Abstract
Methane-oxidizing bacteria (MOB) are crucial to the reduction of CH4 emitted to the atmosphere. However, it is unclear how MOB in rice straw are affected by straw decomposition processes. In a Japanese rice field, a year-round experiment was set up to study the effects of agricultural practice (rice cultivation/winter fallow), straw parts (leaf sheath/blade) and the site of straw placement (plow layer/soil surface) on MOB communities in rice straw using denaturing gradient gel electrophoresis (DGGE) and DNA sequencing analyses of key MOB functional genes (pmoA and amoA). Thirty-eight different DGGE bands were observed over the entire investigation period. Principal component analysis of DGGE pattern suggested that agricultural practice is the key factor regulating the MOB communities. Sequencing of dominant DGGE bands showed that: (1) during the rice cultivation period, methanotrophs (particularly type I methanotrophs) dominated the MOB community, (2) during the winter fallow season both type I and type II methanotrophs were dominant in sheath segments placed both on the soil surface and in the plow layer, whereas ammonia oxidizers seemed to dominate blade segments placed in the plow layer. Alignment of diagnostic amino acid sequences of MOB suggested the presence of novel ammonia oxidizers in rice straw in rice fields.
INTRODUCTION
Rice fields are important sources of atmospheric CH4, the most important non-CO2 greenhouse gas (CitationIntergovernmental Panel on Climate Change 2001). Rice straw incorporation in rice fields significantly increases CH4 emission (CitationKimura et al. 2004). Straw decomposition proceeds anaerobically during the rice cultivation period and aerobically during the winter fallow season in rice fields, and the microbial populations involved have been identified (CitationConrad and Klose 2006; CitationGlissmann et al. 2001; CitationKimura and Tun 1999; CitationSugano et al. 2005a,Citationb; CitationWeber et al. 2001). Under field conditions, the co-existence of oxic and anoxic habitats within rice straw might be highly possible during its year-round decomposition based on our previous molecular survey, particularly for straw placed on the soil surface (CitationAsari et al. 2007). Up to 60–90% of CH4 produced in rice fields was oxidized by methane-oxidizing bacteria (MOB) before it escaped to the atmosphere (CitationWassmann and Aulakh 2000). However, it is unclear how MOB communities change in rice straw along with its decomposition in rice fields.
MOB consist of methanotrophs and autotrophic ammonia oxidizers (CitationHanson and Hanson 1996). Virtually all methanotrophs use CH4 as a sole source of carbon and energy (CitationDedysh et al. 2005), whereas ammonia oxidizers use ammonia oxidation as an energy source and CH4 is co-oxidized at higher Km values without apparent benefit to the cells (CitationBedard and Knowles 1989). All known MOB possess membrane-bound methane monooxygenase (pMMO) or ammonia monooxygenase (AMO), the key enzymes for CH4 oxidation (CitationMurrell and Radajewski 2000), except for Methylocella (CitationDedysh et al. 2000). pMMO and AMO polypeptides showed greater than 40% identity, suggesting that these enzymes are evolutionarily related (CitationHolmes et al. 1995). Therefore, functional gene probes targeting key enzymes (pmoA of pMMO and amoA of AMO) have been developed and have advanced our knowledge of MOB ecology significantly in various ecosystems, such as forest soils (CitationBourne et al. 2001; CitationKnief et al. 2006; CitationKolb et al. 2005), lake sediments (CitationCostello and Lidstrom 1999), agricultural soils (CitationHenckel et al. 1999) and grassland soils (CitationHorz et al. 2005). Most of these studies detected lots of previously unknown pmoA sequences, including Cluster I, Cluster II and Upland Soil Cluster (CitationKnief et al. 2006), as well as unknown amoA sequences (CitationHorz et al. 2001). Although previously unrecognized pmoA are speculated to be atmospheric methane oxidizers (CitationKolb et al. 2005), based on culture-based evidence of the importance of ammonia oxidizers in CH4 oxidation (CitationHyman and Wood 1983; CitationJones and Morita 1983), CitationJiang and Bakken (1999) attempted to clarify whether or not ammonia oxidizers are responsible for atmospheric CH4 consumption. However, the role of ammonia oxidizers in biogeochemical CH4 cycle remains unclear, particularly in complex ecosystems (CitationHanson and Hanson 1996).
Seasonal changes in environmental conditions, such as water regime (CitationAsari et al. 2007) and temperature, in rice fields may influence the microorganisms inhabiting rice straw in soil. Based on phenotypic analyses of phospholipid fatty acids (CitationNakamura et al. 2003) and restriction fragment length polymorphism (RFLP) (CitationTun et al. 2002), agricultural practice (rice cultivation/winter fallow) and different sites of straw placement in rice fields (soil surface/plow layer) are assumed to be the key factors determining bacterial communities. In addition, CitationTun and Kimura (2000) elucidated microscopically that microorganisms conduct the decomposition of sheaths and blades in different ways. Furthermore, 16S rRNA analysis suggested that the straw part (sheath and blade) is crucial to the community structure of microorganisms (CitationSugano et al. 2005a,Citationb). However, it is unknown how these factors affect MOB in rice straw in soil. As rice straw is the main source of CH4 production in rice fields, it is interesting to study MOB inhabiting rice straw in soil from the viewpoint of global warming and soil microbial ecology.
Therefore, using denaturing gradient gel electrophoresis (DGGE) and DNA sequencing analysis of MOB functional genes, a year-round experiment was set up to investigate how MOB communities in rice straw are regulated by these environmental factors, including agricultural practice, different rice straw fractions and the site of straw placement.
MATERIALS AND METHODS
Site description
The experiment was carried out in a rice field at the Anjo Research and Extension Center, central Japan
Table 1 Field management during the rice cultivation period in the present study
(latitude 34°8′N, longitude 137°5′E). Soil was classified as Oxyaquic Dystrudept with 23% clay content. Selected soil properties were: total C 12.8 g kg−1, total N 1.1 g kg−1 and the pH(H2O) was 6.3. Field management is shown in , and very detailed information including meteorological conditions has been described elsewhere (CitationAsari et al. 2007; CitationNakamura et al. 2003; CitationSugano et al. 2005a,Citationb; CitationTun et al. 2002).
Rice straw incorporation and sampling
Rice straw (Oryza sativa L. cv. Koshihikari) was collected immediately after rice harvesting and air-dried. The treatment is outlined in during 1998 rice cultivation (1998-RC), 1998 winter fallow (1998-WF) and 1999 rice cultivation (1999-RC). In brief, leaf sheaths and blades were cut into 5 cm segments and five pieces of sheath segments (approximately 0.65 g dry weight) and 20 pieces of blade segments (approximately 0.45 g) were packed into nylon mesh bags with 1-mm pore size. Sheath segments were placed both on the soil surface and in the plow layer, while blade segments were applied only in the plow layer.
On 19 June and 12 November 1998, fresh sheath and/or blade segments were horizontally placed into the plow layer at a depth of approximately 10 cm or onto the soil surface as shown in . To simulate field conditions, leaf sheath samples on the soil surface that had been weathered during 1998-WF from 12 November 1998 to 14 April 1999 were taken out and placed again on the soil surface on 5 May 1999.
Fresh sheath/blade samples before placement (BP) were used as a control, and the weathered sheath on the soil surface on 14 April 1999 was regarded as the control for samples collected during 1999-RC. These straw samples were collected in three replicates, rinsed carefully with distilled water to remove soil particles, pooled and then kept at −20ºC until DNA extraction.
Figure 1 Schematic illustration of rice straw placement and sampling dates in a Japanese paddy field. Twelve blade and 12 sheath samples in the 10-cm plow layer were scheduled, as well as 15 sheath samplings on the soil surface. Standing water (5 cm) was above the soil surface during rice cultivation unless the field was drained.
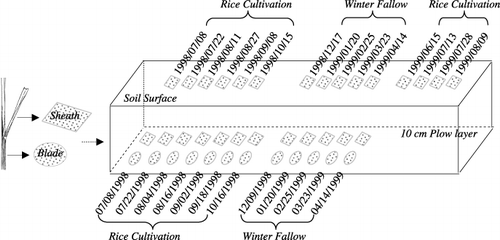
DNA extraction
A 0.5 g frozen rice straw sample was lyophilized overnight (lyophylisator FDU-540, Tokyo Rikakikai, Tokyo, Japan) and cut into 1–2 mm pieces for DNA extraction. Sodium dodecyl sulfate (SDS)-based DNA extraction, as previously described (CitationTun et al. 2002), was used with further cell lysis by freezing–thawing using liquid nitrogen. The DNA extract was purified using Sephadex G-200 silicon gel (CitationWatanabe et al. 2004) and dissolved in 100 µL TE (10 mM Tris — HCl, 1 mM EDTA; pH 8.0) buffer.
Polymerase chain reaction amplification
The functional gene pmoA/amoA primers designed by CitationHolmes et al. (1995) and slightly modified by CitationNold et al. (2000) were used in this study (forward primer: 5′-GGNGACTGGGAYTTCTGG-3′ and reverse primer: 5′-AAVGCVGAGAAGAAWGC-3′). A 40 bp GC clamp (CitationHenckel et al. 1999) was attached to the 5′ end of the forward primer to facilitate subsequent DGGE analysis. The first polymerase chain reaction (PCR) was carried out without a GC clamp. Individual reagents and their final concentrations or amounts were: 1× PCR buffer containing 2.0 mmol L−1 MgCl2 (TaKaRa Bio, Tokyo, Japan), 250 µmol L−1 of each deoxynucleoside triphosphate, 0.5 µmol L−1 of each primer, 400 ng µL−1 of bovin serum albumin, 2.5 units of Taq polymerase (TaKaRa Bio) and 5–20 ng of template DNA. A touchdown thermal program was used including: 94°C, 3 min; 15× (94°C, 30 s; 62°C to 55°C decreased in 0.5°C step, 30 s; 72°C, 45 s); 19× (94°C, 30 s; 55°C, 30 s; 72°C, 45 s); 1× (94ºC, 30 s; 55ºC, 30 s; 72ºC, 10 min). Amplification was started by placing pre-cooled PCR tubes immediately into the preheated thermal block of a PCR thermal cycler with a hot lid (TaKaRa Dice TP600, TaKaRa Bio). The expected PCR product was recovered using Ultrafree-DA kits (Millipore Corporation, Bedford, MA, USA) and used as template DNA for the 2nd PCR, which was the same as that described above except for the addition of a GC clamp to the forward primer. The thermal program consisted of 5 min at 94°C for denaturation, 20 cycles of 30s at 94°C, 30 s at 56°C and 60 s at 72°C with a 10 min final extension at 72°C for the last cycle. Aliquots (2 µL) of the PCR product were visualized by electrophoresis on 2% agarose gel.
Denaturing gradient gel electrophoresis analysis
The DGGE was carried out as described previously (CitationNiswati et al. 2004). Approximately 250 ng of PCR product was loaded and separated using a DCode Mutation Detection System (Bio-Rad Laboratory, Hercules, CA, USA) with 1-mm thick 8% (w/v) polyacrylamide gel (40% acrylamide-bisacrylamide [37.5:1]) with a denaturant gradient of 30–70% (100% denaturant corresponding to 7 mol L−1 urea, 40% formamide and 8% acrylamide). A parallel DGGE gel electrophoresis was run with 1 ¥ TAE buffer (40 mM Tris — HCl, 40 mM acetate, 1.0 mM EDTA) for 14 h at 60°C and 100 V. The gel was stained with 1:10,000 (v/v) SYBR Green I nucleic acid gel staining solution (Cambrex Bio Science, Rockland, ME, USA) for 40 min and photographed (Mamiya-OP, Saitama, Japan). A high reproducibility of DGGE fingerprinting data was obtained in this study, similar to our previous study (CitationJia et al. 2007).
Statistical characterization of DGGE band patterns of pmoA/amoA genes
The DGGE band patterns were normalized on the basis of fluorescent intensity (0, no visible band; 1, weak; 2, moderate; 3, strong; 4, very strong). Dominant DGGE bands were assumed to be the most important MOB, which reflected community changes in MOB in rice straw during its decomposition process over the investigated period. Characteristic DGGE bands (i.e. dominant MOB) with high Eigen values were identified by principal component analysis (PCA) of DGGE community patterns using EXCEL STATISTICS 1997 software (SRI, Tokyo, Japan).
Cloning, sequencing and phylogenetic analysis of pmoA/amoA functional genes
Characteristic DGGE bands were excised under illumination in a dark room. The excised bands were resuspended in 30 µL TE buffer, re-amplified, and then run again on DGGE gel for a mobility check with the parent DGGE bands from which the excised bands were cut. As a result of the appearance of multiple bands in the mobility check, the re-amplified PCR products were purified using Ultrafree-DA kits, ligated to pT7Blue T-vector (Novagen, Darmstadt, Germany) and transformed into competent cells of Escherichia coli XL I-blue (Toyobo, Osaka, Japan). For each sample, 15–30 clones were randomly selected and screened using colony-PCR in combination with a DGGE mobility check. Clones containing the correct insert were inoculated into LB (Luria Bertani broth) liquid medium and incubated overnight. Plasmid DNA was then extracted using a QIAprep spin miniprep kit (Qiagen, Crawley, UK) for sequencing.
DNA sequencing was carried out by an ABI 373S DNA sequencer (Applied Biosystems, Foster City, CA, USA) using a DYEnamic ET Terminator Cycle Sequencing kit (Amersham Biosciences, Sunnyvale, CA, USA) with vector-specific primers, according to the manufacturer's instructions.
DNA and amino-acid sequences were analyzed using GENETYX-Mac8.0 (GENETYX Corporation, Osaka, Japan). All sequences were also checked for their closest relatives using a BLAST search within GenBank. On an amino-acid level, 165 predicted amino-acid residues of PmoA/AmoA were aligned along with their closest relatives from GenBank using ClustalX 1.81 (CitationThompson et al. 1997). A neighbor-joining tree was made using Molecular Evolutionary Genetics Analysis software (MEGA 3.1) (CitationKumar et al. 2004), and the tree topology was independently checked using a Minimum Evolution algorithm.
The DNA sequences of partial pmoA/amoA genes have been deposited in GenBank under accession numbers AB222897 through to AB222932.
RESULTS
Denaturing gradient gel electrophoresis patterns of MOB communities colonizing rice straw in a rice field
Thirty-eight different DGGE bands in total were detected for all samples studied, but no band consistently appeared over the entire period (). The total number of different bands for sheath and blade samples that were placed in the plow layer and on the soil surface is shown in . Thirty-six different bands were observed for sheath samples placed both on the soil surface and in the plow layer across 1998-RC, 1998-WF and 1999-RC (). On the contrary, 29 different bands were found for blade and sheath samples placed in the plow layer (). As no PCR product was obtained from sheath samples collected on 23 March and 14 April 1999 during 1998-WF because of an unknown reason, there was no lane for those samples in . The band number of MOB inhabiting rice straw before incorporation ranged from four to seven, and the band number of MOB on rice straw after its incorporation varied from 1 to 15 (). It should be noted in , that all 11 MOB bands of weathered sheath on 14 April 1999 were thought to be MOB before straw incorporation in 1999-RC.
Statistical characterization of MOB communities in rice straw
As for MOB in sheaths and blades in the plow layer (), PCA analysis of DGGE patterns demonstrated that agricultural practice (rice cultivation/winter fallow) was the key factor regulating MOB communities (). Straw part (sheath or blade; ) and midseason drainage () were also found to affect the communities of MOB in rice straw.
Seventeen dominant MOB bands with high Eigen values were identified using PCA analysis of DGGE patterns across the entire investigated period and are indicated in . These bands accounted for 80% of the total MOB abundance in and 90% in, based on normalized band intensities. In addition, the abundance of newly appeared MOB after straw incorporation accounted for 78% and 76% of the total MOB abundance in and , respectively. However, within a certain straw decomposition period, MOB before and after straw incorporation might show very different dominance patterns. For example, the abundance of MOB before straw incorporation was up to 85% of the total MOB for blade samples in the plow layer during 1998-WF, and represented 76% of the total MOB abundance in 1999-RC.
Figure 2 Denaturing gradient gel electrophoresis (DGGE) fingerprinting patterns of the methane-oxidizing bacteria (MOB) colonizing rice straw applied to a paddy field. (a) Sheath samples in the plow layer and on the soil surface and (b) sheath and blade samples in the plow layer. BP-S1 and BP-S2 represent the fresh sheath control before and after rice cultivation, respectively. BP-B1 and BP-B2 were fresh blade control samples placed before and after rice cultivation, respectively. N refers to the pmoA/amoA-polymerase chain reaction negative control. Sheath on 14 April 1999 in bold was used as a control for the samples that followed. Values in the top and bottom lines above the DGGE pictures are the number of DGGE bands of MOB inhabiting the rice straw before and after its incorporation into the paddy field, respectively.
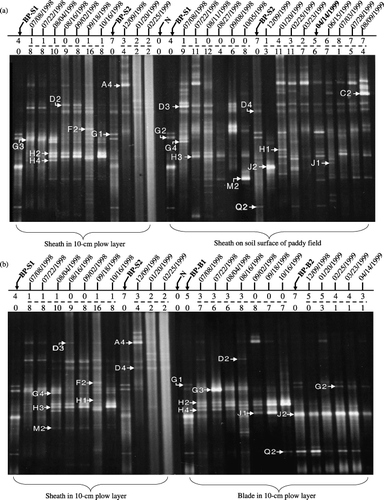
Figure 3 Principal component analysis of denaturing gradient gel electrophoresis (DGGE) fingerprinting patterns of methane-oxidizing bacteria (MOB) colonizing sheath and blade samples applied to the plow layer. (a) Score plots in the coordinate system of first and second principal components (PC) and (b) score plots in the coordinate system of first and third PC. The sample designation is S (sheath) or B (Blade) and the sampling date (as indicated in Fig. 1).
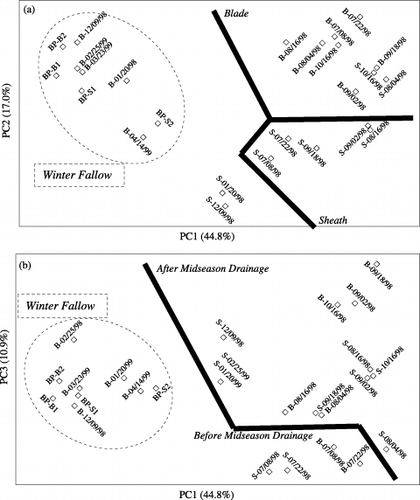
Phylogenetic characterization of MOB communities in rice straw
Phylogenetic analysis suggested that MOB communities in rice straw formed four distinct groups as shown in . As for type I methanotrophs, RS.H2, RS.H3 and RS.H4 were closely related to MOB retrieved from rice roots (CitationHorz et al. 2001), whereas RS.A4 and RS.G2 clustered with MOB from lake sediments (CitationNold et al. 2000). RS.D2, RS.D3 and RS.D4 of uncultured type II methanotrophs fell within cluster I, which was previously proposed as a new type II methanotrophic group (CitationKnief et al. 2006; CitationKolb et al. 2005; CitationRicke et al. 2005), and distantly related to cultured, but taxonomically uncharacterized, methanotroph K3-16 isolate (CitationPacheco-Oliver et al. 2002). RS.H1, RS.J1 and RS.J2 were found
Figure 4 Phylogenetic tree showing the relationship of PmoA/AmoA sequences retrieved from rice straw to public-domain PmoA/AmoA sequences. The tree was constructed using Molecular Evolutionary Genetics Analysis 3.1 software on the basis of a 165 amino-acid segment of the 27 kDa subunit of the PmoA and AmoA monooxygenases using a neighbor-joining algorithm. The scale bar represents the estimated number of substitutions per amino acid. Solid circles at internal nodes indicate bootstrap values >75% based on neighbor joining and a minimum evolution algorithm. Methane-oxidizing bacteria clones from rice straw, floodwater and rice bulk soils of paddy fields were prefixed with RS, FW and PS, respectively. AmoA-like β-proteobacteria refer to unculturedβ-proteobacteria containing AmoA-like peptides.
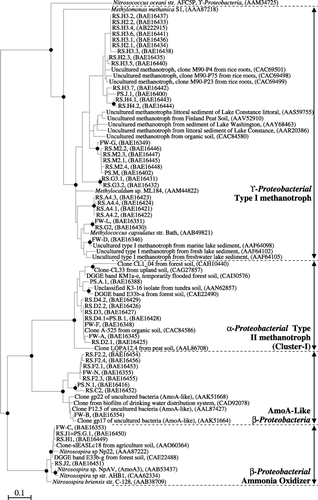
Table 2 Variation of conserved and signature amino acids of PmoA and AmoA of methane-oxidizing bacteria inhabiting rice straw incorporated into the paddy field
to be uncultured Nitrosospira spp., whereas RS.C2 and RS.F2 branched with uncultured AmoA-like bacteria.
An alignment analysis of diagnostic amino acids agreed well with the phylogenetic inference of MOB communities in rice straw. By manually aligning the amino acids of PmoA/AmoA of the MOB-type species indicated in , fifty-two universally conserved amino acids, 21 PmoA-typical and 21 AmoA-typical amino acids, were retrieved as previously reported (CitationHolmes et al. 1999). When compared with these signature residues, high variation was found for RS.C2, RS.F2, RS.D2, RS.D3 and RS.D4 (). RS.C2 and RS.F2 showed less than 60% amino-acid identity to cultured ammonia oxidizers.
DISCUSSION
Linking community changes in MOB in rice straw with environmental factors
Our results demonstrated that MOB communities were mainly regulated by agricultural practice. Regarding sheath and blade samples placed in the plow layer (), rice cultivation and winter fallow were found to be the key factors affecting MOB communities (). Meanwhile, a Shannon–Wiener diversity analysis showed that the diversity of MOB communities was dramatically lower during 1998-WF than the 1998/1999-RC periods (data not shown). Midseason drainage during 1998-RC led to two different clusters (). These results agreed well with our previous analyses of (Phospholipid fatty acid) PLFA (CitationNakamura et al. 2003) and RFLP (CitationTun et al. 2002), and suggested that water regime plays a key role in regulating MOB communities in rice straw because rice cultivation is basically characterized as a unique wetland with special water management (CitationKimura 2000). The PCA analysis () identified that different straw parts (sheath or blade) develop along with different MOB communities. Leaf blades harbored less diverse MOB communities than sheaths in the plow layer across 1998-RC and 1998-WF (Sorensen similarity analysis and Shannon–Wiener diversity analysis; data not shown). To the contrary, CitationSugano et al. (2005a,Citationb) found from 16S rDNA analysis of rice straw that plant parts (blade or sheath) were the primary factor regulating microbial communities inhabiting rice straw in rice fields rather than agricultural practice. This discrepancy may have arisen because MOB are strictly aerobic (CitationHanson and Hanson 1996) and previous results have mostly been obtained under anaerobic conditions.
It was noticeable that different sites of rice straw placement (plow layer/soil surface) showed no effect on MOB communities (data not shown), although rice straw has been shown to decompose more rapidly in soil than on the soil surface (CitationSain and Broadbent 1977). However, using normalized intensity analysis MOB bands originally detected from BP samples made up the major populations of MOB during 1998-WF. This implied that winter fallow strictly regulated MOB communities in sheaths on the soil surface. Interestingly, the newly appeared MOB after straw incorporation dominated MOB communities during 1998/1999-RC and also dominated sheaths and blades incorporated into the plow layer (). The MOB inhabiting rice straw before its incorporation through the 1998-WF weathering period composed 76.0% of the total MOB abundance of samples collected during 1999-RC (). These results might further corroborate the idea that agricultural practice was the leading factor regulating community changes in MOB in rice straw applied to rice fields (CitationAsari et al. 2007).
Phylogenetic characterization of MOB communities in rice straw
Sequencing analysis of dominant MOB bands formed four phylogenetically distinct clusters (). Uncultured type I methanotrophs of RS.A4, RS.G2, RS.H2, RS.H3, RS.H4, RS.G3 and RS.M2 were estimated to account for approximately 43% of the total MOB abundance in and approximately 55% in. Uncultured type II methanotrophic Cluster I constituted an important portion of MOB abundance across the entire investigated period. The abundance of uncultured AmoA-like bacteria (RS.C2 and RS.F2) plus uncultured ammonia oxidizers (RS.H1, RS.J1 and RS.J2) was less than 14% of the total MOB population. During 1998/1999-RC the dominant MOB were found to be closely related to type I methanotrophs retrieved from rice roots (CitationHorz et al. 2001) and lake sediments (CitationNold et al. 2000), regardless of sheath, blade or straw placement. Our previous study also showed that straight monounsaturated 16:1ω7c PLFA, the diagnostic PLFA of some type I methanotrophs (CitationRoslev and Iversen 1999), was abundant during 1998-RC (CitationNakamura et al. 2003). During 1998-WF, type I methanotrophs outplayed type II methanotrophs for the sheath samples on the soil surface. In contrast, type II methanotrophs were more abundant than type I methanotrophs for the sheath samples in the plow layer, which was also corroborated by methanotroph diagnostic PLFA analysis (CitationNakamura et al. 2003). For blade samples in the plow layer, ammonia oxidizers seemed to be predominant. In general, methanotrophs (particularly type I methanotrophs) characterized the rice cultivation period, whereas ammonia oxidizers played a relatively important role during the winter fallow season in this study.
The MOB retrieved using pmoA/amoA functional genes under field conditions have demonstrated a distinct phylogenetic profile compared with those from microcosm studies. For example, type I Methylococcus-related MOB and uncultured ammonia oxidizers were often observed under microcosm (CitationHenckel et al. 1999; CitationHoffmann et al. 2002; CitationHorz et al. 2001) and field conditions. However, MOB from microcosm experiments displayed a higher diversity, particularly MOB related to Methylocystis and Methylosinus of type II methanotrophs, which have never been detected under field conditions using pmoA/amoA gene markers. This trait was further confirmed by a microcosm molecular survey of MOB on a multi-nation sampling scale (CitationHoffmann et al. 2002). Uncultured type II methanotrophic Cluster I and uncultured AmoA-like bacteria were detected in rice fields for the first time in the present study. Cluster-I MOB were also retrieved from laboratory microcosms, whereas methanotrophs containing PmoA2 were only reported from rice roots in a flooded microcosm (CitationHorz et al. 2001). It is noteworthy that uncultured AmoA-like bacteria that have been retrieved only from rice roots (CitationHorz et al. 2001) were also observed in the present study, although amino-acid identity was lower than 65%. Care should be taken interpreting the absence of γ-proteobacterial ammonia oxidizers in the present study. First, the primer used in this study is actually designed based on sequences including γ-ammonia oxidizers (CitationHolmes et al. 1995). Second, γ-ammonia oxidizers have rarely been detected in soil environments. Therefore, we speculated that PCR bias against γ-ammonia oxidizers might have occurred in the present study, or the GC clamp might lower the affinity of pmoA/amoA primer to γ-ammonia oxidizers.
Diagnostic amino-acid analysis of MOB communities on rice straw
As shown in , an alignment analysis of diagnostic amino-acid residues of MOB led to a similar MOB classification to that inferred from the phylogenetic analysis (). Compared with 52 universally conserved residues out of 165 deduced amino acids of PmoA/AmoA polypeptide observed in known MOB, RS.C2 and RS.F2 of uncultured AmoA-like bacteria demonstrated high variation at presumably conserved positions, similar to α-proteobacterial methanotrophic Cluster I (). Cluster I was estimated to distantly belong to the taxonomically unclassified K3-16 isolate (CitationPacheco-Oliver et al. 2002). RS.C2 and RS.F2 of AmoA-like bacteria are likely to represent a novel ammonia oxidizer previously unknown with less than 60% of amino-acid identity to cultured ammonia oxidizers. A subset of seven amino-acid residues were supposed to be potential active-site metal ligands (CitationTukhvatullin et al. 2001), and the predicted residues of all dominant MOB shown in span three of these (H168: H residue at position 168; E100 and Y157), except for Cluster I and uncultured AmoA-like clusters in the present study. A further subset of four residues was proposed to be potential non-active site metal ligands, which could additionally stabilize the peptide structure (CitationTukhvatullin et al. 2001), and the amplified region in the present study spans two of these (D182 and Y196). All five of these residues identified falls within the universally conserved region.
In summary, it was found that MOB inhabiting rice straw that was applied to a rice field were mainly regulated by agricultural practice (rice cultivation/winter fallow). During the rice cultivation period, type I methanotrophs seemed to dominate MOB communities. In contrast, the predominant MOB communities are type I and type II methanotrophs during the winter fallow season for sheath segments placed not only in the plow layer but also on the soil surface, whereas ammonia oxidizers seemed to dominate MOB communities in blade segments in the plow layer. An alignment analysis of diagnostic amino-acid sequences of MOB on rice straw agreed well with the phylogenetic inference and suggested the presence of novel ammonia oxidizers.
ACKNOWLEDGMENTS
The authors are grateful to staff from the Anjo Research and Extension Center for field management and sampling assistance. Zhong-Jun Jia would like to extend his deep gratitude to Natsuko Nakayama and Takeshi Watanabe for invaluable help and to the Japan Society for the Promotion of Science for financial support.
REFERENCES
- Asari , N , Ishihara , R , Nakajima , Y , Kimura , M and Asakawa , S . 2007 . Succession and phylogenetic composition of eubacterial communities in rice straw during decomposition on the surface of paddy field soil . Soil Sci. Plant Nutr , 53 : 56 – 65 .
- Bedard , C and Knowles , R . 1989 . Physiology, biochemistry, & specific inhibitors of CH4, NH4+, & CO oxidation by methanotrophs and nitrifiers . Microbiol. Rev , 53 : 68 – 84 .
- Bourne , DG , McDonald , IR and Murrell , JC . 2001 . Comparison ofpmoA PCR primer sets as tools for investigating methanotroph diversity in three Danish soils . Appl. Environ. Microbiol , 67 : 3802 – 3809 .
- Conrad , R and Klose , M . 2006 . Dynamics of the methanogenic archaeal community in anoxic rice soil upon addition of straw . Eur. J. Soil. Sci , 57 : 476 – 484 .
- Costello , AM and Lidstrom , ME . 1999 . Molecular characterization of functional and phylogenetic genes from natural populations of methanotrophs in lake sediments . Appl. Environ. Microbiol , 65 : 5066 – 5074 .
- Dedysh , SN , Knief , C and Dunfield , PF . 2005 . Methylocella species are facultatively methanotrophic . J. Bacteriol , 187 : 4665 – 4670 .
- Dedysh , SN , Liesack , W Khmelenina , VN . 2000 . Methylocella palustris gen. nov., sp. nov., a new methane-oxidizing acidophilic bacterium from peat bogs, representing a novel subtype of serine-pathway methanotrophs . Int. J. Syst. Evol. Microbiol , 50 : 955 – 969 .
- Glissmann , K , Weber , S and Conrad , R . 2001 . Localization of processes involved in methanogenic degradation of rice straw in anoxic paddy soil . Environ. Microbiol , 3 : 502 – 511 .
- Hanson , R and Hanson , T . 1996 . Methanotrophic bacteria . Microbiol. Rev , 60 : 439 – 471 .
- Henckel , T , Friedrich , M and Conrad , R . 1999 . Molecular analyses of the methane-oxidizing microbial community in rice field soil by targeting the genes of the 16S rRNA, particulate methane monooxygenase, and methanol dehydrogenase . Appl. Environ. Microbiol , 65 : 1980 – 1990 .
- Hoffmann , T , Horz , HP , Kemnitz , D and Conrad , R . 2002 . Diversity of the particulate methane monooxygenase gene in methanotrophic samples from different rice fields in China and the Philippines . Syst. Appl. Microbiol , 2 : 267 – 274 .
- Holmes , AJ , Costello , A , Lidstrom , ME and Murrell , JC . 1995 . Evidence that particulate methane monooxygenase and ammonia monooxygenase may be evolutionarily related . FEMS Microbiol. Lett , 132 : 1225 – 1228 .
- Holmes , AJ , Roslev , P , McDonald , IR , Iversen , N , Henriksen , K and Murrell , JC . 1999 . Characterization of methanotrophic bacterial populations in soils showing atmospheric methane uptake . Appl. Environ. Microbiol , 65 : 3312 – 3318 .
- Horz , HP , Rich , V , Avrahami , S and Bohannan , BJM . 2005 . Methane-oxidizing bacteria in a California upland grassland soil: diversity and response to simulated global change . Appl. Environ. Microbiol , 71 : 2642 – 2652 .
- Horz , HP , Yimga , MT and Liesack , W . 2001 . Detection of methanotroph diversity on roots of submerged rice plants by molecular retrieval of pmoA, mmoX, mxaF, and 16S rRNA and ribosomal DNA, including pmoA-based terminal restriction fragment length polymorphism profiling . Appl. Environ. Microbiol , 67 : 4177 – 4185 .
- Hyman , MR and Wood , PM . 1983 . Methane oxidation by Nitrosomonas europaea . J. Biochem , 212 : 31 – 37 .
- Intergovernmental Panel on Climate Change . 2001 . “ Atmospheric chemistry and greenhouse gases ” . In Climate Change 2001: The Scientific Basis , Edited by: Houghton , JT , Ding , Y Griggs , DJ . 241 – 287 . Cambridge : Cambridge University Press .
- Jia , ZJ , Kikuchi , H , Watanabe , T , Asakawa , S and Kimura , M . 2007 . Molecular identification of methane oxidizing bacteria in a Japanese rice field soil . Biol. Fertil. Soils , DOI 10.1007/s00374-007-0186-x
- Jiang , QQ and Bakken , LR . 1999 . Nitrous oxide production and methane oxidation by different ammonia-oxidizing bacteria . Appl. Environ. Microbiol , 65 : 2679 – 2684 .
- Jones , RD and Morita , RY . 1983 . Methane oxidation by Nitrosococcus oceanus and Nitrosomonas europaea . Appl. Environ. Microbiol , 45 : 401 – 410 .
- Kimura , M . 2000 . “ Anaerobic microbiology in waterlogged rice fields ” . In Soil Biochemistry , Edited by: Bollag , JM and Stotzky , G . 35 – 138 . New York : Marcel Dekker .
- Kimura , M , Murase , J and Lu , YH . 2004 . Carbon cycling in rice field ecosystems in the context of input, decomposition and translocation of organic materials and the fates of their end products (CO2and CH4) . Soil Biol. Biochem , 36 : 1399 – 1416 .
- Kimura , M and Tun , CC . 1999 . Microscopic observation of the decomposition process of leaf sheath of rice straw and colonizing microorganisms during the cultivation period of paddy rice . Soil Sci. Plant Nutr , 45 : 427 – 437 .
- Knief , C , Kolb , S , Bodelier , PLE , Lipski , A and Dunfield , PF . 2006 . The active methanotrophic community in hydromorphic soils changes in response to changing methane concentration . Environ. Microbiol , 8 : 321 – 333 .
- Kolb , S , Knief , C , Dunfield , PF and Conrad , R . 2005 . Abundance and activity of uncultured methanotrophic bacteria involved in the consumption of atmospheric methane in two forest soils . Environ. Microbiol , 7 : 1150 – 1161 .
- Kumar , S , Tamura , K and Nei , M . 2004 . MEGA3: Integrated software for Molecular Evolutionary Genetics Analysis and sequence alignment . Brief. Bioinform , 5 : 150 – 163 .
- Murrell , JC and Radajewski , S . 2000 . Cultivation-independent techniques for studying methanotroph ecology . Res. Microbiol , 151 : 807 – 814 .
- Nakamura , A , Tun , CC , Asakawa , S and Kimura , M . 2003 . Microbial community responsible for the decomposition of rice straw in a paddy field: estimation by phospholipids fatty acid analysis . Biol. Fertil. Soils , 38 : 288 – 295 .
- Niswati , A , Murase , J , Asakawa , S and Kimura , M . 2004 . Analysis of communities of ammonia oxidizers, methanotrophs, and methanogens associated with microcrustaceans in the floodwater of a rice field microcosm . Soil Sci. Plant Nutr , 50 : 447 – 455 .
- Nold , SC , Zhou , J , Devol , AH and Tiedje , JM . 2000 . Pacific northwest marine sediments contain ammonia-oxidizing bacteria in the beta subdivision of the proteobacteria . Appl. Environ. Microbiol , 66 : 4532 – 4535 .
- Pacheco-Oliver , MC , McDonald , IR , Groleau , D , Murrell , JC and Miguez , CB . 2002 . Detection of methanotrophs with highly divergent pmoA genes from Arctic soils . FEMS Microbiol. Lett , 209 : 313 – 319 .
- Ricke , P , Kolb , S and Braker , G . 2005 . Application of a newly developed ARB software-integrated tool for in silico terminal restriction fragment length polymorphism analysis reveals the dominance of a novel pmoA cluster in a forest soil . Appl. Environ. Microbiol , 71 : 1671 – 1673 .
- Roslev , P and Iversen , N . 1999 . Radioactive fingerprinting of microorganisms that oxidize atmospheric methane in different soils . Appl. Environ. Microbiol , 65 : 4064 – 4070 .
- Sain , P and Broadbent , FE . 1977 . Decomposition of rice straw in soils as affected by some management factors . Environ. Qual , 6 : 96 – 100 .
- Sugano , A , Tsuchimoto , H , Tun , CC , Asakawa , S and Kimura , M . 2005a . Succession and phylogenetic profile of eubacterial communities in rice straw incorporated into a rice field: estimation by PCR-DGGE analysis . Soil Sci. Plant Nutr , 51 : 51 – 82 .
- Sugano , A , Tsuchimoto , H , Tun , CC , Kimura , M and Asakawa , S . 2005b . Succession of methanogenic archaea in rice straw incorporated into a Japanese rice field: estimation by PCR-DGGE and sequence analyses . Archaea , 1 : 391 – 397 .
- Thompson , JD , Gibson , TJ , Plewniak , F , Jeanmougin , F and Higgins , DG . 1997 . The ClustalX windows interface: flexible strategies for multiple sequence alignment aided by quality analysis tools . Nucl. Acids. Res , 24 : 4876 – 4882 .
- Tukhvatullin , IA , Gvozdev , RI and Anderson , KK . 2001 . Structural and functional model of methane hydroxylase of membrane-bound methane monooxygenase from Methylococcus capsulatus (Bath) . Russian Chem. Bull , 50 : 1867 – 1876 .
- Tun , CC , Ikenaga , M , Asakawa , S and Kimura , M . 2002 . Community structure of bacteria and fungi responsible for rice straw decomposition in a paddy field estimated by PCR-RFLP analysis . Soil Sci. Plant Nutr , 48 : 805 – 813 .
- Tun , CC and Kimura , M . 2000 . Microscopic observation of the decomposition process of leaf blade of rice straw and colonizing microorganisms in a Japanese paddy field soil during the cultivation period of paddy rice . Soil Sci. Plant Nutr , 46 : 127 – 137 .
- Wassmann , R and Aulakh , MS . 2000 . The role of rice plants in regulating mechanisms of methane missions . Biol. Fertil. Soils , 31 : 20 – 29 .
- Watanabe , T , Asakawa , S , Nakamura , A , Nagaoka , K and Kimura , M . 2004 . DGGE method for analyzing 16S rDNA of methanogenic archaeal community in paddy field soil . FEMS Microbiol. Lett , 232 : 153 – 163 .
- Weber , S , Stubner , S and Conrad , R . 2001 . Bacterial populations colonizing and degrading rice straw in anoxic paddy soil . Appl. Environ. Microbiol , 67 : 1318 – 1327 .