Abstract
The phylogenetic positions of bacterial communities in manganese (Mn) nodules from subsoils of two Japanese rice fields were estimated using polymerase chain reaction-denaturing gradient gel electrophoresis (PCR-DGGE) analysis followed by sequencing of 16S rDNA. The DGGE band patterns and sequencing analysis of characteristic DGGE bands revealed that the bacterial communities in Mn nodules were markedly different from those in the plow layer and subsoils. Three out of four common bands found in Mn nodules from two sites corresponded to Deltaproteobacteria and were characterized as sulfate-reducing and iron-reducing bacteria. The other DGGE bands of Mn nodules corresponded to sulfate and iron reducers (Deltaproteobacteria), methane-oxidizing bacteria (Gamma and Alphaproteobacteria), nitrite-oxidizing bacteria (Nitrospirae) and Actinobacteria. In addition, some DGGE bands of Mn nodules showed no clear affiliation to any known bacteria. The present study indicates that members involved in the reduction of Mn nodules dominate the bacterial communities in Mn nodules in rice field subsoils.
INTRODUCTION
Rice is a staple crop in Asian countries. Irrigated rice fields, which account for more than 50% of the total areas managed for rice production (CitationHalwart and Gupta 2004), provide a unique environment for soil microorganisms because the soils are maintained under flooded conditions during the main stages of rice growth and left to drain after rice harvesting. Flooding the fields results in soil reduction of the plow layer, and the resultant Fe2+ and Mn2+ ions in the plow layer are leached by water percolation and accumulate in the subsoil as exchangeable or oxidized forms at specific soil depths, all of which are finally oxidized after the rice fields are drained (CitationKimura 2000).
The sites of Fe and Mn accumulation in the subsoil layer are termed Fe and Mn illuvial horizons, and the accumulation sites of Fe oxides are slightly shallower than those of Mn oxides as predicted from the redox behavior of these two elements (CitationKyuma 2004). They are horizons specific to irrigated rice fields, and usually recorded of their depths in the soil classification of rice fields. The morphology of illuviated Fe and Mn differs: Fe oxides form cloud-like ferruginous mottles at the horizon, whereas Mn oxides form spot-like manganiferous mottles at the Mn illuvial horizon.
Phylogenetically wide varieties of bacteria have been isolated from versatile environments, including those environments involving in Mn oxidation and reduction processes. To the best of our knowledge, these varieties are Aerobacter sp., Arthrobacter spp., Bacillus spp., Caulobacter sp., Chromobacterium spp., Citrobacter sp., Corynebacterium sp., Cytophaga sp., Gallionella sp., Hyphomicrobium sp., Leptothrix spp., Pedomicrobium sp., Pseudomonas spp., Proteus sp. and Streptomyces sp. as Mn-oxidizing bacteria from Mn nodules (the Atlantic Ocean), Mn concretions (soil), lake waters, groundwater, bay sediments and soils (e.g. CitationBromfield and Skerman 1950; CitationEhrlich 1968; CitationFrancis and Tebo 2001; CitationGregory and Staley 1982; CitationWada et al. 1978b), and Acinetobacter spp., Bacillus spp., Carnobacterium sp., Corynebacterium sp., Desulfovibrio sp., Desulfotomaculum spp., Geobacter sp., Pseudomonas spp., Pyrobaculum sp. and Shewanella sp. as Mn-reducing bacteria from freshwater sediments, marine sediments, Mn nodules (the Atlantic Ocean) and aquifers (e.g. CitationDi-Ruggiero and Gounot 1990; CitationGhiorse and Ehrlich 1974; CitationLovley et al. 1989; CitationSass and Cypionka 2004). However, there are only two papers reporting microbial communities associated with ferromanganese nodules/deposits in Green Bay (CitationStein et al. 2001) and Lechuguilla and Spider Caves (CitationNorthup et al. 2003). And no reports on bacterial communities inhabiting Mn nodules in paddy fields have been published to date.
The present study aimed to elucidate the bacterial communities inhabiting Mn nodules in the subsoil layer of Japanese rice fields using molecular techniques of polymerase chain reaction-denaturing gradient gel electrophoresis (PCR-DGGE) and sequencing. The PCR-DGGE analysis has been found to be a suitable method for detecting microbial community structure from environmental samples (CitationCahyani et al. 2003, Citation2004a, Citationb). This is the first study to examine the bacterial communities in Mn mottles/nodules in the Mn illuvial horizons of rice fields.
MATERIALS AND METHODS
Collection of manganese nodules
Several kilograms of soil blocks were collected from Mn illuvial horizons in two Japanese rice fields located in Kojima Bay reclamation area, Okayama Prefecture, on 16 March 2006. Sites A and B were located in Fujita Omagari (34°34′N, 133°51′E) and Fujita Nishiki-rokku (34°35′N, 133°54′E), respectively. In addition, plow layer soil and subsoil samples apart from Mn nodules were also taken as references. Some of the soil properties at Sites A and B are as follows: (1) plow layer soils: total C content 16 and 17.1 g kg−1, respectively; total N content 1.4 and 1.4 g kg−1, respectively; pH (H2O) 6.6 and 6.4, respectively; cation exchange capacity (CEC) 20.1 and 18.8 cmolc kg−1, respectively, (2) subsoils: total C content 5.6 and 12.8 g kg−1, respectively; total N content 0.5 and 1.2 g kg−1, respectively; pH (H2O) 6.8 and 6.6, respectively; CEC 17.4 and 18.5 cmolc kg−1, respectively.
In the laboratory, approximately 15 g of large and firm Mn nodules (several millimeters in diameter) were collected carefully with forceps and a small spatula by the breaking soil blocks into small pieces. The nodules were immersed into distilled water and sonicated for a minute to disperse clinging soil particles from the nodules. This washing was repeated several times to obtain clean Mn nodules. The nodules were then stored at −80ºC until use.
Chemical analysis of manganese nodules
Concentrations of Mn and Fe in the Mn nodules and in the reference soil samples were determined using an inductively coupled plasma (ICP) spectrophotometer (Model IRIS AP, Nippon Jarrell-Ash, Kyoto, Japan). Air-dried samples were pulverized using a mortar and a pestle. Approximately 100 mg of the samples was placed in a 25-mL glass tube and 10 mL of 50 g kg−1 hydroxylamine in 1 mol L−1 HCl was added. The glass tube was incubated in a water bath at 70°C for 3 h. Five milliliters of 50 g kg−1 hydroxylamine in 1 mol L−1 HCl was added again to the tube, and incubation continued at 70°C for 3 h, when sample residues turned from dark brown to pale brown. The sample was transferred to a 50-mL volumetric flask after cooling it at room temperature and filled up to 50 mL with 1 mol L−1 HCl. The sample was left to stand overnight at room temperature. Then, 10 mL of supernatant was carefully removed to a 10-mL glass tube so as not to disturb settled residues and subjected to the determination of Mn and Fe concentrations using an ICP spectrophotometer.
DNA extraction
The DNA was extracted from approximately 400 mg of Mn nodule and reference samples after melting them using FastDNA SPIN Kit for Soil (BIO 101, Qbiogene, Carlsbad, CA, USA) according to the manufacturer's protocols. The DNA was eluted from the binding matrix with 100 µL of TE buffer (10 mmol L−1 Tris-HCl (pH 8.0), 1 mmol L−1 ethylenediaminetetraacetic acid (EDTA)) and stored at –20°C. The DNA extraction was carried out on three replicates for each sample.
Polymerase chain reaction amplification
The eubacterial sets of two primers 357f-GC clamp and 517r were applied to amplify the 16S rDNA fragments. The DNA sequences of primers 357f-GC clamp (Escherichia coli position: 341-357, 5′-CGCCCGCCGCGCGCGGCGGGCGGGGCGGGGGCACGGCCTACGGGAGGCA GCAG-3′, the underlined sequence corresponded to the GC clamp) and 517r (5′-ATTACCGCGGCTGC TGG-3′, E. coli position: 517–534) (CitationMuyzer et al. 1993) were used. The primers were specific for the V3 variable region of eubacterial 16S rDNA (CitationNeefs et al. 1990). Three replicates of DNA extract from each sample were subjected to PCR amplification. The PCR was carried out with TaKaRa PCR Thermal Cycles Model TP 240 (TaKaRa, Tokyo, Japan). The total volume of the reaction mixture was 50 µL containing 0.5 µL of each primer (50 pmol each), 5 µL of 2.5 mmol L−1 dNTP mixture, 5 µL of 10×Ex Taq buffer (20 mmol L−1 Mg2+; TaKaRa), 0.25 µL of Ex Taq DNA polymerase (TaKaRa), 1 µL of environmental DNA template and 37.75 µL of milli-Q water. Cycle conditions for the PCR amplification were as follows: the first path was one cycle of initial denaturation at 94°C for 3 min, followed by a second path of 35 cycles of denaturation at 94°C for 1 min, annealing at 55°C for 1 min and extension at 72°C for 1.5 min, and the third path was one cycle of final extension at 72°C for 8 min.
DGGE analysis, direct sequencing and sequencing after cloning
The DGGE analysis was carried out by loading the PCR products onto an 80 g L−1 polyacrylamide gel with a denaturing gradient ranging from 30 to 70% in an electrophoresis cell D-code System (Bio-Rad Laboratories, Hercules, CA, USA) with 1× TAE buffer (40 mmol L−1 Tris, 20 mmol L−1 acetic acid and 1 mmol L−1 ethylenediaminetetraacetic acid [EDTA] at pH 8.0) at 60°C and 100 V for 14 h. The procedure from the preparation of the gel to the visualization of the DGGE band pattern was the same as that described previously (CitationCahyani et al. 2003). Most bands with strong intensity in the lane of Mn nodule samples in the DGGE gel were readily isolated and sequenced directly. However, bands with weak intensity in the lane and all bands in the lanes of reference soils (subsoil and plow layer) were unable to be sequenced directly. Therefore, cloning was carried out for those bands before sequencing. The procedures of direct sequencing and sequencing after cloning were the same as those described previously (CitationCahyani et al. 2003). In the DGGE pattern analysis, we first compared the DGGE patterns among three replicates of each sample, and found exactly the same patterns among them. Respective bands were excised from three replicates for the determination of their sequences. However, we presented the DGGE pattern of each sample without replication in .
Phylogenetic analysis
Sequences of the DGGE bands were compared with the available 16S rDNA sequences from the database of the DNA Data Bank of Japan (DDBJ; http://www.ddbj.nig.ac.jp/E-mail/homology.html) using the BLAST search.
Nucleotide sequence accession numbers
The 16S rDNA partial sequences determined in the present study have been deposited in the DDBJ database under the accession numbers AB292482–AB292524.
RESULTS AND DISCUSSION
Chemical properties of manganese nodules
Manganese nodules from site A and site B contained 31 and 42 g Mn kg−1 of soil and 22 and 38 g Fe kg−1 of soil,
Figure 1 Denaturing gradient gel electrophoresis pattern of the bacterial communities in Mn nodules and the reference soil samples in rice field soils. A, Mn nodules at site A; B, Mn nodules at site B; PA, plow layer soil of site A; SA, subsoil of site A; SB, subsoil of site B. Sites A and B were located in Fujita Omagari and Fujita Nishiki-rokku, respectively.
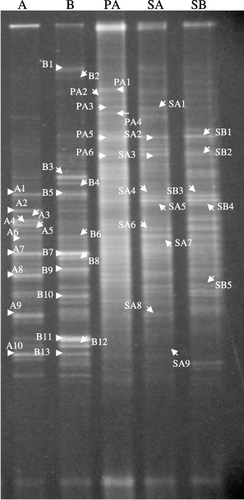
Table 1 Concentrations on a dry weight basis of Mn and Fe in Mn nodules and the reference soils in rice fields
Bacterial communities in Mn nodules
Bacterial communities in Mn nodules are represented by the DGGE band patterns shown in . The DGGE patterns of Mn nodules from both sites were characterized by several strong bands, indicating that several specific bacteria inhabit the Mn nodules. The DGGE patterns of Mn nodules from the two sites showed similar patterns, and several DGGE bands in Mn nodules from site A and site B were located at the same positions in polyacrylamide gel, indicating that common bacteria inhabit Mn nodules at these sites.
In contrast, the DGGE patterns of the reference soils at both sites consisted of many weak bands, especially for the plow layer soil at site A, indicating that microbial communities in the plow layer and subsoils consist of a wide range of bacteria without any specific bacteria as dominant members (). A similar finding of numerous DGGE bands was also observed in the plow layer soils of other Japanese rice fields (CitationH. Kikuchi et al. 2007). There was no band of strong intensity in the plow layer and subsoil samples that showed the same mobility as the DGGE bands of strong intensity observed in the Mn nodules (). Therefore, we concluded that bacterial communities in Mn nodules are markedly different from those in the plow layer and subsoil.
Representative bacteria in Mn nodules
The closest relatives of some representative bands in the Mn nodules from Sites A and B are listed in . Four respective band pairs A1 and B5, A6 and B6, A7 and B7, and A10 and B13 (prefix A from site A and prefix B from site B) that showed equivalent mobility belonged to the same closest relatives, respectively. Bands A1 and B5 corresponded to Betaproteobacteria, and Bands A6, B6, A7, B7, A10 and B13 to Deltaproteobacteria. This finding also indicated that common bacteria inhabit Mn nodules at these two sites. The members of Deltaproteobacteria were also found to be common bacteria in ferromanganous nodules in Green Bay (CitationStein et al. 2001). However, no Deltapropteobacterial member was detected in ferromanganese deposits in Lechuguilla and Spider Caves (CitationNorthup et al. 2003). Thus, the members of Deltaproteobacteria seemed to be bacteria commonly inhabiting Mn nodules in aquatic environments where the rapid cycling of oxidation and reduction occurs. The closest relatives of the common bacteria represented by DGGE bands in the present study mainly originated from aquatic environments (freshwater sediment, groundwater, marine sediment, lake and paddy soil).
Although many studies have reported Mn oxidizing or reducing bacteria belonging to Gram-negative Proteobacteria, these closest relatives were not found in the present study. As the closest relatives conduct anaerobic respiration: sulfate respiration by Olavius crassitunicatus, Desulfocapsa sp. and Desulfovibrio alaskensis (Bands A6, B6, A10, and B13) and iron oxide respiration by Anaeromyxobacter sp. (A7 and B7), the bacteria of those DGGE bands may contribute to the reduction of Mn oxides because produced sulfides and ferrous iron reduce Mn oxides chemically (CitationMurase and Kimura 1997). Many studies also revealed the involvements of some sulfate-reducing and iron-reducing bacteria in biological Mn reduction. For example, Desulfotomaculum reducens (CitationTebo and Obraztsova 1998), Desulfovibrio indonesiensis and Desulfotomaculum geothermicum (CitationSass and Cypionka 2004) used Mn oxides, in addition to various sulfur compounds, as an electron acceptor. And Geobacter metallireducens (CitationLovley et al. 1993) and Shewanella putrefaciens (formerly Alteromonas putrefaciens) (CitationLovley et al. 1989; CitationMyers and Nealson 1990; CitationNealson and Myers 1992) could obtain energy for growth by coupling the oxidation of various electron donors with the dissimilatory reduction of Mn or Fe oxides. The Mn nodules fit to sulfate and iron reducers because substrates for respiration (sulfate and iron oxides) are regenerated chemically by redox reactions with Mn oxides. From these considerations, it is believed that the closest relatives of Bands B3, B4, A8 and B8 belong to sulfate and iron reducers: Thermodesulforhabdus sp., Pelobacter venetianus and Anaeromyxobacter sp.
Bands A2, A3 and A4 were closely related to methane-oxidizing bacteria of Methylococcus capsulatus (CitationDedysh et al. 2004), Methylocystis parvus (CitationHou et al. 1979) and Methylosinus trichosporium (CitationBowman et al. 1993), respectively. The presence of methanotrophs in Mn nodules might be explained by the findings reported by CitationZehnder and Brock (1980) that under anaerobic
Table 2 Closest relatives to denaturing gradient gel electrophoresis bands obtained from Mn nodules at Sites A and B
Table 3 Closest relatives to DGGE bands obtained from reference soil samples at Sites A and B
The involvement of members of Actinobacteria in Mn oxidation has been reported in several studies. However, Bands A9, B11 and B12 belonging to Actinobacteria did not match the listed members. Some bands showed no clear affiliation to any known bacteria (Bands B1, B2, B9 and B10).
Bacteria in plow layer soil and subsoils
It was difficult to excise single DGGE bands from the DGGE gel of plow layer soil and subsoil samples because the DGGE patterns consisted of many weak bands. Furthermore, cloning of some bands from these samples revealed that a seemingly single band contained several DNA fragments with different mobilities in the polyacrylamide gel. Therefore, it was impossible to determine the phylogenetic position of all bacteria in these reference soils from the direct sequence of those DGGE bands.
The closest relatives of some representative bacteria in the plow layer soil and subsoils are listed in . A wide phylogenetic variety of bacteria inhabit these reference soils. It is interesting to note that some bacteria were found commonly as the closest relatives in subsoil samples from Site A and Site B (Bands SA2 and SB1, and Bands SA4, SA5, SB3 and SB4). These common bacteria corresponded to members of Epsilonproteobacteria and Gram-positive bacteria.
Bacteria detected in the reference soils were markedly different from those in Mn nodules. For example, the bacteria belonging to Epsilonproteobacteria (Bands PA1, SA2 and SB1), Spirochaetes (Band PA3) and Chloroflexi (Bands PA2 and SA1) were found in the reference soils, whereas they were not found in the Mn nodules.
Manganese oxidizing and reducing bacteria and microbial communities in Mn nodules
The bacterial communities in Mn nodules consisted of members potentially related to Mn reduction. The predominance of Mn-reducing bacteria and the superficial absence of Mn-oxidizing bacteria in Mn nodules might support the hypothesis of the process of Mn nodule formation proposed by CitationWada et al. (1978a), which is that the process of Mn formation is divided into two stages. In the initial stage, Mn2+ is oxidized by the activities of Mn-oxidizing bacteria, which form tiny dot-shaped Mn deposits. In the later stage, Mn2+ is oxidized non-biologically on the tiny Mn-deposits, resulting in the formation of large Mn deposits (Mn mottles or Mn nodules). As the Mn nodules observed in this study were several millimeters in diameter, the hypothesis suggests that the Mn nodules used in the present study were formed mainly by the abiotic process. And the bacterial communities dominated by members relating to the reduction of Mn nodules were, in part, a result of the utilization of Mn oxides for the regeneration of their electron acceptors.
In this study, we did not find any close relatives of DGGE bands for Mn nodule samples that match the members described previously. This unexpected result may derive from differences in methodology between the method used in the present study and that used for bacterial isolation. The bacteria reported in many previous studies were obtained using cultivation methods that allow the selective proliferation of minor members, which the PCR-DGGE method cannot detect. The present study indicates that Mn-oxidizing bacteria are minor members in abundance in Mn nodules, and that the majority of bacteria in Mn nodules relate directly or indirectly to Mn reduction.
Noteworthy was that many DGGE bands of Mn nodules matched the available DNA database only for short sequences with similarities of less than 95%. Sequences of Bands A5, A6, A7, A10, B3, B4, B6, B7, B8, B9 and B13 were matched for less than 100 bp to their closest relatives. This fact may explain why many bacterial members in Mn nodules have never been cultured or isolated to date. The phylogenetic positions of predominant bacterial community members and their roles in Mn mottle formation in the subsoils of rice fields are interesting topics for future research because the topics are interdisciplinary, bridging soil microbiology and pedology.
ACKNOWLEDGMENTS
This study was supported by the Japan Society for the Promotion of Science (JSPS) Postdoctoral Fellowship Program for Foreign Researchers and the JSPS Grant-in-Aid for Scientific Research. We thank Dr Zhongjun Jia, Mr Takeshi Watanabe and Ms Natsuko Nakayama in our laboratory for their help with the sample collection. Thanks also to Mr Kazuya Nishina, Laboratory of Forest Environment and Resources, Graduate School of Bioagricultural Sciences, Nagoya University, for his technical assistance in the operation of the ICP spectrophotometer.
REFERENCES
- Bowman , JP , Sly , LI , Nichols , PD and Hayward , AC . 1993 . Revised taxonomy of the methanotrophs: description of Methylobacter gen. nov., emendation of Methylococcus, validation of Methylosinus and Methylocystis species, and a proposal that the family Methylococcaceae includes only the group I methanotrophs . Int. J. Syst. Bacteriol , 43 : 735 – 753 .
- Bromfield , SM and Skerman , VBD . 1950 . Biological oxidation of manganese in soils . Soil Sci , 69 : 337 – 347 .
- Cahyani , VR , Matsuya , K , Asakawa , S and Kimura , M . 2003 . Succession and phylogenetic composition of bacterial communities responsible for the composting process of rice straw estimated by PCR-DGGE analysis . Soil Sci. Plant Nutr , 49 : 619 – 630 .
- Cahyani , VR , Matsuya , K , Asakawa , S and Kimura , M . 2004a . Succession and phylogenetic profile of methanogenic archaeal communities during the composting process of rice straw estimated by PCR-DGGE analysis . Soil Sci. Plant Nutr , 50 : 555 – 563 .
- Cahyani , VR , Matsuya , K , Asakawa , S and Kimura , M . 2004b . Succession and phylogenetic profile of eukaryotic communities in the composting process of rice straw estimated by PCR-DGGE analysis . Biol. Fertil. Soils , 40 : 334 – 344 .
- Dedysh , SN , Ricke , P and Liesack , W . 2004 . NifH and NifD phylogenies: an evolutionary basis for understanding nitrogen fixation capabilities of methanotrophic bacteria . Microbiology , 150 ( 5 ) : 1301 – 1313 .
- Di-Ruggiero , J and Gounot , AM . 1990 . Microbial manganese reduction mediated by bacterial strains isolated from aquifer sediments . Microb. Ecol , 20 : 53 – 63 .
- Ehrlich , HL . 1968 . Bacteriology of manganese nodules. II. Manganese oxidation by cell-free extract from a manganese nodule bacterium . Appl.Microbiol , 16 : 197 – 202 .
- Francis , CA and Tebo , BM . 2001 . cumA multicopper oxidase genes from diverse Mn(II)-oxidizing and non-Mn(II)-oxidizing Pseudomonas strains . Appl. Environ. Microbiol , 67 : 4272 – 4278 .
- Ghiorse , WC and Ehrlich , HL . 1974 . Effects of seawater cations and temperature on manganese dioxide-reductase activity in a marine Bacillus . Appl. Microbiol , 28 : 785 – 792 .
- Gregory , E and Staley , JT . 1982 . Widespread distribution of ability to oxidize manganese among freshwater bacteria . Appl. Environ. Microbiol , 44 : 509 – 511 .
- Halwart , M and Gupta , MV , eds. 2004 . Culture of Fish in Rice Fields , Rome : Food and Agriculture Organization and The World Fish Center .
- Hou , CT , Laskin , AI and Patel , RN . 1979 . Growth and polysaccharide production by Methylocystis parvus OBBP on methanol . Appl. Environ. Microbiol , 37 : 800 – 804 .
- Kikuchi , H , Watanabe , T , Jia , Z , Kimura , M and Asakawa , S . 2007 . Bacterial communities in bulk soil of a Japanese paddy field: estimation by denaturing gradient gel electrophoresis of 16S rRNA genes amplified from DNA and RNA . Soil Sci. Plant Nutr , doi: 10.1111/j.1747-0765.2007.00160.
- Kimura , M . 2000 . “ Anaerobic microbiology in waterlogged rice fields ” . In Soil Biochemistry , Edited by: Bollag , JM and Stotzky , G . 35 – 138 . New York : Marcel Dekker . Vol. 10. Eds
- Kyuma , K . 2004 . Paddy Soil Science , Kyoto : Kyoto University Press .
- Lovley , DR . 1991 . Dissimilatory Fe(III) and Mn(IV) reduction . Microbiol. Reviews , 55 : 259 – 287 .
- Lovley , DR , Giovannoni , SJ White , DC . 1993 . Geobacter metallireducens gen. nov. sp. nov., a microorganism capable of coupling the complete oxidation of organic compounds to the reduction of iron and other metals . Arch. Microbiol , 159 : 336 – 344 .
- Lovley , DR , Phillips , EJP and Lonergan , DJ . 1989 . Hydrogen and formate oxidation coupled to dissimilatory reduction of iron or manganese by Alteromonas putrefaciens . Appl. Environ. Microbiol , 55 : 700 – 706 .
- Myers , CR and Nealson , KH . 1990 . Respiration-linked proton translocation coupled to anaerobic reduction of manganese (IV) and iron (III) in Shewanella putrefaciens MR-1 . J. Bacteriol , 172 : 6232 – 6238 .
- Murase , J and Kimura , M . 1997 . Anaerobic reoxidation of Mn2+, Fe2+, S0 and S2 in submerged paddy soils . Biol. Fertil. Soils , 25 : 302 – 306 .
- Muyzer , G , Waal , ECD and Uitterlinden , AG . 1993 . Profiling of complex microbial populations by denaturing gradient gel electrophoresis analysis of polymerase chain reaction-amplified genes coding for 16S rRNA . Appl. Environ. Microbiol , 59 : 695 – 700 .
- Nealson , KH and Myers , CR . 1992 . Microbial reduction of manganese and iron: New approaches to carbon cycling. Minireview . Appl. Environ. Microbiol , 58 : 439 – 443 .
- Neefs , J-M , Van de Peer , Y , Hendriks , L and De Wachter , R . 1990 . Compilation of small ribosomal subunit RNA sequences . Nucleic Acids Res , 18 ( suppl ) : 2237 – 2317 .
- Northup , DE , Barns , SM Yu , LE . 2003 . Diverse microbial communities inhabiting ferromanganese deposits in Lechuguilla and Spider Caves . Environ. Microbiol , 5 : 1071 – 1086 .
- Sass , H and Cypionka , H . 2004 . Isolation of sulfate-reducing bacteria from the terrestrial deep subsurface and description of Desulfovibrio cavernae sp. nov . System Appl. Microbiol , 27 : 541 – 548 .
- Stein , LY , La Duc , MT , Grundl , TJ and Nealson , KH . 2001 . Bacterial and archaeal populations associated with freshwater ferromanganous micronodules and sediments . Environ. Microbiol , 3 : 10 – 18 .
- Tebo , BM and Obraztsova , A Y . 1998 . Sulfate-reducing bacterium grows with Cr(VI), U(VI), Mn(IV), and Fe(III) as electron acceptors . FEMS Microbiol. Letters , 162 : 193 – 198 .
- Vandenabeele , J , Vandewoestyne , M , Houwen , F , Germonpré , R , Vandesande , D and Verstraete , W . 1995 . Role of autotrophic nitrifiers in biological manganese removal from groundwater containing manganese and ammonium . Microb. Ecol , 29 : 83 – 98 .
- Wada , H , Seirayosakol , A and Kimura , M . 1978a . The process of manganese deposition in paddy soils. I. A hyphothesis and its verification . Soil Sci. Plant Nutr , 24 : 55 – 62 .
- Wada , H , Seirayosakol , A , Kimura , M and Takai , Y . 1978b . The process of manganese deposition in paddy soils. II. The microorganisms responsible for manganese deposition . Soil Sci. Plant Nutr , 24 : 319 – 325 .
- Zehnder , AJB and Brock , TD . 1980 . Anaerobic methane oxidation: Occurrence and ecology . Appl. Environ. Mibrobiol , 39 : 194 – 204 .