Abstract
Blue light has been suggested to participate in the acclimation of photosynthesis to growth irradiance. We analyzed the effects of blue light intensity on the photosynthetic properties of leaves with regard to acclimation to irradiance. Spinach (Spinacia oleracea L.) plants were grown under mixtures of blue and red light with blue-light photon flux densities (PFDs) of 0, 30, 100 and 150 µmol m−2 s−1 at a total photosynthetic PFD of 300 µmol m−2 s−1. The light-saturated rate of photosynthesis under white light, leaf N content per unit leaf area, leaf dry weight per unit leaf area and the ratio of cytochrome (Cyt) f content to light-harvesting chlorophyll-binding protein of photosystem II (LHCII) content were evaluated. The photosynthetic rate tended to increase with increasing blue-light PFD up to 100 µmol m−2 s−1, and this was associated with an increase in leaf N content per unit leaf area. However, the increase in leaf N content per unit leaf area did not necessarily result from an increase in leaf dry weight per unit leaf area. The Cyt f to LHCII content ratio increased linearly with increasing blue-light PFD up to 100 µmol m−2 s−1, indicating that plants grown under higher blue-light PFD up to this value resembled plants grown under higher irradiance in terms of N partitioning between electron-transport components and light-harvesting components. This result suggests that the level of blue light is involved only in the acclimation to relatively low growth irradiances at the chloroplast level.
INTRODUCTION
Blue light strongly affects the growth and development of higher plants. There have been many studies on blue-light responses, including photomorphogenesis, mediated by blue-light photoreceptors (CitationBriggs and Huala 1999; CitationLin 2000). In addition, blue light influences leaf photosynthetic properties. CitationLeong and Anderson (1984a) found that Atriplex triangularis plants grown under blue light showed a higher rate of leaf photosynthesis under light-saturating conditions than those plants grown under red light. Plants grown under blue light have a higher chlorophyll (Chl) a/b ratio (CitationLeong and Anderson 1984a; CitationLichtenthaler et al. 1980; CitationLópez-Juez and Hughes 1995), a greater cytochrome (Cyt) f content (CitationLeong and Anderson 1984a; CitationLópez-Juez & Hughes 1995) and a greater ribulose-1,5-bisphosphate carboxylase/oxygenase (Rubisco) content (CitationEskins et al. 1991; CitationLópez-Juez and Hughes 1995) than plants grown under red light. The photosynthetic properties of leaves developing under blue light are similar to those acclimating to high irradiance. In addition, CitationWalters and Horton (1995) found that neither the light-saturated rate of photosynthetic O2 evolution nor the Chl a/b ratio in Arabidopsis thaliana leaves were affected by growth irradiance of red light completely deficient in blue light. Considering these results, several authors suggested that blue light is involved in the acclimation of leaf photosynthesis to growth irradiance (CitationAnderson et al. 1995; CitationSenger and Bauer 1987; CitationWalters 2005).
In most previous studies, the effects of blue-enriched light have been compared with those of red-enriched light at an equal photosynthetic photon flux density (PPFD) using colored fluorescent lamps. It is not known whether plants perceive only the presence of weak blue light or use the absolute level of blue light as a signal to induce acclimation responses to growth irradiance. Thus, it is unclear how the level of blue light in irradiated light is related to the changes in photosynthetic properties during the acclimation to growth irradiance. An analysis of this relationship would contribute to clarifying the mechanism of the acclimation of plants to growth irradiance.
In the present study, we grew spinach plants under mixtures of blue and red light at an equal total PPFD (300 µmol m−2 s−1) with different blue-light photon flux densities (PFDs) (0, 30, 100 and 150 µmol m−2 s−1). Blue and red light-emitting diodes (LED) were used as the light source so that the blue-light PFD could be controlled independently of total PPFD (CitationMatsuda et al. 2004; CitationOhashi-Kaneko et al. 2006). The acclimation of leaf photosynthesis to growth irradiance has been well characterized in terms of changes in the light-saturated rate of photosynthesis, leaf N content and N partitioning among photosynthetic components within chloroplasts (CitationAnderson 1986; CitationBjörkman 1981; CitationBoardman 1977). We determined the photosynthetic rate under saturating white light and the amounts of N and some photosynthetic components in young, fully expanded leaves developing under various blue-light PFDs. Then, we evaluated quantitatively how blue-light intensity was related to changes in the photosynthetic properties of leaves and we discuss the relationships with regard to the acclimation of leaf photosynthesis to growth irradiance.
MATERIALS AND METHODS
Plant material and growth conditions
Seeds of spinach (Spinacia oleracea L., cv. Megaton) plants were sown in vermiculite and grown under white fluorescent lamps in an environmentally controlled chamber for 7 days. The chamber was first operated with 150 µmol m−2 s−1 PPFD at the tops of the plants during a 12-h photoperiod and 25 ± 1/20 ± 1°C day/night temperatures. Seven days after germination, each seedling was transplanted to a 0.5-L plastic bottle containing a nutrient solution. The seedlings were then grown hydroponically in environmentally controlled chambers equipped with an LED array (Iwasaki Electric, Tokyo, Japan). The LED array was composed of 1296 red and 1296 blue LED chips. From 7 days after germination, the plants were grown under mixtures of blue and red light with four levels of blue-light PFD. The blue-light and red-light PFDs were 0 and 300, 30 and 270, 100 and 200 and 150 and 150 µmol m−2 s−1, respectively. Total PPFD was 300 µmol m−2 s−1 for any lighting treatment. Nine seedlings were subjected to each lighting treatment. The chambers were maintained with 12 h photoperiod and 20 ± 1/15 ± 1°C day/night temperatures. The basal nutrient solution used was prepared according to CitationMakino et al. (1988) except that a N concentration of 8.0 mmol L−1 (6.0 mmol L−1 NaNO3 plus 1.0 mmol L−1 NH4NO3) was used. The nutrient solution was renewed every 5 days and was continuously aerated. The strength of the nutrient solution was varied depending on plant growth (days after germination): half strength at 7 days and full strength from 12 days. Gas-exchange measurements and biochemical assays were carried out on young, fully expanded leaves of 32-day-old to 47-day-old plants.
Light environment measurements
The PPFD was measured using a quantum sensor (LI-190SA with LI-250, LI-COR, Lincoln, NE, USA). Spectral PFD distributions for the lighting treatments () were calculated from spectral energy distributions, which were measured using a spectroradiometer (HR2000, Ocean Optics, Dunedin, FL, USA). The spectral energy distributions were measured every 0.4–0.5 nm. We calculated phytochrome photoequilibrium (Pfr/Ptotal) from the spectral PFD distribution for each lighting treatment with the photoconversion cross section of phytochrome according to CitationSager et al. (1988). The Pfr/Ptotal represents the fraction of the active form of phytochrome in the total phytochrome possessing red/far-red reversibility. The Pfr/Ptotal ratio for all lighting treatments was almost identical (0.87–0.88). This means that differences in the reversible action of phytochrome were negligible under the conditions of this study.
Gas-exchange measurements
Gas-exchange rates were measured using a portable gas-exchange measurement system (LI-6400, LI-COR). Light was provided from a 35 W white halogen lamp. Measurements were made at a PPFD of 1,800 µmol m−2 s−1, an atmospheric CO2 partial pressure of 36 Pa, a leaf temperature of 25°C and a leaf-to-air vapor pressure deficit of 1.1 ± 0.1 kPa.
Biochemical assays
A single leaf was homogenized with a chilled mortar and pestle in 50 mmol L−1 Na-phosphate buffer (pH 7.0) containing 2 mmol L−1 Na-iodoacetate, 0.8% (v/v) 2-mercaptoethanol and 5% (v/v) glycerol. The leaf N,
Figure 1 Spectral photon flux density (PFD) distributions for the lighting treatments. Blue-light PFDs were (a) 0, (b) 30, (c) 100 and (d) 150 µmol m−2 s−1. Total photosynthetic photon flux density was 300 µmol m−2 s−1 in any lighting treatment. The PFD was measured every 0.4–0.5 nm.
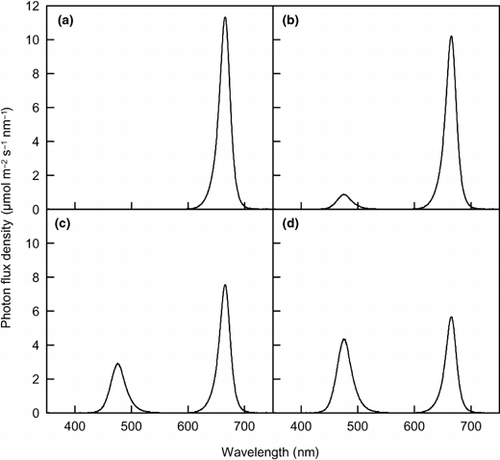
RESULTS AND DISCUSSION
Plants grown under high irradiance exhibit a higher rate of light-saturated photosynthesis at a normal CO2 level associated with a greater leaf N content per unit leaf area (LA) than those grown under low irradiance (CitationHirose and Werger 1987; CitationMakino et al. 1997; CitationSims and Pearcy 1989). The light-saturated rate of photosynthesis per unit LA at an ambient partial pressure of 36 Pa CO2 under white light tended to increase as the blue-light PFD increased, up to 100 µmol m−2 s−1, while the rate at 150 µmol m−2 s−1 was nearly identical to that at 100 µmol m−2 s−1 (). This increase in photosynthetic rate did not result from an increase in stomatal conductance because intercellular CO2 partial pressure was independent of blue-light PFD during growth (data not shown). Leaf N content per unit LA also increased with increasing blue-light PFD except at 150 µmol m−2 s−1, where it tended to decrease slightly from that at 100 µmol m−2 s−1 (). Thus, in the range between 0 and 100 µmol m−2 s−1, plants grown under higher blue-light PFD resembled those grown under higher irradiance in terms of the capacity for photosynthesis as well as N content on an LA basis. The dependency of Rubisco content per unit LA, a determinant for the light-saturated rate of photosynthesis at normal CO2 levels (CitationMakino et al. 1985), on blue-light PFD was similar to that of leaf N content per unit LA (data not shown).
In general, the greater leaf N content per unit LA in plants grown under high irradiance is almost accounted for by a greater leaf dry weight (DW) per unit LA, rather than by a greater leaf N content per unit DW (CitationEvans 1996; CitationEvans and Poorter 2001; CitationSims and Pearcy 1994). This means that, in acclimation to growth irradiance, the change in leaf N content on an LA basis mainly results from morphological alteration. However, the change in leaf N content per unit LA depending on the blue-light PFD was not correlated with changes in leaf DW per unit LA (). Both leaf DW per unit LA
Figure 2 The relationship between (a) the light-saturated rate of photosynthesis per unit leaf area (LA) and (b) leaf N content per unit LA as functions of blue-light photon flux density in spinach leaves. Gas-exchange measurements were made at a photosynthetic photon flux density of 1,800 µmol m−2 s−1, an ambient CO2 partial pressure of 36 Pa, a leaf temperature of 25°C and a leaf-to-air vapor pressure deficit of 1.1 ± 0.1 kPa. Light for measurements was provided from a white halogen lamp. Error bars represent standard error (n ≥ 4). Means with different letters are significantly different using Tukey's honestly significant difference test (P < 0.05).
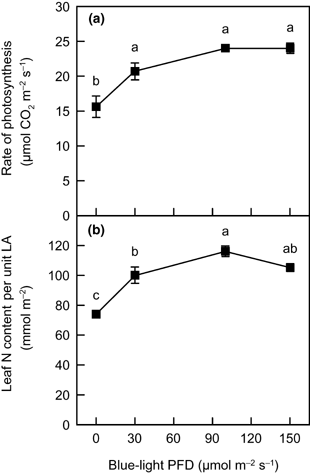
When plants acclimate to growth irradiance, changes are observed not only in the leaf N content on an LA
Figure 3 The relationship between (a) leaf dry weight (DW) per unit leaf area (LA) and (b) leaf N content per unit DW as functions of blue-light photon flux density in spinach leaves. Error bars represent standard error (n ≥ 6). Means with different letters are significantly different using Tukey's honestly significant difference test (P < 0.05).
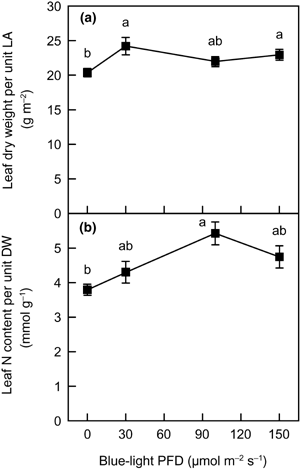
Figure 4 The relationships between (a) cytochrome (Cyt) f, (b) light-harvesting chlorophyll-binding protein of photosystem II (LHCII) and (c) chlorophyll (Chl) content per unit leaf area (LA) and (d) Chl a/b ratio as functions of blue-light photon flux density in spinach leaves. Error bars represent standard error (n ≥ 3). Means with different letters are significantly different using Tukey's honestly significant difference test (P < 0.05).
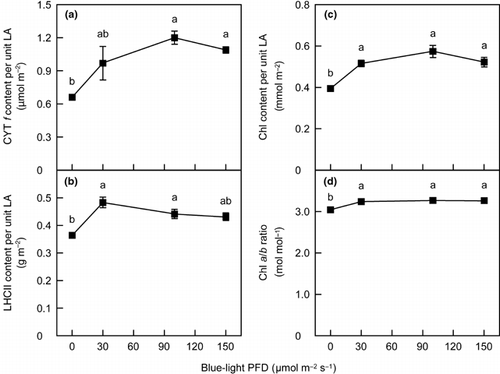
Figure 5 The relationship between the ratio of cytochrome (Cyt) f content to light-harvesting chlorophyll-binding protein of photosystem II (LHCII) content as a function of blue-light photon flux density in spinach leaves. Eror bars represent standard error (n = 3). Means with different letters are significantly different using Tukey's honestly significant difference test (P < 0.05).
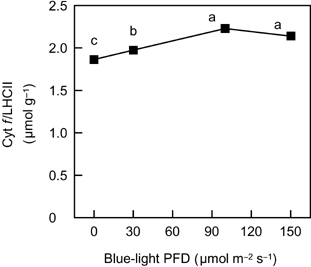
In conclusion, the level of blue light in a low-PFD range (below 100 µmol m−2 s−1 under the conditions of this study) appears to be related to the alteration in N partitioning among thylakoid components to growth irradiance. In contrast, blue light took little part in the change in leaf DW per unit LA, even though the change in leaf DW per unit LA is important in changing leaf N content and the light-saturated rate of photosynthesis per unit LA during the acclimation to irradiance. Several authors suggested that responses to growth irradiance at the chloroplast level and at the leaf morphological level could be regulated by different mechanisms (CitationMurchie and Horton, 1998; CitationWeston et al. 2000; CitationYano and Terashima 2001). The level of blue light may be involved only in the chloroplast-level acclimation to relatively low growth irradiances.
REFERENCES
- Anderson , JM . 1986 . Photoregulation of the composition, function, and structure of thylakoid membranes . Ann. Rev. Plant Physiol , 37 : 93 – 136 .
- Anderson , JM , Chow , WS and Park , YI . 1995 . The grand design of photosynthesis: acclimation of the photosynthetic apparatus to environmental cues . Photosynth. Res , 46 : 129 – 139 .
- Bendall , DS , Davenport , HE and Hill , R . 1971 . Cytochrome components in chloroplasts of the higher plants . Methods Enzymol , 33 : 327 – 344 .
- Björkman , O . 1981 . “ Responses to different quantum flux densities ” . In Physiological Plant Ecology I. Responses to the Physical Environment , Edited by: Lange , OL , Nobel , PS , Osmond , CB and Ziegler , H . 57 – 107 . Berlin : Springer-Verlag .
- Boardman , NK . 1977 . Comparative photosynthesis of sun and shade plants . Ann. Rev. Plant Physiol , 28 : 355 – 377 .
- Briggs , WR and Huala , E . 1999 . Blue-light photoreceptors in higher plants . Ann. Rev. Cell Dev. Biol , 15 : 33 – 62 .
- De la Torre , WR and Burkey , KO . 1990a . Acclimation of barley to changes in light intensity: chlorophyll organization . Photosynth. Res , 24 : 117 – 125 .
- De la Torre , WR and Burkey , KO . 1990b . Acclimation of barley to changes in light intensity: photosynthetic electron transport activity and components . Photosynth. Res , 24 : 127 – 136 .
- Eskins , K , Jiang , CZ and Shibles , R . 1991 . Light-quality and irradiance effects on pigments, light-harvesting proteins and Rubisco activity in a chlorophyll- and light-harvesting-deficient soybean mutant . Physiol. Plant , 83 : 47 – 53 .
- Evans , JR . 1987 . The relationships between electron transport components and photosynthetic capacity in pea leaves grown at different irradiances . Aust. J. Plant Physiol , 14 : 157 – 170 .
- Evans , JR . 1996 . “ Developmental constraints on photosynthesis: effects of light and nutrition ” . In Advances in Photosynthesis Vol. 5. Photosynthesis and the Environment , Edited by: Baker , NR . 281 – 304 . Dordrecht : Kluwer Academic Publishers .
- Evans , JR and Poorter , H . 2001 . Photosynthetic acclimation of plants to growth irradiance: the relative importance of specific leaf area and nitrogen partitioning in maximizing carbon gain . Plant Cell Environ , 24 : 755 – 767 .
- Hidema , J , Makino , A , Kurita , Y , Mae , T and Ojima , K . 1992 . Changes in the levels of chlorophyll and light-harvesting chlorophyll a/bprotein of PS II in rice leaves aged under different irradiances from full expansion through senescence . Plant Cell Physiol , 33 : 1209 – 1214 .
- Hikosaka , K . 2005 . Nitrogen partitioning in the photosynthetic apparatus of Plantago asiatica leaves grown under different temperature and light conditions: similarities and differences between temperature and light acclimation . Plant Cell Physiol , 46 : 1283 – 1290 .
- Hirose , T and Werger , MJA . 1987 . Nitrogen use efficiency in instantaneous and daily photosynthesis of leaves in the canopy of a Solidago altissima stand . Physiol. Plant , 70 : 215 – 222 .
- Leong , TY and Anderson , JM . 1984a . Effect of light quality on the composition and function of thylakoid membranes in Atriplex triangularis . Biochim. Biophys. Acta , 766 : 533 – 541 .
- Leong , TY and Anderson , JM . 1984b . Adaptation of the thylakoid membranes of pea chloroplasts to light intensities. I. Study on the distribution of chlorophyll–protein complexes . Photosynth. Res , 5 : 105 – 115 .
- Leong , TY and Anderson , JM . 1984c . Adaptation of the thylakoid membranes of pea chloroplasts to light intensities. II. Regulation of electron transport capacities, electron carriers, coupling factor (CF1) activity and rates of photosynthesis . Photosynth. Res , 5 : 117 – 128 .
- Lichtenthaler , HK , Buschmann , C and Rahmsdorf , U . 1980 . “ The importance of blue light for the development of sun-type chloroplasts ” . In The Blue Light Syndrome , Edited by: Senger , H . 485 – 494 . Berlin : Springer-Verlag .
- Lin , C . 2000 . Plant blue-light receptors . Trends Plant Sci , 5 : 337 – 342 .
- López-Juez , E and Hughes , MJG . 1995 . Effect of blue light and red light on the control of chloroplast acclimation of light-grown pea leaves to increased fluence rates . Photochem. Photobiol , 61 : 106 – 111 .
- Makino , A , Mae , T and Ohira , K . 1985 . Photosynthesis and ribulose-1,5-bisphosphate carboxylase/oxygenase in rice leaves from emergence through senescence. Quantitative analysis by carboxylation/oxygenation and regeneration of ribulose-1,5-bisphosphate . Planta , 166 : 414 – 420 .
- Makino , A , Mae , T and Ohira , K . 1988 . Differences between wheat and rice in the enzymic properties of ribulose-1,5-bisphosphate carboxylase/oxygenase and the relationship to photosynthetic gas exchange . Planta , 174 : 30 – 38 .
- Makino , A , Nakano , H and Mae , T . 1994 . Responses of ribulose-1,5-bisphosphate carboxylase, cytochrome f, and sucrose synthesis enzymes in rice leaves to leaf nitrogen and their relationships to photosynthesis . Plant Physiol , 105 : 173 – 179 .
- Makino , A , Sato , T , Nakano , H and Mae , T . 1997 . Leaf photosynthesis, plant growth and nitrogen allocation in rice under different irradiances . Planta , 203 : 390 – 398 .
- Matsuda , R , Ohashi-Kaneko , K , Fujiwara , K , Goto , E and Kurata , K . 2004 . Photosynthetic characteristics of rice leaves grown under red light with or without supplemental blue light . Plant Cell Physiol , 45 : 1870 – 1874 .
- Miyake , C , Horiguchi , S , Makino , A , Shinzaki , Y , Yamamoto , H and Tomizawa , KI . 2005 . Effects of light intensity on cyclic electron flow around PSI and its relationship to non-photochemical quenching of Chl fluorescence in tobacco leaves . Plant Cell Physiol , 46 : 1819 – 1830 .
- Murchie , EH and Horton , P . 1998 . Contrasting patterns of photosynthetic acclimation to the light environment are dependent on the differential expression of the responses to altered irradiance and spectral quality . Plant Cell Environ , 21 : 139 – 148 .
- Ohashi , K , Makino , A and Mae , T . 1998 . Gas exchange characteristics in rice leaves grown under the conditions of physiologically low temperature and irradiance . Plant Cell Physiol , 39 : 1384 – 1387 .
- Ohashi-Kaneko , K , Matsuda , R , Goto , E , Fujiwara , K and Kurata , K . 2006 . Growth of rice plants under red light with or without supplemental blue light . Soil Sci. Plant Nutr , 52 : 444 – 452 .
- Sager , JC , Smith , WO , Edwards , JL and Cyr , KL . 1988 . Photosynthetic efficiency and phytochrome photoequilibria determination using spectral data . Trans. ASAE , 31 : 1882 – 1889 .
- Senger , H and Bauer , B . 1987 . The influence of light quality on adaptation and function of the photosynthetic apparatus . Photochem. Photobiol , 45 : 939 – 946 .
- Sims , DA and Pearcy , RW . 1989 . Photosynthetic characteristics of a tropical forest understory herb, Alocasia macrorrhiza, and a related crop species, Colocasia esculenta, grown in contrasting light environments . Oecologia , 79 : 53 – 59 .
- Sims , DA and Pearcy , RW . 1994 . Scaling sun and shade photosynthetic acclimation of Alocasia macrorrhiza to whole-plant performance – I. Carbon balance and allocation at different daily photon flux densities . Plant Cell Environ , 17 : 881 – 887 .
- Terashima , I and Evans , JR . 1988 . Effects of light and nitrogen nutrition on the organization of the photosynthetic apparatus in spinach . Plant Cell Physiol , 29 : 143 – 155 .
- Walters , RG . 2005 . Towards an understanding of photosynthetic acclimation . J. Exp. Bot , 411 : 435 – 447 .
- Walters , RG and Horton , P . 1995 . Acclimation of Arabidopsis thaliana to the light environment: regulation of chloroplast composition . Planta , 197 : 475 – 481 .
- Weston , E , Thorogood , K , Vinti , G and López-Juez , E . 2000 . Light quantity controls leaf-cell and chloroplast development in Arabidopsis thaliana wild type and blue-light-perception mutants . Planta , 211 : 807 – 815 .
- Yano , S and Terashima , I . 2001 . Separate localization of light signal perception for sun and shade type chloroplast and palisade tissue differentiation in Chenopodium album . Plant Cell Physiol , 42 : 1303 – 1310 .