2 to 22 August 2005. The average decolorization rates during the stable period were as follows: column AC15 showed the highest removal rate of 68.0%, followed by Andi (63.4%), Zeo+HDTMA (45.0%), Char30 (30.8%) and Char15 (30.6%). This shows that the columns could continuously remove colored substances at suitable HLR and colored substances concentrations, even after they were almost saturated at the beginning of period 3. Thus, under suitable conditions, soil-based systems can maintain a sustainable decolorization capacity even with only Andisol as an adsorbent. During period 4, from 21 September to 4 October 2005, columns of Char15 and Char30 were treated with aeration at a rate of 1,000 mL min−1 column−1, but this did not have any obvious effect in enhancing the decolorization rates. The effects of aeration on decolorization still require further research to reach any conclusions. Meanwhile, the decolorization rates for Andisol showed a gradual decline, while the rate for Zeo+HDTMA increased during this period. The adsorption sites on the surface of Andisol might get saturated with adsorbed colored substances after 3 months of operation. However, with time passing, the adsorption of NH+ 4-N from wastewater by zeolite with its high eCEC might have reversed the charge on the external surface from negative to positive; thus, imparting an affinity of the zeolite for negatively charged humic substances in the wastewater (CitationLi et al. 1998). In addition, organic matter accumulated in the zeolite surface might have also imparted affinity to colored substances.
Conclusions
Different materials showed different adsorption and recovery capacities in decolorizing sewage plant effluent and livestock wastewater. Andisol was a good adsorbent for colored substances removal because of its high organic content and specific surface area. Although ACs showed high decolorization capacities, their efficiency varied widely because they were made from different source materials and by different activation processes. The recovery rate for natural materials, such as Andisol, zeolite and charcoal, could be enhanced by increasing incubation time or decreasing the original colored substances concentration of the wastewater. The decolorization system based on Andisol+AC was effective and sustainable under the right conditions. However, for practical applications, other factors (such as HLR and aeration) still need further study to establish a real-life treatment system because they were found to influence the decolorization effect to a considerable degree.
ACKNOWLEDGMENT
The authors would like to express their deep gratitude to the Ministry of Education, Science, Sport and Culture of Japan for financial assistance for this study.
REFERENCES
- Mori , T , Sakimoto , M , Mori , T and Sakai , T . 1997 . Decolorization of wastewater from a livestock barn using Andosols . AnimSciTechnol , 68 ( 10 ) : 940 – 947 .
- Figueiredo , SA , Boaventura , RA and Loureiro , JM . 2000 . Color removal with natural adsorbents: modelling, simulation and experimental . SepPurifTechnol , 20 : 129 – 141 .
- Diez , MC , Mora , ML and Videla , S . 1999 . Adsorption of phenolic compounds and color from bleached Kraft mill effluent using allophonic compounds . Water Res , 33 ( 1 ) : 125 – 130 .
- Yatagai , M , Ito , R , Ohira , T and Oba , K . 1995 . Effect of charcoal on purification of wastewater . Mokuzai Gakkaishi , 41 ( 4 ) : 425 – 432 . (in Japanese)
- Mori , T and Sakimoto , M . 1998 . Decolorization and nitrogen removal of wastewater from a livestock barn using an Andosol-column decolorizer with Italian ryegrass cultivation . Osaka Agricultural Forestry Research Center , 34 : 25 – 28 . (in Japanese)
- Mora , ML and Canales , J . 1995 . Interactions of humic substances with allophatic compounds . CommunSoil SciPlant Anal , 26 ( 17/18 ) : 2805 – 2817 .
- Hautala , K , Peuravuori , J and Pihlaja , K . 2000 . Measurement of aquatic humus content by spectroscopic analysis . WatRes , 34 ( 1 ) : 246 – 258 .
- Jaynes , WF and Boyd , SA . 1991 . Clay mineral type and organic compound sorption by HDTMA-exchanged clays . Soil SciSocAmJ , 55 : 43 – 48 .
- Japan Soil Standard Analysis and Measurement Method Committee . 2003a . Soil Standard Analysis and Measurement Method (Dojou Hyojun Bunseki Sokutei Hou) , 6th edn , Edited by: Onikura , Y . 70 – 71 . Tokyo : Hakuyusha . pH. (in Japanese)
- Japan Soil Standard Analysis and Measurement Method Committee . 2003b . “ Particle size distribution ” . In Soil Standard Analysis and Measurement Method (Dojou Hyojun Bunseki Sokutei Hou) , 6th edn , Edited by: Onikura , Y . 14 – 22 . Tokyo : Hakuyusha . (in Japanese)
- Japan Soil Standard Analysis and Measurement Method Committee . 2003c . “ Organic carbon ” . In Soil Standard Analysis and Measurement Method (Dojou Hyojun Bunseki Sokutei Hou) , 6th edn , Edited by: Onikura , Y . 77 – 85 . Tokyo : Hakuyusha . (in Japanese)
- Japan Soil Standard Analysis and Measurement Method Committee . 2003d . “ Total nitrogen ” . In Soil Standard Analysis and Measurement Method (Dojou Hyojun Bunseki Sokutei Hou) , 6th edn , Edited by: Onikura , Y . 104 Tokyo : Hakuyusha . (in Japanese)
- McLean , EO . 1965 . “ Aluminium ” . In Methods of Soil Analysis , Edited by: Black , CA . 978 – 998 . Madison : ASA SSSA .
- Thomas , GW . 1982 . “ Exchangeable cations ” . In Methods of Soil Analysis , Edited by: Page , AL . 159 – 165 . Madison : ASA SSSA .
- Mitsuyoshi , Y , Ran , I , Tatsuro , O and Kihachiro , O . 1995 . Effect of charcoal on purification of wastewater . Mokuzai Gakkaishi , 41 ( 4 ) : 425 – 432 . (in Japanese)
- Navia , R , Fuentes , B , Lober , KE , Mora , ML and Diez , MC . 2005 . In-series columns adsorption performance of Kraft mill wastewater pollutants onto volcanic soil . Chemosphere , 60 : 870 – 878 .
- Pignatello , JJ . 1998 . Soil organic matter as a nanoporous sorbent of organic pollutants . AdvColloid Interface Sci , 76/77 : 445 – 467 .
- Tanner , CC , Clayton , JS and Upsdell , MP . 1995 . Effect of loading rate and planting on treatment of dairy farm wastewaters in constructed wetlands. I. Removal of oxygen demand, suspended solids and faecal coliforms . Water Res , 29 ( 1 ) : 17 – 26 .
- Li , Z , Roy , SJ , Zou , Y and Bowman , RS . 1998 . Long term chemical and biological stability of surfactant-modified zeolite . EnvironSciTechnol , 32 : 2628 – 2632 .