Abstract
To clarify the microbiological factors that explain high N2O emission in an arable peat soil in Central Kalimantan, Indonesia, a substrate-induced respiration-inhibition experiment was conducted for N2O production. The N2O emission rate decreased by 31% with the addition of streptomycin, whereas it decreased by 81% with the addition of cycloheximide, compared with a non-antibiotic-added control. This result revealed a greater contribution of the fungal community than bacterial community to the production of N2O in the soil. The population density of fungi in the soil, determined using the dilution plate method, was 5.5 log c.f.u. g−1 soil and 4.9 log c.f.u. g−1 soil in the non-selective medium (rose bengal) and the selective medium for Fusarium, respectively. The N2O-producing potential was randomly examined in each of these isolates by inoculation onto Czapek agar medium (pH 4.3) and incubation at 28°C for 14 days. Significant N2O-producing potential was found in six out of 19 strains and in five out of seven strains isolated from the non-selective and selective media, respectively. Twenty-three out of 26 strains produced more than 20% CO2 during the 14-day incubation period, suggesting the presence of facultative fungi in the soil. These strains were identified to be Fusarium oxysporum and Neocosmospora vasinfecta based on the sequence of 18S rDNA, irrespective of the N2O-producing potential and the growth potential in conditions of low O2 concentration.
INTRODUCTION
N2O is an important greenhouse gas produced in soil (CitationBouwman 1990). It has a destructive potential in the stratospheric ozone layer (CitationCrutzen 1981). N2O is produced as a byproduct of nitrification and as an intermediate product of denitrification, in which produced NO- 3 is respired in conditions of low O2 availability as an alternative final electron acceptor.
In an arable land in Central Kalimantan, Indonesia, the highest N2O emission is recorded among the values reported from cultivated tropical, temperate and boreal organic soils (CitationTakakai et al. 2006). To clarify the reason for such high N2O emission, isolation of the denitrifying bacteria has been carried out as a source of N2O production (Hashidoko et al.). Although N2O-producing microorganisms have long been regarded to be bacteria (CitationZumft 1997), recent focus has been on the contribution of fungi. CitationShoun and Tanimoto (1991) found potent denitrifying activity in the hyphomycetous fungus Fusarium oxysporum, and CitationShoun et al. (1992) reported that a range of fungi (Fusarium and its teleomorphs) had the relevant enzyme P-450nor and N2O-producing activity in conditions of low O2 concentration. This result was supported by genome projects (CitationZhang et al. 2001) and a laboratory experiment that revealed the requirement of a minimal amount of O2 for the induction of the denitrifying enzyme of fungi (CitationZhou et al. 2001). These results suggest active N2O production by a variety of fungi in upland conditions of soil rather than in flooded conditions, which have previously been believed to be sufficient conditions for denitrification.
Recently, a high contribution of fungi to N2O production in arable soil was demonstrated. CitationLaughlin and Stevens (2002) demonstrated the dominance of fungal N2O production in a grassland soil, which was evaluated using the substrate-induced respiration-inhibition (SIRIN) method, which was originally developed for the estimation of soil microbial biomass (CitationAnderson and Domsch 1975). Streptomycin (C21H39N7O12) was used as the bacterial inhibitor and cycloheximide (C15H23NO4) was used as the fungal inhibitor, and cycloheximide decreased the N2O emission rate by 89%, whereas streptomycin decreased the rate by 23% (CitationLaughlin and Stevens 2002), indicating a greater contribution of fungal N2O production in soil. CitationSpokas et al. (2006) showed stimulation of N2O emissions from soil in a grassland after the application of chloropicrin, and the addition of a bacterial inhibitor (streptomycin and tetracycline: C22H24N2O8) suppressed N2O production by 20%, whereas the addition of a fungal inhibitor (cycloheximide and benomyl: C14H18N4O3) suppressed it by 70%, indicating a greater contribution of fungal N2O production in soil. However, the presence of denitrifying fungi was not confirmed in these studies.
In the present study, the N2O-producing microbial community was characterized by an inhibition experiment, and the relevant microorganisms were isolated from an air-dried soil sample collected from peatland in Central Kalimantan, Indonesia.
MATERIALS AND METHODS
Soil samples
Surface (0–10 cm depth) soil samples were collected from two croplands (referred to as CL-A and CL-C) and adjacent natural forest (referred to as NF) at Kalampangan village, near Palangka Raya (2°S, 114°E) in Central Kalimantan, Indonesia, in 2003 and 2005. Physicochemical properties of the tropical peat soils and general site information are shown in CitationTakakai et al. (2006). Fresh and large plant debris was removed and the soil samples were stored at room temperature until use.
Nitrification and denitrification potential
Nitrification potential was determined by NO- 3 accumulation in the peat soil during 10 days of incubation at 28°C after the addition of 200 µg N g−1soil of (NH4)2SO4, and expressed as the net NO- 3 production rate. Air-dried peat soil from CL-A, CL-C and NF (equivalent to 1 g of dried soil, gravimetric water content was 0.82, 0.61 and 1.81 g g−1 dry soil, respectively) was placed in 50 mL of plastic tube and a substrate solution was added to adjust the water content to 4 g g−1 dry soil. The NO- 3-N content in the soil was determined as previously described (CitationYanai et al. 2004). The denitrification potential was determined using the method described in CitationYanai et al. (in press), with a slight modification in the concentration of KNO3 in 8 g nutrient broth per liter from 5 mmol L−1 to 50 mmol L−1, because of originally high NO3-N content in the soil at CL-A (236 µg N g−1 dry soil; CitationTakakai et al. 2006). The air-dried peat soil (equivalent to 1 g of dried soil) was placed in a 30 mL grass vial and 5 mL of the liquid medium was added. After sealing with a butyl rubber septum in the ambient condition, 100% C2H2 was added to make the headspace concentration of C2H2 10% to suppress the N2O-reducing activity of denitrifiers (CitationYoshinari et al. 1977; referred to as +C2H2) and the total pressure in the vial was set to the atmospheric pressure. To estimate N2O-reducing activity, vials without C2H2 addition were also prepared, and the samples were incubated at 30°C. N2O concentration in the headspace was periodically measured using a gas chromatograph (GC-8A; Shimadzu, Kyoto, Japan) equipped with a thermal conductivity detector (TCD). The running condition is described in CitationWada and Toyota (2007).
Substrate-induced respiration-inhibition experiment
Air-dried peat soil from CL-A (20 g of air-dried material, gravimetric water content was 0.21 g g−1 dry soil) was placed into nine Petri dishes (1.3 cm height and 8.5 cm diameter) without compaction. A glucose solution (final concentration: 5 mg glucose g−1 dry soil), including each antibiotic (streptomycin or cycloheximide), was added into the soil to adjust its water content to 1.1 g g−1 dry soil (58% of water-filled pore space [WFPS]). The concentrations of the antibiotics were 3 mg streptomycin g−1 dry soil and 15 mg cycloheximide g−1 dry soil (CitationLaughlin and Stevens 2002). In this condition, CO2 production was significantly decreased (23 and 26%) by the addition of streptomycin and cycloheximide, respectively (P < 0.01). After the addition of the substrate solution, peat soils were incubated for 6 h in a laboratory (18–23°C) and the N2O emission rate was determined using the closed chamber method as shown in CitationYanai et al. (2007).
Enumeration and isolation of filamentous fungi in soils
Based on the result of the SIRIN experiment, colony-forming units of culturable fungi were estimated using the dilution plate method with rose bengal medium as a non-selective cultivation and GMBP medium (CitationTakehara et al. 2003) as a selective cultivation for Fusarium. After inoculation of the soil dilution, the media were incubated at 28°C for 4 days. Part of the colonies was randomly collected, purified and a freeze stock (in 20% glycerol solution) was prepared for further analysis.
Determination of the N2O-producing potential of isolated fungi
Modified Czapek medium (30 g sucrose, 0.5 g MgSO4 7H2O, 1 g KH2PO4, 0.5 g KCl, 2 g NaNO3, 0.01 g Fe2(SO4)3 in 1 L of distilled water, pH 4.3) with 50 µg mL−1 streptomycin was solidified with 2% (w/v) agar and was used to test the N2O-producing potential of the fungal isolate. The isolate was aseptically inoculated in the Czapek agar medium in a 100 mL Erlenmeyer flask sealed with a rubber stopper in the ambient condition, and incubated at 28°C. After 14 days of incubation, 0.5 mL of the headspace gas was withdrawn from the headspace of the flask through a rubber stopper and the CO2 and N2O concentrations were analyzed using the TCD gas chromatograph. When the N2O concentration was less than 200 p.p.m.v, an additional 5 mL of headspace gas was withdrawn and the N2O concentration was analyzed using a gas chromatograph (GC-14A: Shimadzu, Kyoto, Japan) equipped with a 63Ni-electron capture detector (ECD-GC). The running condition of the ECD-GC is shown in CitationYanai et al. (2007). In the present study, Fusarium oxysporum (Fusarium oxysporum f. sp. lactucae) was examined as a positive control (denitrifying fungi that can grow under conditions of low O2 concentration and produce N2O) and Pythium (Pythium ultimum Trow var ultimum OPU407) and Rhizoctonia sp. were examined as negative controls (fungal strains that can grow only under oxic conditions and do not have N2O-producing activity). After determination of the headspace gas, BTB (C27H28O5Br2S) solution was added to estimate the pH of the agar medium.
Fungal DNA extraction and analysis based on 18S rDNA
Pure cultures of filamentous fungi were grown in the modified Czapek medium for 3–10 days at 30°C prior to DNA extraction. Fungal mycelia were collected from liquid culture and 400–800 µL (bulk volume) was added to 1.5 mL tubes. Mycelium material was washed twice with TE buffer and transferred to 2 mL tubes containing 0.75 g of 0.1-mm diameter zirconium beads and 0.25 g of 0.5-mm diameter glass beads. The mycelium material was suspended in 1,200 µL of a lysis buffer containing 0.1 mol L−1 Tris-HCl (pH 8.0), 0.3 mol L−1 ethylenediaminetetraacetic acid (pH 8.0) and 0.5% sodium dodecyl sulfate, and then bead beating was carried out using a Bead Smash 12 (BS-12; Wakenyaku, Kyoto, Japan) twice for 1 min at 5,000 rev min−1. Then, the tubes were centrifuged for 5 min at 12,000 g and 700 µL of the supernatant was transferred into new 2 mL tubes. Each supernatant was suspended in 377 µL of 5 mol L−1 NaCl and 270 µL of 10% CTAB (cetyltrimethylammonium bromide), and incubated at 60°C for 10 min. After cooling at room temperature, 500 µL of chloroform was added to each sample, mixed vigorously using a vortex for 15 s and then centrifuged for 20 min at 15,000 g. Then, 400 µL of the aqueous layer was transferred to a new 1.5 mL tube, 1 µL of 10 mg mL−1 RNase was added and incubated at 37°C for 30 min. After the incubation, 40 µL of 3 mol L−1 CH3COONa and 400 µL of isopropanol were added to each sample for the precipitation of DNA. The tubes were placed at room temperature for 30 min and centrifuged at 4°C for 5 min at 20,630 g. All supernatant was removed and centrifuged again at 4°C for 5 min at 20,630 g after the addition of 500 µL of 70% ethanol to wash the remaining pellet. The pellets were air-dried and re-suspended in 20–50 µL of sterile TE buffer. The subsequent DNA yields and quality were assessed using standard electrophoresis through a 1% (w/v) agarose gel followed by ethidium bromide staining.
The forward primer NS1-seq (TGG AAA ACG GCC AGT GTA GTC ATA TGC TTG TCT C) and the reverse primer FR1-GC (CCC CCG CCG CGC GCG GCG GGC GGG GCG GGG GCA CGG GCC GAI CCA TTC AAT CGG TAI T) were used for polymerase chain reaction (PCR) amplification of the 18S rDNA gene (CitationVainio and Hautula 2000). The reaction was carried out in a 25 µL volume that contained approximately 15 ng DNA, 10 mmol L−1 Mg-free buffer, 0.2 mmol L−1 deoxynucleoside triphosphates, 3.75 mmol L−1 MgCl2, 0.4 µg µL−1 BSA (Bovine Serum Albumin), 0.16 µmol L−1 of each primer and 2 U µL_1 of Taq DNA polymerase (TaKaRa Ex Taq; TaKaRa Bio, Shiga, Japan). The samples were subjected to 30 cycles of 94°C for 30 s, 48°C for 45 s and 72°C for 180 s for denaturation, annealing and elongation steps, respectively, using a thermal cycler (Dice mini; TaKaRa Bio). An initial denaturation step (94°C for 5 min) was used to ensure complete denaturation of the template DNA. The PCR products were first analyzed using electrophoresis in 0.75% (w/v) agarose gels and ethidium bromide staining. Direct sequencing of the PCR products derived from various isolates was done by Hitachi Science Systems (Tokyo, Japan). The sequences obtained have been submitted to the DDBJ (DNA Data Bank of Japan) under accession numbers AB302197 to AB302214, and were examined using the DDBJ homology search system BLAST (http://blast.ddbj.nig.ac.jp/top-j.html).
RESULTS AND DISCUSSION
The CL-A and CL-C peat soils showed higher activity of nitrification and denitrification compared with the NF peat soil (,). These results are comparable with the amount of N2O emission from these fields (CitationTakakai et al. 2006), suggesting that biochemical N
Figure 1 Nitrification potential of the peat soils collected from arable land (CL-A, CL-C) and adjacent natural forest (NF). Values shown are mean ± standard deviation (n = 2).
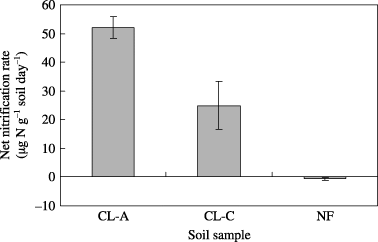
Figure 2 Denitrification potential of the peat soils collected from arable land (CL-A, CL-C) and adjacent natural forest (NF). Values shown are mean ± standard deviation (n = 2). After a 70-h incubation period, N2O production of the CL-A soil sample incubated with and without C2H2 was significantly different according to an unpaired t-test (P < 0.05). +, the incubation of soil with 10% C2H2 in the headspace; –, the incubation of soil without 10% C2H2 in the headspace.
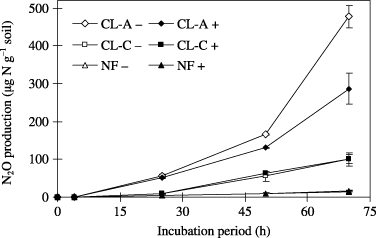
Figure 3 Effect of the addition of antibiotics on N2O production in the peat soil (CL-A). Values shown are mean ± standard deviation (n = 3). NS, not significant (P = 0.121); **P < 0.01 (Dunnett's test results).
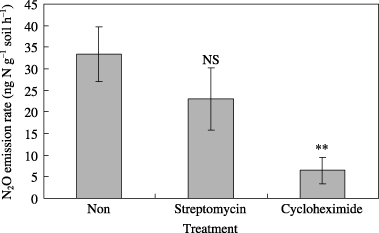
The N2O emission rates from the CL-A peat soil were 33 ± 6.3, 23 ± 7.2 and 6.5 ± 3.1 ng N2O-N g−1 dry soil per hour in the non-antibiotic control, the streptomycin addition and the cycloheximide addition, respectively (mean ± standard deviation [SD], n = 3; ). The cycloheximide addition decreased the N2O emission rate by 81% (P < 0.01), whereas streptomycin decreased the rate by 31% (P = 0.12), although the bacterial and fungal biomass ratio was approximately 1:1. As a high N2O emission rate (approximately 8,000 µg N m−2 h−1) was recorded at the CL-A site at 60% WFPS (CitationTakakai et al. 2006), and the moisture condition in the SIRIN experiment was similar (58% WFPS), this result suggests that fungal N2O production may have a considerably high contribution at the CL-A site. In this sense, the unusual pattern of N2O production of soil incubated with or without C2H2 () can be interpreted as fungistasis caused by trace C2H4 in the C2H2 (CitationLiebman and Epstein 1994), although we did not test this experimentally.
The population density of culturable fungi in the CL-A peat soil was 5.5 log c.f.u. g−1 dry soil (n = 2) and 4.9 log c.f.u. g−1 dry soil (n = 3) in non-selective and selective media, respectively, and six out of 19 strains from the non-selective medium and five out of seven strains from the selective medium had N2O-producing potential (). Irrespective of the N2O-producing potential, some strains showed unexpectedly high CO2 production, that is, the CO2 concentration in the headspace exceeded 20% during the 14-day incubation period, suggesting significant growth even after the consumption of O2 in the ambient air (). The nearest species of these strains were identified as Fusarium oxysporum and Neocosmospora vasinfecta (). In general, eukaryotic organisms, including fungus, only use O2 as a final electron acceptor. In fact, the Pythium did not produce N2O and the CO2 concentration did not exceed 20% even after a 14-day incubation (data not shown). In contrast, the Rhizoctonia did not produce N2O, but the CO2 concentration in the
Table 1 Isolation of fungi from peat soil in a crop land (CL-A) and their potential denitrification activity (14-day incubation period)
In addition, despite high CO2 accumulation in the flask and the use of acidified agar medium (pH 4.3), eight out of 31 cultures showed neutral or alkali pH after the 14-day incubation period (), suggesting ammonia fermentation by fungi (CitationZhou et al. 2002). Denitrification by fungi is an ATP-producing reaction in mitochondria at low O2 conditions that results in the production of N2O, whereas ammonia fermentation by fungi is an ATP-producing reaction at cytosol at low O2 conditions that does not result in the production of N2O. Therefore, this study experimentally suggested that a variety of fungi in soil can grow under conditions of low O2 concentration and a some of these fungi are involved in N2O production in the soil.
CitationTakakai et al. (2006) suggested that changing land use from forestry to agriculture increases N2O production in the soil. Although there is no experimental evidence for this suggestion, such changes in land use can affect microbial community structure (enrichment of N2O-producing microorganisms), resulting in the stimulation of N2O production in the soil. Further study is expected to clarify the contribution of fungal communities to N2O emissions from peat soil, the diversity of the fungal community that produces N2O in the soil, and the relationship between N2O emission from soil and soil-borne plant disease because the fungi obtained in this study are Fusarium oxysporum and Neocosmospora vasinfecta, which are known to be plant-pathogenic fungi (CitationO'Donnell and Gray 1995).
ACKNOWLEDGMENTS
We thank Mr S. Yoshida (Nagano Chusin Agricultural Experiment Station) for providing a fungal strain of Fusarium oxysporumf. sp. lactucaeand Dr M. Tojo (Osaka Prefecture University) for providing a fungal strain of Pythium ultimum Trowvar ultimumOPU407. We also thank Mr M. Amari, Ms E. Sato, Mr T. Shirakashi and Ms A. Enomoto for their technical assistance. We are grateful to Professor M. Okazaki for fruitful discussions. This study was financially supported by the Japan Society for the Promotion of Science (JSPS) Research Fellowships for Young Scientists (17-6518), a Japanese Grant-in-Aid for Science Research from the Ministry of Education, Culture, Sports, Science and Technology (13574012), and the JSPS-LIPI (Indonesian Institute of Sciences) Core University Program.
REFERENCES
- Anderson , JPE and Domsch , KH . 1975 . Measurement of bacterial and fungal contributions to respiration of selected agricultural and forest soils . Can. J. Microbiol , 21 : 314 – 322 .
- Bouwman , AF . 1990 . “ Exchange of greenhouse gases between terrestrial ecosystems and the atmosphere ” . In Soils and the Greenhouse Effect , Edited by: Bouwman , AF . 61 – 127 . New York : John Wiley .
- Crutzen , PJ . 1981 . “ Atmospheric chemical processes of the oxides of nitrogen, including nitrous oxide ” . In Denitrification, Nitrification and Atmospheric Nitrous Oxide , Edited by: Delwiche , CC . 17 – 44 . New York : John Wiley .
- Hashidoko , Y , Takakai , F Toma , Y . in press . Emergence and behaviors of acid-tolerant Janthinobacterium sp. that evolves N2O from deforested tropical peatlandSoil . Biol. Biochem , doi: 10.1016/j.soilbio.2007.07.014
- Laughlin , RJ and Stevens , RJ . 2002 . Evidence for fungal dominance of denitrification and codenitrification in a grassland soil . Soil Sci. Soc. Am. J , 66 : 1540 – 1548 .
- Liebman , JA and Epstein , L . 1994 . Partial characterization of volatile fungistatic compound(s) from soil . Phytopathology , 84 : 442 – 446 .
- Lockhart , RJ , Van Dyke , MI , Beadle , IR , Humphreys , P and McCarthy , AJ . 2006 . Molecular biological detection of anaerobic gut fungi (Neocallimastigales) from landfill sites . Appl. Environ. Microbiol , 72 : 5659 – 5661 .
- O'Donnell , K and Gray , LE . 1995 . Phylogenetic relationships of the soybean sudden-death syndrome pathogen Fusarium solani f. sp. phaseoli inferred from rDNA sequence data and PCR primers for its identification . Mol. Plant Microbe Int , 8 : 709 – 716 .
- Shoun , H , Kim , D , Uchiyama , H and Sugiyama , J . 1992 . Denitrification by fungi . FEMS Microbiol. Lett , 94 : 277 – 282 .
- Shoun , H and Tanimoto , T . 1991 . Denitrification by the fungus Fusarium oxysporum and involvement of cytochrome-P-450 in the respiratory nitrite reduction . J. Biol. Chem , 266 : 11078 – 11082 .
- Spokas , K , Wang , D , Venterea , R and Sadowsky , A . 2006 . Mechanisms of N2O production following chloropicrin fumigation . Appl. Soil Ecol , 31 : 101 – 109 .
- Takakai , F , Morishita , T Hashidoko , Y . 2006 . Effects of agricultural land-use change and forest fire on N2O emission from tropical peatlands, Central Kalimantan, Indonesia . Soil Sci. Plant Nutr , 52 : 662 – 674 .
- Takehara , T , Kuniyasu , K , Mori , M and Hagiwara , H . 2003 . Use of a nitrate-nonutilizing mutant and selective media to examine population dynamics of Fusarium oxysporum f. sp. spinaciae in soil . Phytopathol , 93 : 1173 – 1181 .
- Tiedje , JM . 1994 . “ Denitrifiers Methods of Soil Analysis ” . In Part 2-Microbiological and Biochemical Properties , Edited by: Weaver , RD , Angle , JS and Bottomley , PS . 245 – 267 . Wisconsin : Soil Science Society of America .
- Vainio , EJI and Hantula , J . 2000 . Direct analysis of wood-inhabiting fungi using denaturing gradient gel electrophoresis of amplified ribosomal DNA . Mycol. Res. , 104 : 927 – 936 .
- Wada , S and Toyota , K . 2007 . Repeated applications of farmyard manure enhance resistance and resilience of soil biological functions against soil disinfection . Biol. Fertil. Soils , 43 : 349 – 356 .
- Yanai , Y , Toyota , K and Okazaki , M . 2007 . Effects of charcoal addition on N2O emissions from soil resulting from rewetting air-dried soil in short-term laboratory experiments . Soil Sci. Plant Nutr , 53 : 181 – 188 .
- Yanai , Y , Toyota , K and Okazaki , M . in press . Response of denitrifying communities to successive soil freeze–thaw cycles . Biol. Fertil. Soils , doi: 10.1007/s00374-007-0185-y
- Yanai , Y , Toyota , K and Okazaki , M . 2004 . Effects of successive freeze thaw cycles on nitrification potential of soils . Soil Sci. Plant Nutr , 50 : 831 – 837 .
- Yoshinari , T , Hynes , R and Knowles , R . 1977 . Acetylene inhibition of nitrous oxide reduction and measurement of denitrification and nitrogen fixation in soil . Soil Biol. Biochem , 9 : 177 – 183 .
- Zhang , L , Takaya , N , Kitazume , T , Kondo , T and Shoun , H . 2001 . Purification and cDNA cloning of nitric oxide reductase cytochrome P450nor (CYP55A4) from Trichosporon cutaneum . Eur. J. Biochem , 268 : 3198 – 3204 .
- Zhou , ZM , Takaya , N , Nakamura , A , Yamaguchi , M , Takeo , K and Shoun , H . 2002 . Ammonia fermentation, a novel anoxic metabolism of nitrate by fungi . J. Biol. Chem , 277 : 1892 – 1896 .
- Zhou , ZM , Tanaka , N , Sakairi , MAC and Shoun , H . 2001 . Oxygen requirement for denitrification by the fungus Fusarium oxysoirum . Arch. Microbiol , 175 : 19 – 25 .
- Zumft , WG . 1997 . Cell biology and molecular basis of denitrification . Microbiol. Mol. Biol. Rev , 61 : 533 – 616 .