Abstract
Multi-soil-layering systems composed of soil mixture blocks (SMB) arranged in a brick-like pattern and surrounded by permeable zeolite layers (PL) were designed for domestic wastewater and polluted river water treatment. To investigate the efficiency of MSL systems in relation to structural differences, five MSL systems with different sizes and layers of SMB and PL were constructed in five 50 cm × 10 cm × 60 cm acrylic boxes. The SMB comprised sandy soil, charcoal, sawdust and iron on a dry weight ratio of 7:1:1:1. Diluted domestic wastewater with an average suspended solids (SS), biological oxygen demand (BOD5), total phosphorus (T-P) and total nitrogen (T-N) concentration of 73, 34, 1.3 and 15 mg L−1, respectively, was applied at a hydraulic loading rate (HLR) ranging from 1,000 to 3,000 L m−2 day−1. At HLR of 1,000 and 2,000 L m−2 day−1, systems with larger SMB surface area had higher removal rates for SS, BOD5, chemical oxygen demand (COD) and T-P because of the enhanced contact efficiency between wastewater and SMB. The structural difference was greatest at a HLR of 2,000 L m−2 day−1, especially for COD and T-P removal. An increase in the top surface area of SMB was found to affect the efficiency of MSL systems more than an increase in side surface area. When the HLR was increased to 3,000 L m−2 day−1, the effect of structural differences on MSL systems was not as great, probably because of preferential water flow into PL as well as the oxidation–reduction potential ORP change caused by the accumulation of SS at high HLR. For T-N removal, no structural difference was found at a low HLR of 1,000 L m−2 day−1 and when HLR increased to more than 2,000 L m−2 day−1, a secondary effect of SS accumulation and ORP change appeared to influence the systems’ performance more than the expansion of SMB surface area.
INTRODUCTION
In ecosystems, soil is not only important as a medium for crop production, but also acts as an ecosphere for the disposal of wastes. Soil's high purification capacity comes from many of its environment-related features, including developed pore systems, co-existence of aerobic–anaerobic and hydrophilic–hydrophobic conditions as well as habitat for various kinds of microorganisms. Soil has been widely researched as a medium for waste treatment because of it's ubiquitous presence and special characteristics for purification (CitationBhamidimarri 1988; CitationReed et al. 1995). However, traditional soil-based wastewater treatment systems display several limitations. Clogging is one of the major limitations even in on-site tank and soil leachline systems (CitationHo and Mathew 1993). Although soil exhibits a high purification capacity, the function of soil wastewater treatment systems strongly depends on the properties of the respective soil type. Therefore, even similar systems do not always perform with the same level of treatment efficiency (CitationSato et al. 2005a).
To fully exploit the environmental purification function of soil, a new type of water treatment system, multi-soil-layering (MSL) system, has been developed and studied. The MSL system is typically composed of soil mixture blocks (SMB) arranged in a brick-like pattern and surrounded by water-permeable particles, such as zeolite and gravel. MSL systems are cheap because they can be developed mainly from local resources, such as soil, sawdust, iron, charcoal, zeolite and alternative materials (CitationBoonsook et al. 2003; CitationLuanmanee et al. 2001). Various studies have been conducted on MSL systems to examine performance characteristics. In a previous study, we found that we could modify the structure of SMB depending on the water quality and treatment target to control and optimize the treatment efficiency of MSL systems. The contact of wastewater with SMB decreased with increasing hydraulic loading rate (HLR), while the process for organic matter, phosphorus and nitrogen removal depended, to a large degree, on physicochemical reactions, such as filtration and adsorption. Thus, it was suggested that effective contact between the wastewater and SMB was a major factor for MSL performance (CitationMasunaga et al. 2003; CitationSato et al. 2005b) and increasing the surface area of the SMB might enhance the removal efficiency of MSL systems. However, to date, there has been no quantitative research on the effect of structural difference on MSL system performance. Therefore, we conducted a laboratory-scale experiment to analyze quantitatively the relationship between size and surface area of SMB and the treatment efficiencies of selected parameters in MSL systems that can contribute to the optimal design of MSL structures suitable for different treatment conditions.
MATERIAL AND METHODS
Structure and components of the MSL systems
shows a detailed description of the structures of the five MSL systems used in this study, with different sizes and layers of SMB. The five MSL systems evaluated in this study were packed in five individual W50 cm × H60 cm × D10 cm acrylic boxes. The SMB was placed in the acrylic boxes in an alternating brick-like pattern. Zeolite with a diameter ranging from 3 to 5 mm was used to fill in the void spaces between adjacent SMB. The SMB was composed of soil (humus poor granite sandy soil), charcoal, sawdust and granular metal iron (20 mesh) at a ratio of 7:1:1:1 on a dry-weight basis and packed at a density of 1.2 g cm−3. MSL 1 had three layers and the height of the SMB was 8 cm. MSL 2, MSL 3 and MSL 4 had six layers and the height of the SMB was 4 cm. MSL 5 had 12 layers and the height of the SMB was 2 cm. MSL 2, MSL 3 and MSL 4 differed mainly in the width of the SMB, 12 cm, 9 cm and 4.5 cm,
Figure 1 Structure and components of the five multi-soil-layering (MSL) systems (W50 cm × H60 cm × D10 cm) used for wastewater treatment. The size of the soil mixture block (SMB) is shown in parentheses (W cm × H cm × D cm).
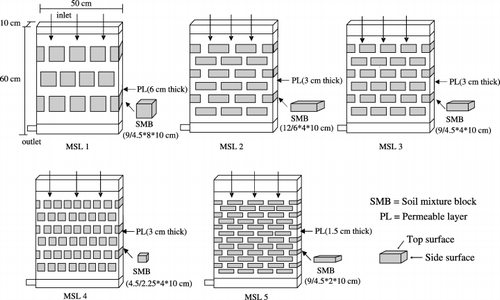
Table 1 Top surface area (cm2), side surface area (cm2) and total volume (cm3) of the soil mixture blocks in the five multi-soil-layering (MSL) systems
Table 2 Operation period, time, multi-soil-layering (MSL) systems, hydraulic loading rate (HLR) and temperature during the three experimental periods
Quality of the wastewater and operating conditions
The wastewater used in this study was from a local community disposal plant and was diluted fourfold before flowing into the systems. In practical terms, we hoped to apply the results from the experiment to the advanced treatment of sewage plant wastewater, individual house wastewater after septic tank treatment or polluted river water treatment. The degree of dilution was decided by using the biological oxygen demand (BOD5) level of approximately 30 mg L−1 that was often observed in the released water from septic tanks of individual houses (Aoyama 2001, unpubl. data). The average properties of the inflow water were as follows: suspended solids (SS) 73 mg L−1, BOD5 34 mg L−1, chemical oxygen demand (COD) 63 mg L−1, total phosphorus (T-P) 1.3 mg L−1 and total nitrogen (T-N) 15 mg L−1. The water quality did not fluctuate greatly and the coefficient of variation was less than 20% for all parameters during the experimental period. The study was conducted over three periods. The HLR increased from 1,000 to 3,000 L m−2 day−1 and the air temperature ranged from 11–16, 5–15 and 20–27°C, respectively, during the three experimental periods (). In each period, the experiment was conducted for more than 4 weeks, and this was considered empirically to be long enough to obtain average data for the discussion of structure influence on water contact efficiency with SMB separately from other factor influences. According to the results from a previous study, MSL systems under similar treatment conditions could keep stable treatment efficiency continuously for 212 days’ operation at a HLR of 1,000 L m−2 day−1 (CitationSato et al. 2005a). However, when the HLR was increased to 3,000 L m−2 day−1, the treatment efficiency of some parameters, such as BOD5 and NH+ 4-N, tended to gradually decrease and the period was extended to 8 weeks to follow the change. New MSL systems with the same structure and composition were re-constructed during Period 3 to avoid the possible influence of accumulated organic matter on the systems’ treatment efficiency. For the same reason, calculation of the mean treatment efficiency was based on data from the first 5 weeks of Period 3 as the removal rates dropped sharply from week six, which might be because of the accumulation of SS. In Period 3, MSL 2 was stopped because the amount of wastewater applied every day was beyond the experimental facility capacities (i.e. pumps and the transportation of wastewater).
Water samples and laboratory analysis
To investigate the effects of structural differences in MSL systems, wastewater and treated water were sampled once per week during the three experimental periods. The oxidation–reduction potential (ORP) was analyzed using the electrometric method, BOD5 using the standard method of the CitationAmerican Public Health Association (1992), COD using the potassium dichromate method, PO3− 4-P using the ascorbic acid method, NH+ 4-N using the Nessler method, NO− 2-N and NO− 3-N using the ion chromatography method (DIONEX DX-120 Suanyvale, USA). T-P and T-N were determined as PO3− 4-P and NO− 3-N after potassium peroxodisulfate digestion.
Statistical analysis of the experimental data
To analyze the structural influence on MSL treatment efficiency for different parameters, we used a logarithmic equation to express the experimental data. The equation was expressed as follows:
Statistical significance of the structural effect on MSL system performance was subjected to a uni-variant analysis using Tukey's honestly significant difference (HSD) method in SPSS (version 12.0) (CitationIshimura 2001).
RESULTS AND DISCUSSION
Effect of structural difference on SS, BOD5 and COD removal
shows the effect of MSL structural differences on the treatment efficiency of SS, BOD5 and COD during the study period. The analysis for wastewater quality at each period showed a coefficient variation of less than 20% for the different parameters (), which means that the wastewater quality did not vary substantially. The MSL systems were quite effective in SS removal, and the removal rates ranged from 90.6% to 97.2% during Period 1 and from 87.1% to 95.8% during Period 2, resulting in the following order: MSL 5 > MSL 4 > MSL 3 > MSL 2 > MSL 1 (). showed that SS removal efficiency differed significantly among the MSL systems as the main mechanism for SS removal was through physical adsorption and filtration by the SMB layers (CitationWakatsuki et al. 1990). An increase in surface area could enhance the contact efficiency of the wastewater with the SMB as well as the filtration capacity of the systems. However, when the HLR increased to 3,000 L m−2 day−1 during Period 3 (), the difference for SS removal efficiency among the systems became smaller. This was probably caused by the increasing ratio of water flow into the permeable layers (CitationSato et al. 2005b).
The trend for BOD5 removal was similar to that of SS when the systems had a HLR of 1,000 and 2,000 L m−2 day−1. Average BOD5 removal rates were 87.5–92.6% during Period 1 and 82.2–91.0% during Period 2 (). At a HLR of 2,000 L m−2 day−1, structural difference among the five MSL systems became clearer than the difference among them during Period 1 at a HLR of 100 L m−2 day−1 (). MSL 5 and MSL 4 were better
Figure 2 Concentration of (a) suspended solids (SS), (b) biological oxygen demand (BOD5) and (c) chemical oxygen demand (COD), and (d) the value of the oxidation–reduction potential (ORP) in the wastewater (WW) and in the treated water of the five multi-soil-layering (MSL) systems as affected by hydraulic loading rate. P, period; W, week.
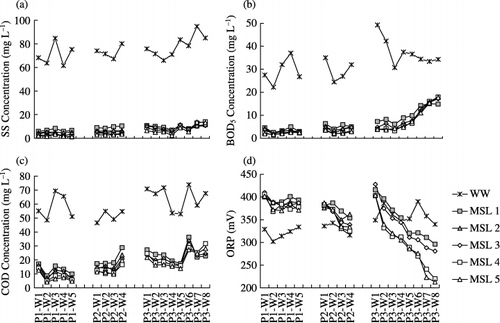
Table 3 Average concentrations (mg L−1) and removal efficiency (%) of suspended solids (SS), biological oxygen demand (BOD5), chemical oxygen demand (COD), total phosphorus (T-P) and total nitrogen (T-N) in the multi-soil-layering (MSL) systems during the three experimental periods
The effect of structural difference on COD removal was greater than the effect on SS and BOD5. During Period 1, COD removal rates remained stable at 80–90% and the average removal rates for the five systems were 77.7–87.0% (). MSL 4 and 5 showed superiority over the other systems and the removal efficiency increased by 9.3% when the top plus side surface area of SMB increased from 3,000 cm2 (MSL 1) to 6,240 cm2 (MSL 5), while the efficiency of SS and BOD removal increased by only 6.6% and 5.1%, respectively, over the same period. The removal of COD might need a more effective contact between the wastewater and the SMB because it represents slowly decomposable organic matter. CitationMbuligwe (2004) also reported that in an engineered wetland system, effective contact between the system and wastewater was necessary to achieve a high treatment for COD. When HLR increased during Period 2, the average removal rates of the MSL systems dropped to 60.2–74.9%, while that of SS and BOD5 remained around the same level during Periods 1 and 2 (). COD removal efficiency was more influenced by the increase in HLR than that of SS and BOD5, possibly because the decomposition of COD requires a longer retention time, and retention time decreases as HLR increases (CitationSato et al. 2005b).
Effect of structural difference on phosphorus removal
shows the change in T-P concentration during the study period and the average removal rates are presented in . During Period 1, T-P removal rates increased and remained stable at 60.8–83.4% after 3 weeks operation because the system needed a few
Figure 3 Concentration of (a) total phosphorus (T-P), (b) -N, (c) -N and (d) total nitrogen (T-N) in the wastewater (WW) and treated water of the five MSL systems as affected by hydraulic loading rate. P, period; W, week.
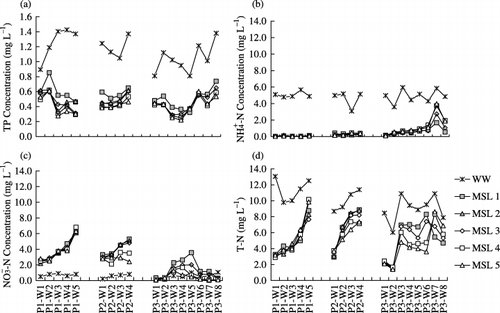
Increasing HLR to 2,000 L m−2 day−1 decreased T-P removal rates in all systems to lower than 70%, and structural differences among the systems became more obvious than those observed in Period 1 (.). The highest average T-P removal rate was found in MSL 5 (65.0%), followed by MSL 4 (63.9%), MSL 3(58.2%), MSL 2 (57.3%) and MSL 1 (51.9%). MSL 4 and 5 were significantly different from MSL 1 and 2 in T-P removal because of the expanded surface area, which enhanced the contact efficiency of wastewater and SMB. Reducing sizes and increasing numbers of SMB achieved a more effective T-P removal result by increasing the adsorption sites in the surface of SMB.
When HLR was set at 3,000 L m−2 day−1 during Period 3, average removal rates for the systems became lower compared with the rates recorded during Periods 1 and 2 when the HLR was lower. The removal efficiency difference became smaller among the systems and only MSL 1 and MSL 5 showed a significant difference (). The high HLR influenced the treatment efficiency and narrowed the effect of structural difference among the systems by hindering the fixation of phosphorus and oxidation of ferrous iron through loss of adsorption sites and a decrease in ORP as well as increasing the ratio of water flow into the PL (CitationSato et al. 2005b).
Effect of structure difference on nitrogen removal
Nitrogen in the wastewater was mainly in the form of NH+ 4-N and its concentration ranged from 3 to 6 mg L−1. Concentration of NH+ 4-N in treated water remained low for all systems during Periods 1 and 2 () because of adsorption on zeolite, which had a high cation exchange capacity (CEC) of approximately 110 cmolc kg−1. At the beginning of each period, the MSL systems showed a high capacity to remove T-N from wastewater for all the systems (). However, T-N concentration in the treated water increased with time because of the increase in NO− 3-N in the treated water ().
During Period 1, there was no significant difference in NH+ 4-N, NO− 3-N and T-N concentrations in the treated water among the five systems (). Although during Period 2, NO− 3-N and T-N concentrations were
lower in MSL 4 and 5 than in the other systems. As NH+ 4-N concentration in the treated water was still low, as in Period 1, better T-N removal rates resulted from the lower NO− 3-N concentration in MSL 4 and 5. This was possibly because of the high rate of denitrification that might be induced by lower ORP () because the higher removal rates of SS, BOD5, COD in these two systems needed to consume more oxygen for organic decomposition. A similar trend was also found during Period 3. It is possible that the secondary effect caused by structural differences, such as ORP change and the accumulation of SS, influenced nitrogen removal more than the surface area change did as the process of nitrogen removal in MSL systems consists of mineralization of organic N and nitrification of NH+ 4-N under aerobic conditions followed by denitrification under anaerobic conditions. This is more complicated than the removal process of other parameters such as SS, BOD5, COD or phosphorus, which are removed through adsorption and decomposition under aerobic conditions. Thus, the NH+ 4-N, NO− 3-N and T-N concentrations in treated water became unstable during Period 3 in the present study. To discuss the influence of structural difference on T-N removal we need to separately analyze the data in the two phases, aerobic and anaerobic, in which the different processes occur. Thus, further data analysis and discussion focused on SS, BOD5, COD and T-P removal efficiencies in relation to structural differences in MSL systems.Relationship between the treatment efficiency of selected parameters and the surface area of SMB in MSL systems
shows the relationship between treatment efficiency of SS, BOD5, COD, T-P and the top plus side surface area of SMB at HLR of 1,000 and 2,000 L m−2 day−1. At a high HLR of 3,000 L m−2 day−1, the efficiency of the MSL systems was possibly influenced by the secondary effect of structural difference, such as change of ORP and accumulation of organic matter, but these data were not included in the figures so that we could concentrate on the direct influence of structural difference. As shown in , with the increase in the top plus side surface area of SMB (), the average removal efficiency for SS, BOD5, COD and T-P increased at HLR of 1,000 and 2,000 L m−2 day−1. In general, MSL systems’ removal efficiency for SS, BOD5, COD and T-P was in the order of MSL 5 = MSL 4 > MSL 3 > MSL 2 > MSL 1, which was positively related to the top plus side surface area of the SMB in the systems.
In , the influence of an increase in the top surface area (MSL 1, 3, 5) or side surface area of SMB (MSL 2, 3, 4) on the treatment of SS, BOD5, COD and T-P was shown separately. The decrease in SMB height
Figure 5 Relationship between the average removal rates of (a) suspended solids (SS), (b) biological oxygen demand (BOD5), (c) chemical oxygen demand (COD) and (d) total phosphorus (T-P) and the top plus side surface area of the soil mixture blocks (SMB). P, period.
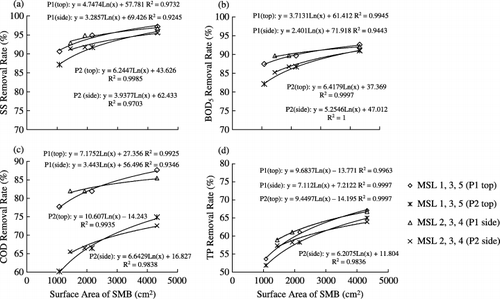
It is clear from the present study that MSL systems with smaller (thinner and narrower) SMB are better for SS, BOD5, COD and T-P removal because of the enhanced contact efficiency. This result agreed with the findings of CitationSato et al. (2005c), which indicated that MSL systems with narrower SMB had a larger side surface area and resulted in higher efficiency in BOD5 and T-P removal. However, if the HLR and pollutant concentration were high, systems with smaller SMB might exert influence on the treatment efficiency of the systems, especially on nitrogen removal, by changes in ORP and accumulation of SS. Therefore, we need to consider this secondary effect when designing MSL system structures. In order to generalize the findings from this research and apply it for optimal MSL structural design in the future, we need to conduct further study and collect more data on different water quality and treatment conditions.
Conclusion
Multi-soil-layering systems with thinner and narrower SMB showed a higher removal efficiency for SS, BOD5, COD and T-P treatment at HLR from 1,000 to 2,000 L m−2 day−1 because of the better contact efficiency between wastewater and SMB caused by the expansion of the top and side surface areas of the SMB. The effect of structural difference on the performance of MSL systems was more obvious at a HLR of 2,000 L m−2 day−1, especially for COD and T-P, which require more efficient contact with SMB for adsorption and decomposition. When HLR was increased to 3,000 L m−2 day−1, the difference among the systems became smaller probably because of preferential water flow into PL as well as ORP change caused by SS accumulation within the systems. This type of secondary effect of structural difference influenced T-N removal more than the expansion of SMB surface area did, although detailed discussion could not be conducted based on the present study. When designing MSL systems for wastewater treatment, we need to evaluate both the first and secondary effects of structural differences based on the different treatment targets and conditions, especially at high HLR and pollutant concentrations.
ACKNOWLEDGMENT
The authors would like to express their deep gratitude to the Ministry of Education, Science, Sport and Culture of Japan for financial assistance for this study.
REFERENCES
- Bhamidimarri , R . 1988 . “ Alternative waste treatment systems ” . In Chapter 6 Poster Papers , Edited by: Bhamidimarri , R . 174 – 249 . London : Elsevier .
- Reed , SC , Crites , RW and Middlebooks , EJ . 1995 . “ Natural systems for waste management and treatment ” . In Chapter 6 Wetland System , 2nd edn , Edited by: Reed , SC . 173 – 281 . New York : McGraw-Hill .
- Ho , G and Mathew , K . 1993 . Appropriate waste management techonologies IAWQ . Water SciTechnol , 27 ( 1 ) : 220
- Sato , K , Masunaga , T and Wakatsuki , T . 2005a . Characterization of treatment process and mechanisms of COD, phosphorus and nitrogen removal in a multi-soil-layering system . Soil SciPlant Nutr , 51 ( 2 ) : 213 – 221 .
- Boonsook , P , Luanmanee , S Attanandana , T . 2003 . A comparative study of permeable layer materials and aeration regime on efficiency of multi-soil-layering system for domestic wastewater treatment in Thailand . Soil SciPlant Nutr , 49 ( 6 ) : 873 – 882 .
- Luanmanee , S , Attananndana , T Masunaga , T . 2001 . The efficiency of a multi-soil-layering system on domestic wastewater treatment during the ninth and tenth years of operation . EcoEng , 18 : 185 – 199 .
- Masunaga , T , Sato , K Zennami , T . 2003 . Direct treatment of polluted river water by the Multi-soil-layering method . JWater EnvTech , 1 ( 1 ) : 97 – 104 .
- Sato , K , Masunaga , T and Wakatsuki , T . 2005b . Water movement characteristics in a multi-soil-layering system . Soil SciPlant Nutr , 51 ( 1 ) : 75 – 82 .
- American Public Health Association . 1992 . “ Standard method for the examination of water and wastewater ” . In Chapter 5 Aggregate Organic Constituents , 18th edn , Edited by: Young , JC . 5152 – 5210 . Washington : American Public Health Association .
- Ishimura , S . 2001 . “ Statistical analysis method for SPSS ” . In Chapter 7 , Edited by: Ishimura , S . 116 – 129 . Tokyo : Tokyo Pub . (in Japanese)
- Wakatsuki , T , Omura , S Abe , Y . 1990 . Treatment of domestic wastewater by Multi-Soil-Layering system (Part 3) Role and life of purification abilities of soil materials in the systems . JpnJSoil SciPlant Nutr , 61 : 74 – 84 . (in Japanese with English summary)
- Mbuligwe , SE . 2004 . Comparative effectiveness of engineered wetland systems in the treatment of anaerobically pre-treated domestic wastewater . EcoEng , 23 : 269 – 284 .
- Wakatsuki , T , Esumi , H and Omura , S . 1993 . High performance and N&P removal on-site domestic wastewater treatment system by multi-soil-layering method . Water SciTechnol , 27 ( 1 ) : 31 – 40 .
- Sato , K , Masunaga , T Inada , K . 2005c . The development of high speed treatment of polluted river water by the multi-soil-layering method: examination of various materials and structures . JpnJSoil SciPlant Nutr , 76 : 449 – 458 . (in Japanese with English summary)