Abstract
Acid phosphatase (APase) produced by the cluster roots of white lupin (Lupinus albus L.) plays an important role in inorganic phosphate (Pi) acquisition. Although the importance of cluster roots in Pi acquisition is well known, information on the distribution of APase within tissues of normal and cluster roots is lacking. Isoelectric focusing of APase isoforms as well as histochemical localization and visualization of APase were used to clarify the importance of secretory APase for P nutrition of white lupin grown under P deficiency. Isoelectric focusing revealed that both the secretory type and other major APase isoforms probably involved in P translocation were inducible. The major activity in the rhizosphere soil of cluster roots and roots grown under hydroponic conditions corresponded to LASAP2, a previously purified APase secreted from white lupin roots. Histochemical localization using enzyme-labeled fluorescence (ELF)-97 phosphate as a substrate was applied to rhizosphere samples. This substrate provides fluorescent precipitates after hydrolysis by phosphatase. Strong APase activity in the epidermal tissues of normal roots and cluster rootlets and in root hairs of cluster rootlets under P deficiency was detected. These results support the hypothesis that APase activities in the rhizosphere liberate Pi and supply it to white lupin plants grown under P-deficient conditions.
INTRODUCTION
The white lupin plant has developed several mechanisms to obtain phosphorus from the soil. Abundant exudates, such as acid phosphatase (APase), and organic acids from the roots cause inorganic phosphate (Pi) to be released from organic and sparingly soluble P compounds (CitationDinkelaker et al. 1989; CitationGardner et al. 1983; CitationTadano and Sakai 1991). White lupin forms unique root clusters resembling bottle brushes, designated as “cluster roots” (or proteoid roots), under P and Fe deficiency (CitationNeumann and Martinoia 2002). Cluster roots yield P not only by increasing the root surface area, but also by the upregulation of Pi transporters and APases (CitationLiu et al. 2001; CitationMiller et al. 2001; CitationWasaki et al. 2003).
CitationOzawa et al. (1995) used isoelectric focusing to demonstrate that secreted APase was one of the APase isoforms in the roots. They also purified and characterized the secreted APase protein: it is a homodimer with an estimated molecular weight of 140 kDa, 72 kDa for each subunit, it has wide substrate specificity, and is stable at pH 4.0–9.0. Like many other secreted proteins, the APase is glycosylated, which protects it against proteolytic enzymes and contributes to its stability over a wide pH range (CitationOzawa et al. 1995). The half-life of the enzyme in soil solution at 25°C was estimated to be 2 weeks, suggesting high stability (CitationTadano et al. 1993). These properties are consistent with the role suggested for APase in the hydrolysis of organic P in soil.
The APase secreted from lupin roots is important for P acquisition from organic P sources in soils. Injecting crude APase collected from lupin exudates into the rhizosphere of tomato and sugar beet plants increased plant growth and P absorption (CitationTadano and Komatsu 1994). This result suggests that APase contributes to phosphorus acquisition from organic phosphate compounds. LASAP2 cDNA for the secreted APase has been isolated from phosphorus-deficient white lupin (CitationWasaki et al. 2000). The mRNA was not constitutive, but inducible in phosphorus-deficient roots, especially in cluster roots (CitationWasaki et al. 2000, Citation2003). The age of the root cluster and the cultivation methods influenced the activity of the secreted APase. Mature and senescent cluster roots showed the highest APase activity in hydroponic and soil cultures, respectively (CitationNeumann et al. 2000; CitationWasaki et al. 2005).
Although the importance of cluster roots for the local release of Pi is well known, no information is available on the small-scale distribution of APase within roots and cluster roots. APase was localized histochemically using a new type of fluorogenic compound, enzyme-labeled fluorescence (ELF)-97 phosphate (CitationNedoma and Vrva 2006; CitationNedoma et al. 2003). This substrate is converted to a water-insoluble, crystalline, fluorescent product at the site of enzymatic hydrolysis, thus localizing active enzymes when viewed by fluorescence microscopy (CitationKandeler 2007).
The present study evaluated the physiological importance of APase activities in cluster roots formed under phosphorus-deficient conditions in hydroponic and in soil culture. The approach used involved isoelectric focusing of APase isoforms and histochemical localization and visualization of APase.
MATERIALS AND METHODS
Plant culture
Hydroponic culture
White lupin (Lupinus albus L. cv. Amiga) seeds were sterilized by immersion in 30% H2O2 for 15 min with subsequent washing in deionized water. Sterilized seeds were incubated for 4 h in 10 mmol L−1 CaSO4 and germinated in the dark for 4 days on filter paper soaked with 2.5 mmol L−1 CaSO4. After 3 days in the light, the seedlings were transferred to a nutrient solution (10 plants per 2.5-L pot). Plants were cultivated under controlled environmental conditions in closed growth chambers with a 16/8 h day/night cycle, a light intensity of 300 µmol m−2 s−1, and a 25/20°C day/night temperature regime with a relative humidity of 60%.
The nutrient solution was composed of 2 mmol L−1 Ca(NO3)2, 0.7 mmol L−1 K2SO4, 0.5 mmol L−1 MgSO4, 0.1 mmol L−1 KCl, 1 µmol L−1 H3BO4, 0.5 µmol L−1 MnSO4, 0.5 µmol L−1 ZnSO4, 0.2 µmol L−1 CuSO4, 0.01 µmol L−1 (NH4)Mo7O24, and 20 µmol L−1 Fe-ethylenediaminetetraacetic acid (Fe-EDTA) with (+P) or without (–P) 0.25 mmol L−1 KH2PO4 application in continuously aerated 2.5-L pots. The solution was adjusted to pH 5.6 and renewed every 2 days.
Soil culture
Rhizoboxes (26 cm × 11.5 cm × 1.9 cm) developed by CitationDinkelaker and Marschner (1992) were used for soil culture. White lupin seedlings were grown as described for hydroponic culture. The seedlings were transferred to rhizoboxes (two seedlings per box) that contained 250 g dry matter of a P-deficient calcareous loess sub-soil (CaCO3: 21.5%; pH 7.5; Corg: 0.1%; P (CAL): 3 mg kg−1 soil (CitationSchüller 1969); P (Bray1): 2 mg kg−1 soil (CitationBray and Kurtz 1945); P (Olsen): 1 mg kg−1 soil (CitationOlsen et al. 1954) (representing easily plant-available P fractions); total acid-soluble P (H2SO4): 332 mg kg−1 soil (CitationWalker and Adams 1958) and total organic P: 2 mg kg−1 soil (CitationWalker and Adams 1958); Ntotal: 0.02%; K: 40 mg kg−1 soil), mixed with 10% (w/w) of fresh field soil for microbial inoculation, 50% (w/w) fine quartz sand and fertilizers (described below) and sieved through a 2-mm mesh. Fertilization occurred prior to transplanting the seedlings by applying 100 mg N kg−1 as Ca(NO3)2; 150 mg K kg−1 as K2SO4; 50 mg Mg kg−1 as MgSO4 and 20 µmol Fe kg−1 as Fe-EDTA to the soil, without applying P. Soil moisture was adjusted to 20% (w/v) and appropriate volumes of water were added daily by gravimetric determination. The rhizoboxes were fixed at a horizontal angle of 50° to stimulate root growth along the transparent root observation window of the boxes.
Isoelectric focusing and activity staining of acid phosphatase on a polyacrylamide gel
White lupin plants were hydroponically grown with or without P for 14 days after transfer. Roots were collected, the moisture was removed using paper towels, and the roots were then frozen immediately in liquid nitrogen. Crude protein from the roots was extracted according to CitationWasaki et al. (1997). Five micrograms of crude protein was loaded onto each lane. An additional two white lupin plants cultured in hydroponics with or without P were transferred to a bottle containing 150 mL of 1 mmol L−1 CaCl2 solution and incubated for 24 h in a greenhouse. The solution was collected and filtrated after incubation, then transferred to a clean dialysis tube. The secreted protein was concentrated with polyethyleneglycol up to 1 mL. The rhizosphere soil suspension of cluster roots was prepared according to CitationWasaki et al. (2005). These solutions containing crude protein were used directly for electrophoretic separation, which was carried out using a mini gel system (Mini-Protean III; Bio-Rad Laboratories, Hercules, CA, USA) according to CitationOzawa et al. (1995). In brief, the crude protein was applied on a 5% polyacrylamide gel containing 2% BioLyte (pH range 3–10; Bio-Rad Laboratories) and 10% glycerol and separated for 2 h at 200 V and then for 2 h at 400 V using 20 mmol L−1 AcOH and 25 mmol L−1 NaOH as the cathode and anode solutions, respectively. After electrophoresis, the gel was transferred into a container with staining solution (400 mmol L−1 Citrate-NaOH at pH 5.0 containing 20 mmol L−1 4-methylumbelliferyl phosphate and 20 mmol L−1 EDTA). The fluorescence of methylumbelliferone liberated by phosphatase activity was visualized under ultraviolet light (260 nm). The pI was estimated with a pI marker (Serva Electrophoresis, Heidelberg, Germany).
Activity staining using fluorescent substrate
At 35 days after transplanting (DAT), normal and cluster roots were harvested from the plants grown in hydroponics and in soil. Cluster roots collected from soil were transferred to a microcentrifuge tube containing 1 mL of 75 mmol L−1 Tris-malate buffer (pH 4.3) and washed by gentle agitation several times using forceps and paper towel to remove soil particles and water around the cluster roots. The roots were frozen in optimum cutting temperature (OCT) compound embedding medium at –20°C and sliced at 50 µm thickness using cryostats (Cicrom HM500M; Microm International, Waldorf, Germany or CM3050S; Leica Microsystems, Wetzler, Germany). Sliced root sections were stretched onto a slide glass (Histobond; Marienfeld, Lauda-Königshofen, Germany or Superfrost; Matsunami Glass, Osaka, Japan).
ELF97 phosphate (Invitrogen, Carlsbad, CA, USA) was used as a substrate for APase (CitationNedoma et al. 2003; CitationNedoma and Vrva 2006) in the histochemical activity staining analysis. The buffer for substrate dilution and washing was adjusted to pH 4.3, which was the optimal condition for purified APase secreted from white lupin roots (CitationOzawa et al. 1995). ELF97 phosphate was diluted to 25 µmol L−1 with 75 mmol L−1 Tris-malate buffer. Thirty to fifty microliters of diluted substrate solution was dropped onto the stretched root section and incubated in a dark box at room temperature for 10–20 min. As a negative control, the same volume of Tris-malate buffer without substrate was dropped onto a root section. After the incubation, the substrate solution was removed with a micropipette and washed with the same volume of Tris-malate buffer three times to remove the remaining substrate.
Fifty microliters of Tris-malate buffer was dropped onto the washed root section and covered with a cover glass. ELF97, the hydrolyzed product of APase activity, was observed using fluorescence microscopes (Axiovert 200M; Carl Zeiss, Oberkochen, Germany or Leica FW4000; Leica Microsystems) with 360 and 450 nm for excitation and emission, respectively. Fluorescent images were recorded using high-resolution CCD cameras (AxioCam HRm; Carl Zeiss or Leica DFC300FX; Leica Microsystems). Exposure time was set as short as possible to avoid the influence of autofluorescence. Unstained sections, chosen from the closest part of the stained section, were also captured as a negative control using the same method. Sections captured by the same exposure time were compared to each other. A fluorescent image was merged with the transmitted image.
RESULTS
Profile of APase in white lupin roots and secreted APase
At 14 DAT, the dry matter production of white lupin was similar in –P and +P treated plants. The inorganic phosphate concentration differed between treatments (1.2 and 0.7 mg P per gram dry weight (DW) in +P and –P roots, respectively). The profile of APase isoforms in the roots of white lupin grown in nutrient solution is shown in . These isoforms were detected as 10 independent bands by isoelectric focusing. High activity was detected at pI 4.7, which corresponded to the pI of purified secretory APase (CitationOzawa et al. 1995). Image analysis also revealed that the APase activities of all isoforms were higher in –P than in +P (data not shown).
The APase activity in secreted proteins was also detected with isoelectric focusing (). Activity was low in secreted protein from +P plants grown under hydroponic conditions, but strong at pI 4.7 in –P plants. In contrast, a strong signal at pI 4.7 and a broad and weak signal at a higher pI were detected in the lane of soil suspension prepared from the rhizosphere soil of mature cluster roots ().
Distribution of APase activity in normal roots of white lupin
Histochemical activity staining of APase using a fluorescent substrate was carried out for transverse sections of normal roots of white lupin grown under hydroponic conditions (). As shown in the right panels in , autofluorescence was negligible. The intensity of fluorescence, indicating APase activity, was stronger in –P roots than +P roots. Fluorescence was distributed over the whole section of –P-treated roots (). Specific enrichment of APase was found in the epidermis of –P roots.
Figure 1 Activity staining of APase separated by isoelectric focusing. (A) Profile of APase isoforms in crude protein extracted from roots of hydroponically cultured white lupin. (B) Profile of secreted crude protein from roots of hydroponically cultured white lupin and extracted protein of rhizosphere cluster roots cultured in soil. pI, xxxx.
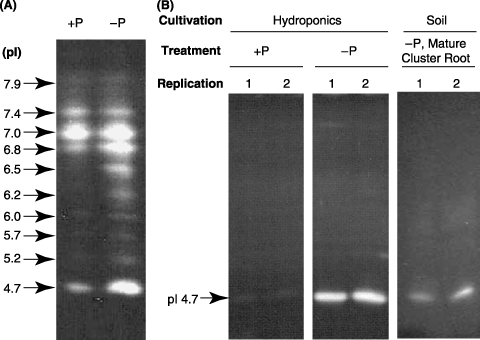
Distribution of APase activity in rootlets of white lupin cluster roots
Histochemical activity staining of APase using a fluorescent substrate was carried out for a vertical section of rootlet of cluster roots formed under hydroponic conditions (). The distribution of APase activity differed considerably between +P and –P rootlets. In the former, activity was weak in vascular tissue, whereas in the latter it was strong both in vascular tissue and in the epidermis (). Fluorescence was most intense in the root tip of rootlets (). Fluorescence in epidermal tissue was higher in the rootlet than in the secondary root, which was the basal root of the cluster rootlets ().
Distribution of APase activity in cluster rootlets of P-deficient white lupin grown under hydroponic and soil conditions
Activity staining of APase for cluster rootlets of P-deficient plants grown under hydroponic and soil conditions were conducted using the method described in the previous section (). In hydroponic plants, activity was strong not only in vascular tissues but also in the epidermis and root hairs, both in vertical and transverse sections (). The APase distribution in vertical sections of cluster rootlets was similar to that in normal roots (,). The activity of cluster rootlets was distributed similarly in hydroponic and soil-cultured plants (). Autofluorescence was negligible in both plant types (), indicating that the fluorescence from APase of root tissue is far stronger than the influence of autofluorescence of soil particles.
DISCUSSION
In a previous study, secretion of APase from lupin roots was induced by decreasing external P; the function essentially depended on inducing LASAP2 expression (CitationWasaki et al. 2003). Therefore, LASAP2 mRNA is accumulated by internal P decrease followed by active secretion of the enzyme. The present study induced APase activities at pI 4.7 and other isoforms (), suggesting that APase isoforms involved in internal P recycling were also induced after internal P concentrations dropped. The response of each APase isoform to P nutrition has been described: CitationBosse and Köck (1998) examined the pattern of these isoforms in tomato roots and showed that the activity of one root-specific isoform increased and a new isoform appeared. Using Arabidopsis as a model plant, only one of five isoforms was induced under low P conditions (CitationTrull et al. 1997). Our results revealed that low P availability induced many isoforms of APase.
A strong signal at pI 4.7 was detected both in secreted proteins and in a suspension of the rhizosphere soil of mature cluster roots (). This indicates that the major activity of APase secreted from cluster roots is LASAP2 at pI 4.7. Only a broad and weak signal at higher pI was detected in the lane of soil suspension (). APase activity from other isoforms of plants and microbes contributed very little to the phosphorus mineralization in the rhizosphere of cluster roots.
APase activity at pH 4.3 was high in the epidermis of normal roots and cluster rootlets (,,). This APase fraction was probably responsible for Pi release from the organic substrate in the rhizosphere. A previous study of CitationWasaki et al. (1997) using histochemical immunostaining provides evidence that this APase fraction at pH 4.3 is accumulated in epidermal cells. Therefore, LASAP2 occupies a large proportion of APase acting in the rhizosphere of white lupin. In addition, cluster root formation is significantly induced under low P conditions (CitationGardner et al. 1983; CitationNeumann and Martinoia 2002; CitationShane and Lambers 2005; CitationWasaki et al. 2005). APase activity in the rhizosphere and the accumulation of mRNA for secretory APase were high in cluster roots (CitationWasaki et al. 2003, Citation2005). A higher activity in the epidermis of cluster rootlets supports these results (). In white lupin, increased cluster root formation and APase secretion could contribute to Pi release from organic P compounds and Pi uptake.
Figure 2 Visualization of the histochemical activity of APase for normal roots using ELF97 phosphate as a substrate. Hydroponically cultured white lupin roots were used for this experiment. Fluorescent precipitates of ELF97 (green color), the product of phosphatase activities, were observed using a fluorescence microscope. The results of the activity staining and autofluorescence are shown in the left and right panels, respectively. Upper and lower panels indicate the transverse sections of normal roots under +P and –P conditions, respectively.
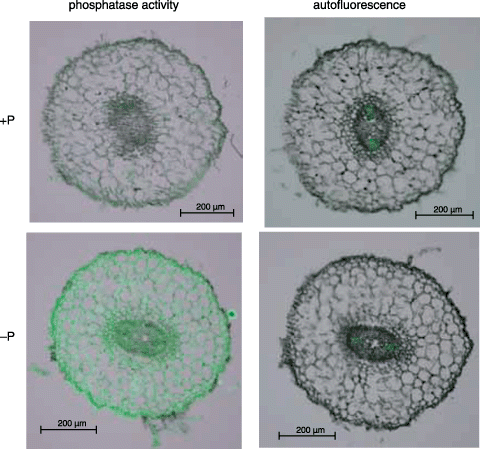
Figure 3 Visualization of the histochemical activity of APase for cluster rootlets using ELF97 phosphate as a substrate. Hydroponically cultured white lupin roots were used for this experiment. The results of the activity staining and autofluorescence are shown in the left and right panels, respectively. Upper and lower panels indicate the cluster rootlets formed under +P and –P conditions, respectively.
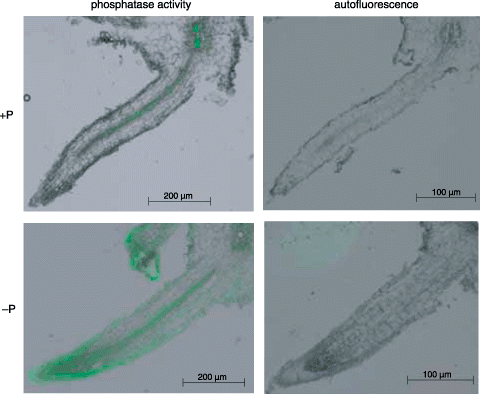
Figure 4 Visualization of the histochemical activity of APase for cluster rootlets formed under –P conditions using ELF97 phosphate as a substrate. Hydroponically and soil-cultured white lupin roots were used for this experiment. The results of the activity staining and autofluorescence are shown in the left (A,C,E) and right (B,D,F) panels, respectively. (A,B) Vertical sections of a cluster rootlet in a hydroponic plant; (C,D) transverse sections of a cluster rootlet in a hydroponic plant; (E,F) transverse sections of a cluster rootlet in a soil culture plant. Triangles indicate typical root hairs.
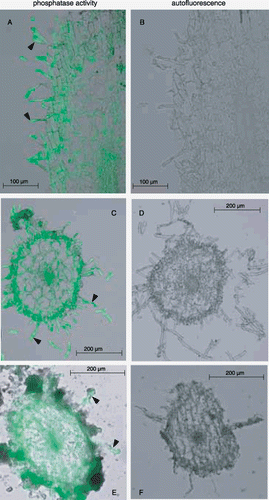
Clear APase activity took place at pI 4.7 in +P crude root extracts, whereas it was very low in +P crude secreted protein (). CitationWasaki et al. (2003) have shown that LASAP2 mRNA accumulated only in –P roots. A more constitutive APase activity representing an isoform differing from LASAP2 probably exists at pI 4.7. This isoform might correspond to LASAP1, which has been isolated and characterized as a probable cell wall or plasma membrane binding protein in a previous study (CitationWasaki et al. 1999).
The promoter region of secretory APase has been isolated and characterized from Arabidopsis thaliana (CitationHaran et al. 2000). Their experiment showed the strongest expression in the meristematic regions of lateral roots, corresponding to our result for cluster rootlets (). Those authors also showed that P starvation induced expression of the reporter gene in vascular tissue soon after germination; within 10 days this extended throughout most of the root tissue. This finding corresponds to our results: high activity in the central cylinder of the cluster rootlet under P deficiency and distribution of the activity in the whole rootlet (). High APase activities in lateral roots of Arabidopsis also correspond to the strategy of white lupin for P acquisition by increasing tertiary lateral rootlets, that is, cluster root formation under low P conditions.
APase activity was very strong in the aquatic plant duckweed Spirodela oligorrhiza, mainly because of the production of a glycosylphosphatidylinositol (GPI)-anchored APase (CitationMorita et al. 1996). Immunostaining using polyclonal antibody to purify GPI-anchored APase revealed that this enzyme is located in the outermost cortical cells of the roots (CitationNishikoori et al. 2001). Such APase is apparently present in root surfaces and contributes to the P acquisition of S. oligorrhiza. In this plant, the main phosphatase activity was localized near the root surface and was enriched in the cell wall (CitationBieleski and Johnson 1972), minimizing enzyme loss to the aquatic environment. In contrast, white lupin produces cell wall-bound APase and other APases, which are released into the rhizosphere. An APase isoform secreted from roots of white lupin grown in soil was also high (). Therefore, the activities of inducible secreted APase as well as the relatively constitutive epidermal APase might help white lupin acquire P.
In conclusion, APase secreted by cluster roots plays an important role in P uptake under P-deficient conditions. Moreover, other isoforms of APase induced under P deficiency might be responsible for P translocation within roots and cluster roots.
ACKNOWLEDGMENTS
This research has been partly supported by the Special Coordination Funds for Promoting Science and Technology and a Grant-in-Aid (17688004) from the Ministry of Education, Culture, Sports, Science and Technology, Japan.
Notes
Present address: †Plant Science Center, RIKEN Yokohama Institute, 1-7-22 Suehiro-cho, Tsurumi-ku, Yokohama 230-0045, Japan.
REFERENCES
- Bieleski , RL and Johnson , PN . 1972 . The external location of phosphatase activity in phosphorus-deficient Spirodela oligorrhiza . Aust. J. Biol. Sci , 25 : 707 – 720 .
- Bosse , D and Köck , M . 1998 . Influence of phosphate starvation on phosphohydrolases during development of tomato seedlings . Plant Cell Environ , 21 : 325 – 332 .
- Bray , RH and Kurtz , LT . 1945 . Determination of total, organic and available forms of phosphorus in soils . Soil Sci , 59 : 39 – 45 .
- Dinkelaker , B and Marschner , H . 1992 . In vivo demonstration of acid phosphatase activity in the rhizosphere of soil-grown plants . Plant Soil , 144 : 199 – 205 .
- Dinkelaker , B , Römheld , V and Marschner , H . 1989 . Citric acid excretion and precipitation of calcium citrate in the rhizosphere of white lupin (Lupinus albus L.) . Plant Cell Environ , 12 : 285 – 292 .
- Gardner , WK , Barber , DA and Parbey , DG . 1983 . The acquisition of phosphorus by Lupinus albus L. III. The probable mechanism by which phosphorus movement in the soil/root interface is enhanced . Plant Soil , 70 : 107 – 124 .
- Haran , S , Logendra , S , Seskar , M , Bratanova , M and Raskin , I . 2000 . Characterization of Arabidopsis acid phosphatase promoter and regulation of acid phosphatase expression . Plant Physiol , 124 : 615 – 626 .
- Kandeler , E . 2007 . “ Physiological and biochemical methods for studying soil biota and their function ” . In Soil Microbiology, Biochemistry and Soil Ecology , 3rd edn. , Edited by: Paul , EA . 53 – 83 . San Diego : Elsevier .
- Liu , J , Uhde-Stone , C , Li , A , Vance , C and Allan , D . 2001 . A phosphate transporter with enhanced expression in proteoid roots of white lupin (Lupinus albus L.) . Plant Soil , 237 : 257 – 266 .
- Miller , SS , Liu , J , Allan , DL , Menzhuber , CJ , Fedorova , M and Vance , CP . 2001 . Molecular control of acid phosphatase secretion into the rhizosphere of proteoid roots from phosphorus-stressed white lupin . Plant Physiol , 127 : 594 – 606 .
- Morita , N , Nakazato , H , Okuyama , H , Kim , Y and Thompson , GA Jr . 1996 . Evidence for a glycosylinositolphospholipid-anchored alkaline phosphatase in the aquatic plant Spirodela oligorrhiza . Biochim. Biophys. Acta , 1290 : 53 – 62 .
- Nedoma , J and Vrva , J . 2006 . Specific activity of cell-surface acid phosphatase in different bacterioplankton morphotypes in acidified mountain lake . Environ. Microbiol , 8 : 1271 – 1279 .
- Nedoma , J , Strojsova , A , Vrba , J , Komarkova , J and Simek , K . 2003 . Extracellular phosphatase activity of natural plankton studied with ELF97 phosphate: fluorescence quantification and labeling kinetics . Environ. Microbiol , 5 : 462 – 472 .
- Neumann , G and Martinoia , E . 2002 . Cluster roots – an underground adaptation for survival in extreme environments . Trends Plant Sci , 7 : 162 – 167 .
- Neumann , G , Massonneau , A Langlade , N . 2000 . Physiological aspects of cluster root function and development in phosphorus-deficient white lupin (Lupinus albus L.) . Ann. Bot , 85 : 909 – 919 .
- Nishikoori , M , Washio , K , Hase , A , Morita , N and Okuyama , H . 2001 . Cloning and characterization of cDNA of the GPI-anchored purple acid phosphatase and its root tissue distribution in Spirodela oligorrhiza . Physiol. Plant , 113 : 241 – 248 .
- Olsen , SR , Cole , CV , Watanabe , FS and Dean , LA . 1954 . Estimation of available phosphorus in soils by extraction with sodium bicarbonate , Washington : US Department of Agriculture . Circ. 939
- Ozawa , K , Osaki , M , Matsui , H , Honma , M and Tadano , T . 1995 . Purification and properties of acid phosphatase secreted from lupin roots under phosphorus-deficiency conditions . Soil Sci. Plant Nutr , 41 : 461 – 469 .
- Schüller , H . 1969 . Die CAL-Methode zur Bestimmung des pflanzenverfügbaren Phosphates in Böden . Z. Pflanzenernaehr. Bodenkd , 123 : 48 – 63 . (in German)
- Shane , MW and Lambers , H . 2005 . Cluster roots: a curiosity in context . Plant Soil , 274 : 101 – 125 .
- Tadano , T and Komatsu , K . 1994 . Utilization of organic phosphorus in soil by plant roots . Proc. Trans. 15th World Congr. Soil Sci , 9 : 521 – 522 .
- Tadano , T and Sakai , H . 1991 . Secretion of acid phosphatase by the roots of several crop species under phosphorus-deficient conditions . Soil Sci. Plant Nutr , 37 : 129 – 140 .
- Tadano , T , Ozawa , K , Sakai , H , Osaki , M and Matsui , H . 1993 . Secretion of acid phosphatase by the roots of crop plants under phosphorus-deficient conditions and some properties of the enzyme secreted by lupin roots . Plant Soil , 155/156 : 95 – 98 .
- Trull , MC , Guiltinan , MJ , Lynch , JP and Deikman , J . 1997 . The response of wild type and ABA mutant Arabidopsis thaliana plants to phosphorus starvation . Plant Cell Environ , 20 : 85 – 92 .
- Walker , TW and Adams , AFR . 1958 . Studies on soil organic matter: I. Influence of phosphorus content of parent materials on accumulation of carbon, nitrogen, sulfur, and organic phosphorus in grassland soils . Soil Sci , 85 : 307 – 318 .
- Wasaki , J , Ando , M Ozawa , K . 1997 . Properties of secretory acid phosphatase from lupin roots under phosphorus-deficient conditions . Soil Sci. Plant Nutr , 43 : 981 – 986 .
- Wasaki , J , Omura , M Ando , M . 2000 . Molecular cloning and root specific expression of secretory acid phosphatase from phosphate deficient lupin (Lupinus albus L.) . Soil Sci. Plant Nutr , 46 : 427 – 437 .
- Wasaki , J , Omura , M Osaki , M . 1999 . Structure of a cDNA for an acid phosphatase from phosphate-deficient lupin (Lupinus albus L.) roots . Soil Sci. Plant Nutr , 45 : 439 – 449 .
- Wasaki , J , Rothe , A Kania , A . 2005 . Root exudation, P acquisition and microbial diversity in the rhizosphere of Lupinus albus as affected by P supply and atmospheric CO2concentration . J. Environ. Qual , 34 : 2157 – 2166 .
- Wasaki , J , Yamamura , T , Shinano , T and Osaki , M . 2003 . Secreted acid phosphatase is expressed in cluster roots of lupin in response to phosphorus deficiency . Plant Soil , 248 : 129 – 136 .
- Present address: †Plant Science Center, RIKEN Yokohama Institute, 1-7-22 Suehiro-cho, Tsurumi-ku, Yokohama 230-0045, Japan.