Abstract
The effects of steam-treated (hydrolytic process using high temperature 207°C and high pressure steam 1.6 MPaG for 30 min) grass clippings and their liquid residue on growth, drainage water quality and soil microbial properties were evaluated. Soils were placed in plastic containers (47 cm length × 40 cm width × 22.5 cm depth) so that the surface (0–10 cm depth) was a mixture of Andisol and sand (3:2 volume) and the subsurface soil (10–20 cm depth) was Andisol only. A basal dose of chemical fertilizer (25 g m−2 NPK 8–8–8) and poultry manure (20 g m−2) was applied. The treatments were: CF (without incorporation of plant residues and 25 g m−2 NPK 8–8–8 top dressing 1 month after transplanting), LL (1 kg m−2 commercial leaf litter), GT (1 kg m−2 steam-treated grass clippings), GT + BL (1 kg m−2 steam-treated grass clippings plus 6 L m−2 liquid residue in a single application as basal fertilizer), GT + FL (1 kg m−2 steam-treated grass clippings plus 3.2 L m−2 liquid residue diluted 10-fold and applied six times as fertigation), and GT10 (10 kg m−2 steam-treated grass clippings). In all treatments, Manila grass (Zoysia matrella) was transplanted. The total dry matter yield of clippings was highest in GT10 (752 kg m−2), followed by GT (503 kg m−2), LL (491 kg m−2) and lowest in CF (378 kg m−2). Liquid residues did not show a positive effect on grass growth, probably because of low pH (4.5). Incorporation of high amounts of steam-treated grass clippings (GT10) can result in more nitrate leaching. The trends in microbiological properties in response to the different treatments were the same as the grass growth (GT10 > GT > LL > CF). Steam-treated grass clippings can enhance microbial activities and can partially replace chemical fertilizer. However, the incorporation of an adequate amount is necessary to minimize the environmental load by nitrate leaching. Liquid residue requires another form to be used.
INTRODUCTION
In Japan, increasing need for green space management, such as in parks and golf lands, leads to an increase in the amount of plant residues, such as grass clippings and pruning branches. In general, these residues are incinerated as common organic wastes, while lesser quantities are used as an organic resource and recycled in agricultural land (CitationKitou and Yoshida 1992). Large-scale incineration requires large amounts of fuel and may cause air pollution from SOx, NOx, CO, CO2, hydrogen chloride and chlorinated hydrocarbons including dioxins, unless the incinerators are equipped with appropriate pollutant control devices (CitationHaruta et al. 2005).
Large quantities of plant residues are also placed in landfills. However, this can result in rapid exhaustion of landfill capacity and may also cause pollution of groundwater and air (CitationHaruta et al. 2005). Composting is the best option for treating organic residues in many cases. However, in golf lands, grass clippings are collected frequently because of rapid grass growth stimulated by frequent irrigation and heavy fertilization, making composting difficult. Moreover, this process can result in a bad smell that may be annoying for visitors and neighbors.
Alternative time-saving and space-saving methods of treating grass clippings need to be established. Furthermore,
it is mandatory to develop environmental protection technologies that will have a lower impact on the environment (CitationNoike 2005).Steam treatment may be an alternative method. This method reduces the volume of fresh plant residues in a short time and the product after steam treatment can be recycled back into the soil as conditioner. Incorporation of plant residues into the soil supplies organic matter that enhances biological activities, improves physical properties and increases the nutrient availability of the soil. However, the use of a new organic source as soil amendment requires some agro-environmental assessment.
Some eventual problems associated with organic residues are: (i) the release of easily decomposable materials that may pollute the river and groundwater with nitrate and phosphate through runoff and leaching; (ii) the release of organic acids or allelopathic substances that can prejudice plant germination and growth, and microbial activity; (iii) organic matter with high C:N ratios can cause nitrogen deficiency through a microbial nitrogen immobilization process, (iv) imbalance of nutrients in the soil that may consequently damage plant growth; and (v) the release of heavy metals that can destroy the soil biota.
A golf course is characterized by a high leaching potential because it has a sand base and receives frequent irrigation (CitationMuramatsu and Nioh 1994; CitationShuman 2003). Thus, the management of golf courses requires special attention. Therefore, the objective of this study was to evaluate the influence of steam-treated grass clippings and liquid residue derived from this process as new organic resources in relation to grass growth, drainage water quality and soil microbiological properties after incorporation into microcosms that simulated a golf course.
MATERIALS AND METHODS
Steam-treatment process
Steam treatment is a hydrolytic process using high temperature (207°C) and high-pressure steam (1.6 MPaG) for 30 min. These processes, as well as the treating machine, were recently developed by Ishikawajima-Harima Heavy Industries (Yokohama, Japan). The maximum capacity of this machine is 300 kg batch−1 for grass clippings. The steam treatment emits 1/7 less CO2 than incineration and does not emit dioxin (CitationSasa et al. 2004). This process can reduce the volume and weight of grass clippings by 70% and 40%, respectively. This reduction in weight means the release of water from fresh grass clippings during the steam-treatment process. Approximately 20% of this water release (approximately 8% of the total initial weight) is dark brown liquid, referred to hereafter as liquid residue. Grass clippings of Japanese lawngrass (Zoysia japonica Steud) before and after steam treatment are shown in . The color of the grass clippings changed from green to dark brown-black and the clippings became thinner and soft by reducing the water content and softening the plant material. However, the steam-treated grass clippings maintained a similar shape to the non-treated grass clippings.
Experimental setting
The experiment was conduced at the Faculty of Horticulture, Chiba University, Matsudo City, Chiba, Japan, from July 2004 to September 2005. Treatments were arranged in a randomized complete block design. The open-field microcosm simulating a golf course was placed in a container (47 cm length × 40 cm width × 22.5 cm depth) that had two soil layers: (i) surface soil, a mixture of Andisol and sand (3:2 volume); and (ii) subsurface soil, Andisol only (). The Andisol was collected in Matsudo City and classified as Light-colored Andisol (Typic Hapludand) with loam texture (CitationSakamoto and Hodono 2000).
The experiment had six treatments with three replicates in each treatment (). In all treatments, 25 g m−2 chemical fertilizer (NPK 8–8–8) and 20 g m−2 commercial poultry manure (NPK 2.0–2.1–3.6) were incorporated as basal fertilizer. Two treatments were setup as a control: chemical fertilizer (CF) and commercial leaf
Figure 2 Schematic diagram of plant material, liguid residue and the golf course microcosms simulated using plastic containers. CF, chemical fertilizer.
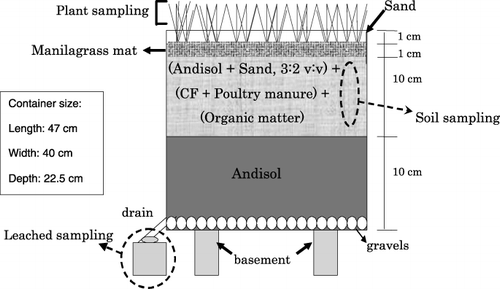
Table 1 Amount of plant material, liquid residue and fertilizer applied per m−2 in the different treatments
In May 2005, all treatments received 10 g m−2 chemical fertilizer (NPK 8–8–8) as top dressing. This low amount of fertilizer was applied to reduce the effect of chemical fertilizer, to maximize the residual effect of organic matter applied in 2004, and to verify the effect of steam-treated grass clippings. In 2005, the application of liquid residues was suspended because of a negative effect observed in plant growth. The treatments with liquid residue (GT + BL and GT + FL) were evaluated in 2004 only, with respect to plant growth and drainage water quality. In the other treatments, grass growth and drainage water quality were evaluated in both years (2004 and 2005) and soil microbiological properties were evaluated in 2005.
Grass growth sampling
Grass clippings were collected twice in 2004 (August and September) and 2005 (July and September). Grass
Figure 3 (a) Sampling times and main management during the experiment until 1 October 2005 and (b) rainfall until 26 June 2005. CF chemical fertilizer; GT + FL, 3.2 L m−2 of liquid residue was diluted 10-fold and applied as fertigation six times, once per week, starting 1 month after transplanting in addition to 1 kg m−2 steam-treated grass clippings.
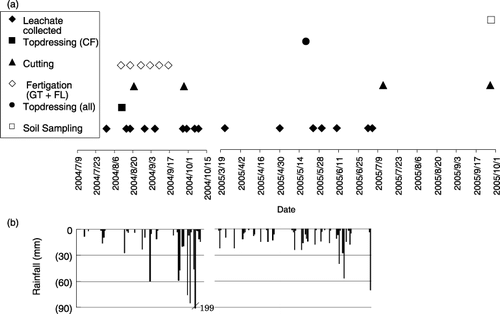
The main management and sampling timing are summarized in .
Drainage water sampling
Drainage water (> 50 mL) was collected for laboratory analyses. The total amount of leachate depended on the rainfall. Rainfall data were obtained from the CitationMinistry of Land, Infrastructure and Transport, Japan (2005), representing the nearest station located in Matsudo City.
The pH and electrical conductivity (EC) were measured and the sample was filtered with filter paper (Advantec No.6) for the analyses of nitrate, ammonium and phosphates. After transplanting until the middle of autumn 2004, the leachate was sampled periodically nine times. Sampling was stopped after the 9th sampling because no detectable amount of nitrate was observed in the 5th to the 9th samples taken. In March 2005, water sampling was reinitialized and strategically collected when the nitrification process may increase with increases in temperature and after chemical application as top dressing in May 2005.
Soil sampling
Soil used in this experiment for analyses of chemical properties was collected prior to incorporation of chemical and organic fertilizers.
At the end of September 2005, two subsamples per replicate were taken for soil biochemical and microbiological properties from the 4–10 cm depth without the mat layer, representing the original surface layer where the chemical fertilizer and organic matter were applied.
Soil samples were sieved to 2 mm, mixed and homogenized. One part of this soil was air-dried for 7 days and the other part was stored in a refrigerator at 4°C for soil biochemical analyses.
Chemical and microbiological analyses
Total carbon (C) and nitrogen (N) in the soil and organic matter were determined using a Yanaco MT-700 CN corder (Yanagimoto, Kyoto, Japan). Total potassium (K) and phosphorus (P) in the soil and organic matter were determined from nitric acid digests using inductively coupled plasma (ICP) (ICPS-1000IV, Shimadzu). The amounts of calcium (Ca) and magnesium (Mg) in the organic matter were also measured in the nitric acid digests using atomic absorption spectrometry
Table 2 Chemical properties of the soil
Table 3 Chemical properties of the organic matter
The nitrate-N (NO− 3-N) and ammonium-N (NH+ 4-N) contents in the soil extracted using 0.5 mol L−1 K2SO4 (1:5 soil : extract) and leachate were determined using the hydrazine reduction method (CitationHayashi et al. 1997) and the nitroprusside method (CitationAnderson and Ingram 1989), respectively. Phosphorus ions in the leachate were determined using the method described by CitationOlsen and Sommers (1982). The amount of dissolved organic carbon (DOC) and dissolved nitrogen (DN) in the liquid residue were determined using automatic combustion (Shimadzu, TOC 5000) and the persulfate oxidation method (CitationCabrera and Beare 1993), respectively. The pH of the liquid residue was measured using the same pH meter as described above. Electric conductivity was measured using a portable EC meter (TOA, CM-14p; TOA, Tokyo, Japan).
Soil microbial biomass C was determined using the chloroform fumigation–extraction method described by CitationVance et al. (1987). The total organic C content in the extract was measured according to the method described above. The soil enzyme activities, dehydrogenase, phosphatase and protease, were determined according to the methods described by CitationCasida (1964), CitationTabatabai and Bremer (1969) and CitationLadd and Butler (1972), respectively. For measuring soil respiration, fresh sieved soil was aerobically pre-incubated for 7 days with 60% water-holding capacity at 25°C. This procedure permits uniform rewetting and allows microbial activity to settle down after the initial disturbances (CitationChander et al. 1997). After pre-incubation, 10 g of soil was placed in a 100 mL bottle with a stopper and incubated for 28 days in the same condition described for pre-incubation to measure microbial respiration. The CO2 production was determined every week using gas chromatography with thermal conductivity detector (TCD), Shimadzu GC-14B (CitationMurakami et al. 2005). The amounts of CO2 produced each week were added to determine the cumulative C mineralized during the incubation period. The respiratory quotient was obtained by dividing the rate of soil respiration (in the last week of incubation) by the amount of microbial biomass.
Statistical analyses
Statistical analyses were carried out using the statistical package SPSS 11 for Windows (SPSS Inc., Chicago, IL, USA). Differences among treatments and data variances were determined by anova. Comparisons of means among treatments were done using least significant differences (LSD) at P < 0.05.
RESULTS
Chemical properties of the soil, organic matter and liquid residue
The results showed a lower amount of C, N, P and K in the surface soil than in the subsurface soil because it was diluted by sand (). The difference was approximately 2.4-fold in N and 2.7-fold in C. Nitrate and ammonium contents were not significantly different in the surface and subsurface soils. The soil pH value was very similar in the two layers.
Significant differences were observed among plant residues in terms of their chemical properties (). The steam-treated grass clippings (GT) had more N, P and K contents and were approximately 2.3-fold, 3.4-fold and 8.8-fold greater than LL. In contrast, the amounts
Table 4 Chemical properties of the liquid residue
Figure 4 Dry matter of grass clippings in different treatments during two cuts in 2004 and two cuts in 2005. Statistical analyses of total dry matter: least significant difference (P < 0.05). The treatments GT + BL and GT + FL were only conducted at the first and second cuttings. CF, chemical fertilizer; LL, commercial leaf litter; GT, 1 kg m−2 steam-treated grass clippings; GT10, 10 kg m−2 steam-treated grass clippings; GT + BL, 6 L m−2 liquid residue was applied once as basal fertilizer in addition to 1 kg m−2 steam-treated grass clippings; GT + FL, 3.2 L m−2 of liquid residue was diluted 10-fold and applied as fertigation six times, once per week, starting 1 month after transplanting in addition to 1 kg m−2 steam-treated grass clippings.
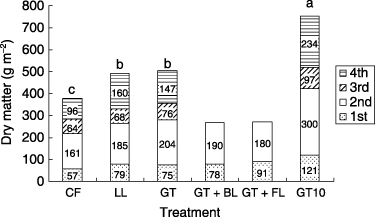
Low amounts of total and mineral N, and low pH were observed in liquid residues ().
Grass growth
In 2004 (first and second cuttings), the amount of grass clipping dry matter of Manila grass varied from 218 in CF to 421 kg m−2 in GT10 (). The amount of dry matter in GT was similar to LL, but higher than CF. Dry matter of GT10 was 1.5-fold higher than GT. In GT + BL, initial damage was visually observed after the application of concentrated liquid, but later recuperated. In the second cutting, a smaller amount of dry matter was observed in GT + FL compared with GT.
The amount of dry matter in 2005 was less than in 2004 in all treatments. Moreover, the dry matter yield was similar in both years with GT10 showing the highest and CF the lowest dry matter yield ().
The total dry matter yield of grass clippings was statistically highest in GT10 (752 kg m−2) and lowest in CF (378 kg m−2). There was no statistical difference between GT and LL in terms of dry matter yield. The total dry matter in GT10 was 1.5-fold greater than GT.
Drainage water quality
The pH value of the drainage water varied from 6.5 to 7.5, with little difference among treatments (). The EC values declined in the second sampling in all treatments except GT10. The EC value of GT10 increased from 1.9 dS m−1 in the first sampling to 2.5 in the third sampling and then decreased gradually. In other treatments, the values were approximately 1.5 in the first sampling and then decreased to less than 0.7 dS m−1 in the second sampling, and then remained at a constant level after that ().
In 2004, nitrate concentrations had similar values (30 mg L−1) in the first sampling, except in GT10 which had 21.5 mg L−1 (). These amounts are greater than the maximum limit of 10 mg L−1 for drinking water (CitationMinistry of the Environment, Japan, 2005). In the second sampling, however, all samples had values lower than 10 mg L−1 and maintained values of less than 2 mg L−1 in succeeding samplings, except in GT10 which had values ranging from 7 to 9 mg L−1 and declined to less than 2 mg L−1 by the fifth sampling. In 2005 (10–16th sampling time), no detectable or significant amounts of nitrate were found in any of the treatments.
The total amount of nitrate leached in drainage water was estimated only until the fourth sampling when the nitrate concentration was detectable. The results showed similar amounts in all treatments (data not shown).
In 2004, initial ammonium concentrations ranged from 1.9 to 3.3 mg L−1, but declined significantly to less than 0.5 mg L−1 in all treatments from the second sampling (). In 2005, the concentration was less than 0.5 mg L−1 in the 11th and 12th collections in all treatments. A small peak was observed in the 13th sampling in LL, while in GT and GT10 small peaks were observed in the 15th sampling.
Phosphate was measured in 2004, but was not detectable through the year (data not shown).
Soil biochemical properties
The amounts of total C and N in the soil were significantly higher in GT10 compared with the other treatments (). The lowest total C and N contents were observed in CF. Total C and N values of LL and GT were not significantly different from each other. Extractable C showed a similar trend to total C and N. In all treatments, the soil pH was similar.
Figure 5 Changes in drainage water (a) pH, (b) electrical conductivity (EC), (c) nitrate concentration and (d) ammonium concentration. Error bars in EC, pH and ammonium concentration are the standard deviation, and in nitrate concentration the error bars indicate the least significance difference values at P < 0.05. Leached samplings: 1∼9 in 2004 and 10∼16 in 2005.
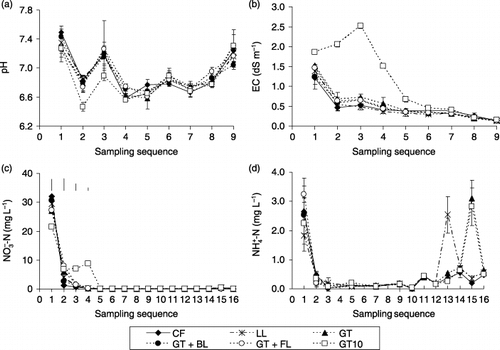
Table 5 Total carbon and nitrogen, extractable carbon and pH in the surface layer (4–10 cm) sampled at the end of the growing season in 2005
The amount of microbial biomass was significantly higher in GT10 compared with the other treatments (). This amount was almost 2.5-fold higher than GT. The lowest value was observed with CF, but no significant difference was observed between LL and CF.
Dehydrogenase activity was highest in GT10, but it was not significantly different from that of LL and GT. The lowest value was observed with CF, but it was also not significantly different from that of LL and GT (). Protease activity showed a similar pattern to dehydrogenase activity (). Protease activity of GT10 was not statistically different from GT. Similarly, GT and LL were not significantly different in terms of protease activity. The lowest protease activity was observed with CF. Phosphatase activity was statically lowest in CF compared with the other treatments (). Among LL, GT and GT10, no significant differences were observed in terms of phosphatase activity.
Soil respiration was 1.4-fold greater in GT10 than in GT (). Among the other treatments, no significant differences were observed, but the lowest respiration was observed in CF.
Metabolic coefficient (amount of C mineralized as soil respiration per microbial biomass) was statistically lower in GT10 than the other treatments, which did not differ from each other ().
DISCUSSION
The larger amount of dry matter in GT compared with CF indicates that steam-treated grass clippings can partly substitute for chemical fertilizer. The similar
Figure 6 Soil biochemical and microbiological properties in the surface layer (4–10 cm): (a) microbial biomass carbon, (b) dehydrogenase activity, (c) protease activity, (d) phosphatase activity, (e) soil respiration and (f) microbial metabolic quotient. Values with the same letter are not significantly using least significance difference values at P < 0.05. Error bars indicate standard deviation. Note: Soil respiration refers to the cumulative carbon mineralized during the incubation period. CF, chemical fertilizer; LL, commercial leaf litter; GT, 1 kg m−2 steam-treated grass clippings; GT10, 10 kg m−2 steam-treated grass clippings.
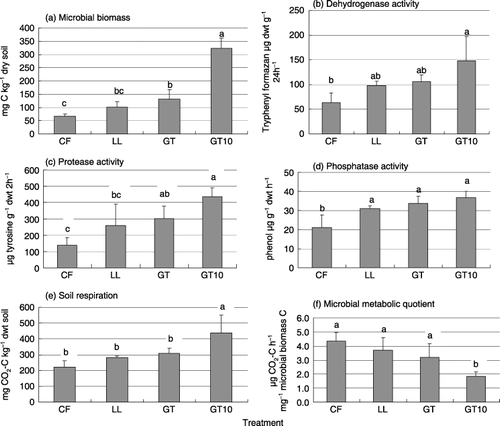
The liquid residue also contains nutrients, but it did not contribute to grass growth in the form that it was used. In GT + BL, the liquid residue caused visual damage. In GT + FL, principally during the second cutting, fertigation had a slightly negative effect on plant growth. It is likely that the low pH and/or possible presence of phytotoxic substances, such as phenol and organic acids, in this liquid may have affected the root growth negatively, especially at high concentrations.
The pH value of the drainage water varied in the neutral zone in all treatments, probably because of the balance between cations and anions. However, the higher EC value in GT10 showed that high amounts of ions leached when large amounts of organic matter were applied because of greater amounts of water-soluble substances.
In general, the higher amounts of nitrate and ammonium in the drainage water in the first sampling probably occurred because grass growth was not yet established and lower nutrient uptake was associated with rapid nitrification process during the summer season. CitationZachary and Petrovic (2004) reported that new grass establishment can promote water contamination because of leaching.
Another factor contributing to higher levels of nitrate in the first sampling was disturbance to the reconstituted soil minerals when the microcosm containers were prepared (CitationWong et al. 1998). After that, the concentrations decreased with the establishment of the plants. High levels were also observed in CF where the chemical fertilizer was applied as top dressing, 1 month after transplanting, but no effect of leaching was observed.
In GT10, the smaller concentration of nitrate in the first sampling probably resulted from an initial immobilization of mineral nitrogen by microorganisms, probably because a high amount of carbon became available because of the incorporation of a large amount of steam-treated grass clippings. The greater concentration of nitrate in three consecutive samplings probably resulted from the release of nitrate initially immobilized and additional nitrate mineralized from steam-treated grass clippings. However, the application of large amounts of steam-treated grass clippings in GT10 did not result in greater amounts of total nitrate leached. The different trends in EC and nitrate in GT10 indicate that large amounts of steam-treated grass clippings can be more susceptible to leaching ions and other elements, such as soluble organic nitrogen, which is considered a readily decomposable nitrogen form. However, the liquid residue did not indicate any effect on nitrate and ammonium leaching because of the low nitrogen content. The high capacity of Andisol to form phosphorus complexes is widely understood (CitationNagatsuka 1997). Therefore, the absence of phosphate in drainage water was probably because of the formation of P-complexes in the soil.
The steam-treated treatment (GT) had similar and higher amounts of microbial biomass and microbial activity (soil respiration and dehydrogenase), as well as enzyme activities (protease and phosphatases) when compared with commercial litter and the treatment without steam-grass (CF), respectively. In addition, in treatments where large amounts (10-fold) of steam-treated grass were applied, a higher microbial biomass, soil respiration and a tendency for higher dehydrogenase, protease and phosphatase activities were found.
Some reports show that when compost of municipal waste or sewage sludge is applied in high amounts, the soil enzymes are negatively affected, probably by the higher level of heavy metals (CitationCrecchio et al. 2004; CitationMarcote et al. 2001). CitationChander et al. (1995) suggest that allelochemicals released into the soil during decomposition of eucalyptus leaves had a toxic effect on soil microorganisms. In the present experiment, large amounts of steam-treated grass clippings had no negative effects on soil microorganisms, indicating no harmful effect of any substances released from steam-treated grass clippings.
The higher respiratory quotients (qCO2) infer stress or adverse environmental conditions. In addition, the metabolic quotient is high when the microorganisms cannot fully metabolize C efficiently while feeding on N (CitationMiyittah and Inubushi 2003; CitationZaman et al. 2002). In this study, increased amounts of applied steam-treated grass clippings show a reduction in respiratory quotients, which indicates a positive effect of this organic resource.
This biological effect on soil microorganisms confirmed our previous work (CitationUshiwata and Inubushi 2005), when a short incubation experiment (10 weeks) was conducted and the results showed that steam-treated grass clippings increased the water-soluble organic carbon and consequently increased the soil microbial biomass.
The present experiment showed that steam-treated grass clippings had a good influence on soil microbial biomass, microbial activity and enzyme activity, and probably on soil physics.
Conclusions
Incorporation of steam-treated grass clippings contributed to plant growth and enhanced microbial activity. The results from this study indicate that steam-treated grass clippings can be used as a new organic matter resource, which can be recycled back into the soil. However, the incorporation of an adequate amount is necessary to minimize the environmental load by nitrate leaching. In relation to the liquid residue, a negative effect on plant growth was found, which suggests that another way to use the liquid residue is needed.
There is a need to conduct further studies in actual field conditions to verify the results obtained from this experiment and to optimize the application rate of steam-treated grass clippings under plant species.
ACKNOWLEDGMENTS
The authors thank Mr N. Shimoda of Ishikawajima-Shibaura Machinery for providing technical support to conduct this experiment. We also thank Dr Agnes T. Padre (International Rice Research Institute, Philippines) for valuable suggestions.
REFERENCES
- Anderson , JM and Ingram , JSI . 1989 . “ Colorimetric determination of ammonium ” . In Tropical Soil Biology and Fertility: A Handbook of Methods , 42 – 43 . Wallingford : CAB International .
- Cabrera , ML and Beare , MH . 1993 . Alkaline persulfate oxidation for determining total nitrogen in microbial biomass extracts . Soil Sci. Soc. Am. J , 57 : 1007 – 1012 .
- Casida , LEJ , Klein , DA and Santoro , T . 1964 . Soil dehydrogenase activity . Soil Sci , 98 : 371 – 376 .
- Chander , K , Goyal , S and Kapoor , KK . 1995 . Microbial biomass dynamics during the decomposition of leaf litter of poplar and eucalyptus in a sandy loam . Biol. Fertil. Soils , 19 : 357 – 362 .
- Chander , K , Goyal , S , Mundra , MC and Kapoor , KK . 1997 . Organic matter, microbial biomass and enzyme activity of soil under different crop rotations in the tropics . Biol. Fertil. Soils , 24 : 306 – 310 .
- Crecchio , C , Curci , M , Pizziagallo , MDR , Ricciuti , P and Ruggiero , P . 2004 . Effects of municipal solid waste compost amendments on soil enzymes activities and bacterial genetic diversity . Soil Biol. Biochem , 36 : 1595 – 1605 .
- Haruta , S , Nakayama , T Nakamura , K . 2005 . Microbial diversity in biodegradation and reutilization process of garbage . J. Biosci. Bioeng , 99 : 1 – 11 .
- Hayashi , A , Sakamoto , K and Yoshida , T . 1997 . A rapid method for determination of nitrate in soil by hydrazine reduction procedure . Jpn. J. Soil Sci. Plant Nutr , 68 : 322 – 326 . (in Japanese with English summary)
- Kitou , M and Yoshida , S . 1992 . Chemical and cultural properties of plant culture soils made by composting some weed and crop residues . Jpn. J. Soil Sci. Plant Nutr , 63 : 511 – 516 . (in Japanese with English summary)
- Ladd , JN and Butler , JHA . 1972 . Short-term assays of soil proteolytic enzyme activities using proteins and dipeptide derivatives as substrates . Soil Biol. Biochem , 4 : 19 – 30 .
- Marcote , I , Hernandez , T , Garcia , C and Polo , A . 2001 . Influence of one or two successive annual applications of organic fertilisers on the enzyme activity of a soil under barley cultivation . Biores. Technol , 79 : 147 – 154 .
- Ministry of Land, InfrastructureTransport 2005 Water Information System. Available from: http://www1.river.go.jp/
- Ministry of the Environment2005Laws and Regulations. Available from: http://www.env.go.jp/en/lar/index.html
- Miyittah , M and Inubushi , K . 2003 . Decomposition and CO2-C evolution of okara, sewage sludge, cow and poultry manure composts in soils . Soil Sci. Plant Nutr , 49 : 61 – 68 .
- Murakami , M , Furukawa , Y and Inubushi , K . 2005 . Methane production after liming to tropical acid peat soil . Soil. Sci. Plant Nutr , 51 : 697 – 699 .
- Muramatsu , K and Nioh , I . 1994 . Effect of the kind of fertilizers on the leaching of the components by rainfall, and growth and load tolerance of turf . Jpn. J. Soil Sci. Plant Nutr , 65 : 514 – 521 . (in Japanese with English summary)
- Nagatsuka , S . 1997 . “ Andosol Class ” . In Ecological Atlas of Soils in Japan , Edited by: Nagatsuka , S . 63 – 73 . Tokyo : Fuji Technosystem . (in Japanese)
- NoikeT2005Present Status of Biowaste Recycling in Japan. Available from: http://www.jora.jp/anor/eng/img/noike.pdf
- Olsen , SR and Sommers , LE . 1982 . “ Phosphorus ” . In Methods of Soil Analysis , Edited by: Page , AL . 815 – 820 . Madison : American Society of Agronomy and Soil Science Society of America . part 2.
- Sakamoto , K and Hodono , N . 2000 . Turnover time of microbial biomass carbon in Japanese upland soils with different textures . Soil Sci. Plant Nutr , 46 : 483 – 490 .
- Sasa , H , Akiyama , H , Shimoda , N and Kawate , S . 2004 . Plant waste recycling technology with steam treatment . Ishikawajima-Harima Engineering Rev , 44 : 338 – 344 . Ishikawajima-Harima Heavy Industries, (in Japanese with English summary)
- Shuman , LM . 2003 . Fertilizer source effects on phosphate and nitrate leaching through simulated golf greens . Environ. Pollut , 125 : 413 – 421 .
- Tabatabai , MA and Bremer , JM . 1969 . Use of p-nitrophenyl phosphate for assay of soil phosphatase activity . Soil Biol. Biochem , 1 : 301 – 307 .
- Ushiwata , SY and Inubushi , K . 2005 . Influence of plant residues after steam-treatment with high temperature and pressure on soil microbial biomass C and N, water-soluble C and N, and pH . Soil Sci. Plant Nutr , 51 : 775 – 777 .
- Vance , ED , Brookes , PC and Jenkison , DS . 1987 . An extraction method for measuring soil microbial biomass C . Soil Biol. Biochem , 19 : 703 – 707 .
- Wong , JWC , Chan , CWY and Cheung , KC . 1998 . Nitrogen and phosphorus leaching from fertilizer applied on golf course: lysimeter study . Water, Air, Soil Pollut , 107 : 335 – 345 .
- Zachary , ME and Petrovic , AM . 2004 . Fertilizer source effect on ground and surface water quality in drainage from turfgrass . J. Environ. Qual , 33 : 645 – 655 .
- Zaman , M , Di , HJ , Sakamoto , K , Goto , S , Hayashi , H and Inubushi , K . 2002 . Effects of sewage sludge compost and chemical fertilizer application on microbial biomass and N mineralization rates . Soil Sci. Plant Nutr , 48 : 195 – 201 .