Abstract
Crop residues are important agricultural resources and incorporating wheat straw into rice fields is becoming an alternative to open-field burning after wheat harvest in rice–wheat rotation systems. Organic acids are important soil constituents that are believed to be linked to many biological and environmental processes in the soil, including nutrient cycling, phytotoxicity, metal solubility and greenhouse gas formation. A number of studies have focused on the impact of wheat straw incorporation on organic acid accumulation under flooded soil conditions, but information on the relationship between organic acid accumulation and the properties of wheat straw, especially the C:N ratio, and the response of organic acid accumulation to N application is rare. In this study, incubation experiments were conducted to investigate the difference in organic acid (formic, acetic, propionic and butyric acids) accumulation in soil solution under flooded conditions between wheat and rice straw incorporation, and the relationship between the C:N ratio of the straw materials and organic acid accumulation. Results showed that the concentration of organic acids in the soil solution increased with the rate of straw incorporated. The overall accumulation of organic acids was higher when soil was incorporated with wheat straw than with rice straw. The NH+ 4 concentration in soil solution decreased more with wheat straw incorporation because of its higher C:N ratio. The addition of urea-N significantly enhanced CH4 flux and reduced the concentration of organic acids in soil solution in the wheat straw treatment, whereas CH4 flux and the concentration of organic acids in the rice straw treatment were less sensitive to the addition of urea-N. The practical implication of the results obtained in this study to N management in rice fields is also discussed.
INTRODUCTION
Low molecular weight organic acids (LMWOAs) are important soil constituents that are believed to be involved in many chemical and biological reactions and processes in soils and soil solutions. CitationStrobel (2001) summarized that the anionic forms of LMWOAs functioned as ligands and affected the behaviors of metal cations in soils. Many studies have focused on LMWOAs in the soil rhizosphere for their involvement in plant and microbial nutrition, heavy metal decontamination and other rhizosphere processes (CitationJones 1998; CitationJones et al. 2003).
In general, LMWOAs originate from plant litter breakdown, root exudation and organic matter decomposition (CitationFox 1995; CitationJones 1998; CitationTsutsuki 1984). Under flooded conditions, such as in submerged rice paddy soils, a wide variety of reduced organic metabolites, including LMWOAs, are produced at low redox potential. Acetate, formate and other organic acids are indicative of anaerobic processes (CitationGottschalk 1986; CitationLynch 1980). Of the LMWOAs derived from the fermentation process, formic, acetic, propionic and butyric acids are produced in the largest quantities (CitationChandrasekaran and Yoshida 1973; CitationGotoh and Onikura 1971; CitationRao and Mikkelsen 1977b; Citationde Sousa et al. 2002; CitationWang et al. 1967). These organic acids may cause agronomic concern if they accumulate to a toxic level, although they are generally converted to CO2 or CH4 after a short residence time (CitationAngeles et al. 2006; CitationJones and Darrah 1994).
World crop production has been greatly improved by advances in crop science and technology; meanwhile, vast amounts of crop residues are produced. Open-field burning of crop residues after harvest can cause the loss of organic matter and produce air pollutants. Directly incorporating rice straw into paddy soil is common practice in rice–rice rotation systems (e.g. in tropical Asia), and rice plants might be subject to the toxic effect of the organic acids produced during the anaerobic decomposition of the added rice straw. CitationRao and Mikkelsen (1977a) reported that the order of greater inhibition to root growth of young rice seedling was: butyric > propionic > acetic. The amount of organic acids increased with the rate of the added rice straw (CitationGotoh and Onikura 1971) and peak production occurred between 15 and 20 days after incubation (CitationRao and Mikkelsen 1977b). In wheat–rice rotation systems, for example, in the mid-lower reaches of the Yangtze River, China, there is generally no time for the pre-decomposition of wheat straw before rice transplanting because of seasonal confliction between the crops in the intensified cropping systems. Thus, a detrimental effect of organic acids on rice seedlings as a result of wheat straw incorporation may occur. CitationChidthaisong et al. (1996a) compared the difference in soil organic acid accumulation and methane production between wheat and rice powder incorporation in a sealed incubation using flooded soil with continuous straw application. However, very little information is known about the effect of various wheat straw properties, especially C:N ratio, on organic acid accumulation and its relationship with N supply when large amounts of wheat straw are incorporated into rice fields.
The quality of the substrate for microbes is one of the most important factors impacting on the degradation of plant residues incorporated into soil (CitationPaul and Clark 1996). The turnover rate of plant residues during the decomposition process is affected by the C:N ratio of the residue materials (CitationCheshire and Chapman 1996; CitationOades 1988). Organic acids, as well as other reduced substances, are the intermediate metabolites derived from anaerobic decomposition of incorporated plant residue and can be used further by soil microbes as a carbon source. The accumulation of organic acids at any moment during decomposition depends on the balance between their production and consumption and they accumulate when their production exceeds consumption. This balance may be influenced by the C:N ratio of the residue impacting on the available N level in soil during early decomposition of incorporated residue materials. Wheat straw and rice straw usually have different C:N ratios. The objectives of the present study were: (1) to identify the difference in organic acid accumulation in soil solution between wheat and rice straw incorporation under flooded conditions, (2) to understand the relationship between organic acid accumulation and gas emission, (3) to investigate the link between organic acid accumulation and the C:N ratios of wheat and rice straw.
MATERIALS AND METHODS
Experimental design and sampling
Two incubation experiments, EXP 1 and EXP 2, were conducted at the International Rice Research Institute (IRRI) and Yangzhou University (YU), China, respectively. The soil used in EXP 1 was collected from the plow layer (0–15 cm) of a rice field at the IRRI experimental station. The soil had a pH of 6.5 (1:1 soil/water) and the total C was 1.845%, total N was 0.158%, cation exchange capacity (CEC) was 36.4 cmol kg−1 soil and Olsen P was 79.7 mg kg−1 soil. The soil used in EXP 2 was from a rice field at the experimental farm in Yangzhou University and it had a pH of 6.8 and total C was 1.623%, total N was 0.147%, Olsen P was 65.3 mg kg−1 soil and CEC was 28.6 cmol kg−1 soil. Neither soil was treated with any straw before rice transplanting. Non-soil debris was removed immediately after collection from the rice field and the puddled soils were mixed thoroughly without air-drying for uniform consistency before use.
Rice straw (var. IR68) and wheat straw (var. Yangmai 158) sampled at harvest were used in this study and were chopped into 1–2 cm lengths before use. The composition of the straw materials is listed in .
The objective of EXP 1 was to investigate the response of organic acid accumulation in soil solution to the rate of straw added, and to identify any difference in organic acid accumulation between wheat and rice straw incorporation under flooded soil conditions. The treatments (with three replications) were rice and wheat straw with a rate of 0, 0.5, 1.0 or 2.0% (of dried soil, w/w). Three hundred and ten grams of soil slurry (wet weight with a moisture content of 0.55 g water g−1 dry soil) was distributed into a plastic container (12 cm high and 10.5 cm in diameter) and thoroughly mixed with the straw materials. The soil mixture in the container was approximately 6 cm thick. The porous tube of a Rhizon solution sampler (Rhizosphere Research Products, Wageningen, The Netherlands) was horizontally installed into the soil layer (at approximately 4 cm in depth) for organic acid sampling (CitationAngeles et al. 2006). The water to soil (dry base) ratio in the container was 1.5:1. The water layer in the container was approximately 2 cm thick and was maintained by adding water during incubation. The incubation temperature was 30°C. The soil solution (5 mL in volume) was sampled at 7, 10 and 14 days after the incubation started.
Table 1 Composition of the straw materials used in the incubation experiments
Two treatments with three replications were used in EXP 2: one treatment was straw type, rice and wheat straw (the rate added for both was 2%) with no straw as a control, and the second treatment was the addition of N at a rate of 0, 0.2 and 0.4 g urea kg soil−1. The procedure and incubation conditions in EXP 2 were identical to those used in EXP 1. Soil solution and gas (CO2 and CH4) sampling were conducted at 1, 3, 5, 7, 10, 14 and 21 days after incubation. The redox potential and pH were measured at each sampling time. Before gas sampling, the gas bubble (developed during incubation) trapped in the soil was removed by gently stirring the soil mixture in the container. The initial gas sample was obtained immediately after the container was stoppered using a rubber stopper and 30 min later a second sample was taken. The volume of the space between the water surface and the rubber stopper was measured. Gas flux during incubation was calculated using the following equation:
After gas sampling, the rubber stopper was removed from the container.
Measurements
Organic acids in soil solution
Organic acids (formic, acetic, propionic and butyric acids) were analyzed using high performance liquid chromatography (HPLC). The soil solution was filtered through a 0.45 µm membrane filter (Millex-HV, PVDF, Warsaw, Poland) before HPLC analysis. Separation of the organic acids was achieved by using an ion exclusion column (Shodex RS-Pak KC-811 column; Waters Corporation, Milford, MA, USA) with 0.0033 mol L−1 H3PO4 as the mobile phase. Organic acids were quantified using an ultraviolet–visible (UV-VIS) spectrum detector (Waters 2487 Dual Absorbance detector) attached to the column. Other conditions for the HPLC analysis were: column temperature 40ºC; flow rate 0.75 mL min−1; wavelength 210 nm and run time 20 min. Jasco–Borwin interface software (v.1.5) (JMBS Developments 1998) was used to report peak information about the organic acids. It was necessary to manually assign peak names according to the retention time and to manually adjust baselines to remove noise from the soil solution samples (CitationAngeles et al. 2006). The soil solution was diluted if the concentrations of the organic acids exceeded the loading capacity of the ion exclusion column.
Carbon dioxide and methane
The CO2 and CH4 concentration in the gas samples was analyzed by gas chromatography (GC; Shimadzu GC 12A, Kyoto, Japan) using a thermal conductivity detector (TCD; Hitachi 023, Hitachi, Tokyo, Japan) and a Porapak-QS column (Waters Corporation) for CO2 detection and a flame ionization detector (FID) and a 2-m Porapak Q (80/100 mesh) column for CH4 (CitationDing et al. 2002).
Ammonium in the soil solution
The NH+ 4 concentration in the soil solution sample was determined using the colorimetric method. Indophenol blue complex, derived from the reaction between ammonia and alkaline phenol and hypochlorite, was detected using a UV-VIS spectrophotometer (Beckman Du 520, Fullerton, California, USA).
Statistical analysis
Data analysis, including anovas and multiple comparisons (least significant difference [LSD]), was conducted using the SAS system (v.9.01; SAS Corporation, Cary, North Carolina, USA).
RESULTS
Response of organic acid accumulation to the rate and type of straw material added
In EXP 1 organic acids in the soil solution were tested at 7, 10 and 14 days after incubation (). As no organic acids were detectable, data for the control without straw incorporation were not included. Organic acids in the soil solution for both the wheat and rice treatments accumulated at higher concentrations at 7 and 10 days of incubation, and remained in relatively low concentrations or disappeared at 14 days of incubation.
Figure 1 Effect of straw type and rate on the concentration of organic acids in soil at different incubation times.
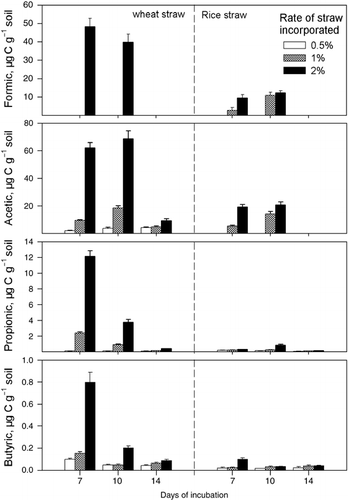
The concentration of individual organic acids in the soil solution responded to the rate of straw incorporated. Formic acid was not detectable at incorporation rates of 0.5 and 1% in the treatment with wheat straw incorporation and formic and acetic acids were also undetectable at 0.5% incorporation rate for rice straw (). The highest concentrations of organic acids appeared at the 2% incorporation rate for both wheat and rice straw.
shows that the total accumulation of the four organic acids tested increased with the rate incorporated. When 0.5% straw was added, there was no significant difference in total organic acid accumulation between wheat and rice straw incorporation. However, the total concentration of organic acids in the soil solution was significantly higher in the wheat straw treatment than in the rice straw treatment at 7 and 10 days after incubation when 1 or 2% of straw was incorporated. At 14 days of incubation, the total concentration of the organic acids was significantly higher in the wheat straw treatment with 2% straw incorporation than in the rice straw treatment, but there was no difference between the two treatments when straw was incorporated at lower rates.
Table 2 Comparison of the total concentration (µg C g−1 soil) of the four organic acids at each sampling date between the two straw materials
Table 3 Soil pH value in the different straw treatments over the incubation period†
Effect of straw incorporation on soil pH and redox potential
The soil pH values for different sampling dates in EXP 2 are listed in . Straw incorporation did not affect soil pH during the early incubation period and significantly lowered soil pH during the late incubation period. However, no significant difference in soil pH between rice and wheat straw incorporation was found over the whole incubation period.
Soil redox potential in all treatments declined sharply when incubation started, and was relatively stable after approximately 1 week of incubation (). Straw incorporation significantly lowered soil redox potential, but straw type had no effect on soil redox potential.
Change in NH+ 4 concentration in the soil solution
The reduction in the NH+ 4 concentration in the soil solution with straw incorporation compared with that without straw incorporation was indicative of the immobilization of soil-available N. With no urea added, the NH+ 4 concentration in the soil solution was significantly lower in the rice straw incorporation treatment than in the no straw treatment after approximately 5 days of incubation, whereas a reduction in NH+ 4 concentration in the wheat straw incorporation occurred from the beginning of incubation (). The NH+ 4 concentration in the soil solution incorporated with wheat straw was significantly lower than that with rice straw over the whole incubation process. This indicates that wheat straw incorporation results in more severe immobilization of soil-available nitrogen, which leads to lower NH+ 4 concentration in the soil solution, compared with rice straw incorporation. With the addition of urea and its hydrolysis, the NH+ 4 concentration in the soil solution with straw incorporated maintained a relatively high level despite the immobilization of the available nitrogen ().
Response of organic acid accumulation to the addition of nitrogen
Although a different soil was used, the trend that wheat straw incorporation resulted in the accumulation of more organic acids in the soil solution was confirmed in EXP 2 (). Organic acid accumulation in the soil solution incorporated with wheat straw was more sensitive to the addition of N. In the wheat straw incorporation treatment, the concentration of organic acids was significantly reduced by the addition of N, but there was no significant difference between N rates of 0.2 and 0.4 g urea kg−1 soil at 14 days of incubation for acetic, propionic and butyric acids (). At 10 days of incubation, the concentration of formic, acetic, propionic and butyric acids without the addition of N was 21.178, 33.44, 6.64 and 0.56 µg C g−1 soil, respectively, and declined to 12.76, 15.40, 2.43 and 0.23 µg C g−1 soil, respectively, when 0.4 g urea kg−1 soil was added.
As to the incorporation of rice straw, the concentration of propionic acid did not respond to the addition of urea-N. The addition of N significantly reduced the concentration of formic, acetic and butyric acids at 10 days of incubation in the rice straw incorporation treatment, but the differences observed with and without urea-N addition were much smaller compared with the differences observed in the wheat straw incorporation.
It is worthwhile noting that the overall accumulation of organic acids in the soil solution with the addition of urea-N was still higher in the incorporation with wheat straw than that with rice straw (). This implies that there may be other factor(s), along with available N, that are responsible for the higher accumulation of organic acids associated with wheat straw incorporation (,).
Response of CO2 and CH4 flux to the addition of nitrogen
The CH4 flux in the rice incorporation treatment was higher than that in the wheat straw treatment when no urea-N was added (). Nitrogen addition significantly enhanced the CH4 flux during the incubation period in the treatment with wheat straw incorporation. It is worthwhile noting that the peak CH4 flux appeared at 15 days of incubation, after which the flux decreased more in the wheat straw treatment with N added, and at 20 days of incubation the fluxes of the different urea rates became closer. The CH4 flux in the rice straw treatment also increased with the rate of N added, but the difference with and without the addition of N was smaller compared with that in the wheat straw treatment. shows that the peak CO2 flux in the rice straw treatment appeared earlier than in the wheat straw treatment, indicating a faster decomposition rate of rice straw. The relationship between CO2 flux and the addition of urea-N was not clear in either the wheat treatment or the rice treatment.
DISCUSSION
The degradation process of plant residues and the activity and size of soil microbes are dependent on the quality of the substrates for the soil microbes (CitationPaul and Clark 1996). The C:N ratio is often used to explain factors influencing the turnover rate of plant residues during the decomposition process (CitationCheshire and Chapman 1996; CitationOades 1988). The C mineralized in the flooded conditions was mainly from labile pools with significant amounts coming from more recalcitrant pools, such as cellulose and lignin (CitationDevevre and Horwath 2000). The order of decomposition and acetic acid accumulation was: glucose > starch > gelatin > cellulose (CitationYamane and Sato 1967). The addition of glucose increased organic acid accumulation in the soil without continuous straw application (CitationChidthaisong et al. 1996b). In our study, the amount of cellulose, hemi-cellulose and lignin in the wheat straw was relatively close to that in the rice straw (), but rice straw contains more soluble sugar than wheat straw, which might lead to faster production of organic acids. However, higher accumulation of organic acids was found in the wheat straw treatment compared with the rice straw treatment, which indicated that the utilization of organic acids by microbes was more inhibited by the application of wheat straw. The C:N ratio of wheat straw almost doubled that of rice straw (). It is generally agreed that organic matter with a higher C:N ratio has a lower decomposition rate. As shown in and however, the overall accumulation of organic acids in soil solution with wheat straw was much higher than that with rice straw, which indicated that the higher accumulation in wheat straw was related to the higher C:N ratio of wheat straw.
Figure 5 Effect of straw type and N addition on CH4 flux. (A) Wheat straw and (B) rice straw. N0, N1 and N2 refer to 0, 0.2 and 0.4 g urea kg−1 soil, respectively. Errors bar are standard error.
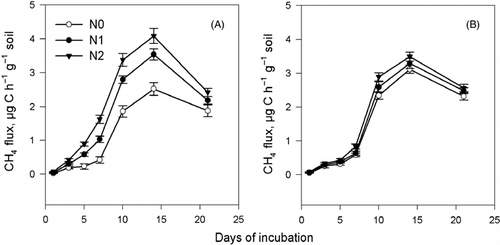
Figure 6 Effect of straw type and N addition on CO2 flux. (A) Wheat straw and (B) rice straw. N0, N1 and N2 refer to 0, 0.2 and 0.4 g urea kg−1 soil, respectively. Errors bar are standard error.
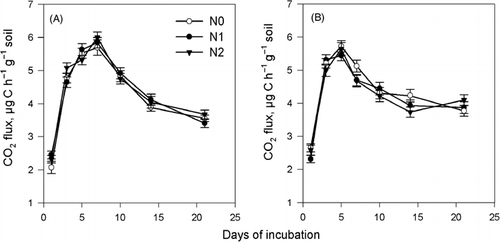
Organic acids are intermediate products formed during the anaerobic decomposition process of straw incorporated into soil and can be used by soil microbes as a C source and converted to CO2 and CH4 after a short residence time (CitationAngeles et al. 2006; CitationJones and Darrah 1994). The formation of CH4 in rice fields could be considered to be an approach for organic acid detoxication (CitationChandrasekaran and Yoshida 1973). CitationKrylova et al. (1997) reported a close relationship between propionate consumption and CH4 production in a paddy soil amended with straw. Organic acids accumulate if their production is greater than their consumption. Of the two steps, organic acid production and consumption, the latter may be more sensitive to the deficiency of available N because of the immobilization during the early decomposition of incorporated straw. It is also accepted that more available N immobilization occurs during early decomposition when organic matter with a higher C:N ratio is incorporated into the soil. In EXP 1, we did not add N to the soil. In this case, more indigenous available N was immobilized in the soil incorporated with wheat straw than that with rice straw, which may affect the balance between production and consumption of organic acids and may contribute to the difference in organic acid accumulation between wheat and rice incorporation. In EXP 2, wheat straw incorporation caused more severe immobilization of available N because of its higher C:N ratio (). This may restrain the gas formation process, which may partly contribute to the higher accumulation of organic acids in the wheat straw treatment. The addition of urea alleviated the deficiency of available N () and significantly reduced organic acid accumulation in the wheat straw treatment (). The fermentation process became predominant at low redox potential (CitationInubushi et al. 1984). In our study, redox potential in the straw treatment decreased sharply after incubation started, which enhanced the fermentation process and the production of organic acids. The reduction in the concentration of organic acids in the soil solution was closely linked to the increase in CH4 (rather than CO2) flux because both CH4 flux and organic acid accumulation responded to the addition of urea, whereas urea addition did not affect CO2 flux (,), which indicated that the release of CH4 increased the consumption of organic acids and reduced their accumulation. The pH and redox potential in flooded soil may also affect organic acid accumulation. In our experiment, however, no apparent difference in pH and redox potential between treatments with wheat straw or rice straw was found, although pH and redox potential was lowered by the incorporation of straw (, ).
In a wheat–rice rotation system, directly returning wheat straw to the rice field is becoming an alternative to open-field burning. There is usually no time for the pre-decomposition of wheat straw returned before rice transplanting because of seasonal conflict in many areas, for example, the mid-lower reaches of the Yangtze River in China. In this case, a detrimental effect pertaining to organic acid accumulation on young rice seedlings may occur, especially when soil-available N is low. CitationTanaka and Nishida (1996) reported that the reduction of N uptake in rice plants at an early growth stage was not associated with biological N immobilization, but with inhibition in N uptake, although N immobilization was enhanced by wheat straw application. However, the microbes using organic acids to form CH4 might be more sensitive to the level of available N in the soil. Increasing the rate of N fertilizing in the basal application greatly reduced the initial growth inhibition of paddy rice caused by wheat straw application (CitationWakimoto and Seino 1983), which implied that the addition of N eased some detrimental effects, possibly including organic acid accumulation, by wheat straw incorporation. The results obtained in the present study suggest that applying a sufficient amount of chemical N fertilizer is one option to reduce the danger from organic acid accumulation.
Many previous studies have emphasized the toxic level of organic acids to rice seedlings (CitationTakijima 1965; CitationTanaka and Navasero 1967). CitationRao and Mikkelsen (1977a) reported that at a concentration of 1 mmol L−1 all organic acids evaluated reduced root length by 40–50%, and at 10 mmol L−1 concentration both root elongation and initiation were completely inhibited. In our study, wheat straw incorporation exhibited higher organic acid accumulation. The highest concentration of formic and acetic acids in the soil solution in wheat straw treatments was 2.45 and 1.75 mmol L−1, respectively, which reached the toxic level reported in previous studies, whereas propionic and butyric acids did not accumulate to toxic levels (). In wheat–rice rotation systems, the incorporation rate of wheat straw seldom exceeds 5 t ha−1 (ca. 0.5% of straw application in plow soil), which is much lower than the rate in this study and in many previous studies. However, damage by organic acids to rice plants may still occur because of uneven incorporation of the wheat straw in some regions because of a lack of labor or relevant machinery. The accumulation of some organic acids (e.g. acetic and butyric acids) decreased with increasing temperature (CitationYamane and Sato 1967) and a lower temperature in the early growth stage may also increase the danger of organic acid accumulation. Aromatic acids caused inhibitory effects on rice growth in solution culture (CitationTanaka and Nishida 1998) and their relationship with wheat straw application and N supply requires further study.
In the present study, the addition of urea-N reduced organic acid accumulation in soil solution, but enhanced CH4 flux, which may cause environmental concerns. The addition of urea stimulated CH4 flux, which might be related to the organic C in the urea (CitationCai et al. 2007); however, more CH4 was released from the wheat straw treatment, which indicated that such a change was more likely to be linked to available N in the soil. In our study, the higher CH4 flux as a result of the addition of urea-N lasted for approximately 3 weeks (). The contribution of such enhancement of CH4 flux to overall CH4 emission over longer incubation periods needs further study.
In conclusion, compared with rice straw incorporation, wheat straw incorporation exhibited higher organic acid accumulation in soil solution under flooded conditions because of the higher C:N ratio in the wheat straw. The addition of urea-N reduced organic acid accumulation by enhancing the conversion of organic acids to CH4 flux.
ACKNOWLEDGMENTS
This study was supported by National Basic Research Program of China (also called 973 Program), the international collaborative project “Managing Crop Residues for Healthy Soils in Rice Ecosystems” sponsored by the German Ministry of Economic Cooperation and Development and a fund from the Key Lab of Environmental Materials and Engineering in Jiangsu Province, China. The authors thank Ms O.R. Angeles and Mr S. Pantoja from the Division of Crop, Soil and Water Science of the International Rice Research Institute for their technical support in soil solution sampling and organic acid measuring.
REFERENCES
- Angeles , OR , Johnson , SE and Buresh , RJ . 2006 . Soil solution sampling for organic acids in rice paddy soils . Am. J. Soil Sci. Soc , 70 : 48 – 56 .
- Cai , ZC , Shan , YH and Xu , H . 2007 . Effects of nitrogen fertilization on CH4emissions from rice fields . Soil Sci. Nutr , 53 : 353 – 361 .
- Chandrasekaran , S and Yoshida , T . 1973 . Effects of organic acid transformations in submerged soils on growth of the rice plant . Soil Sci. Plant Nutr , 19 : 39 – 45 .
- Cheshire , MV and Chapman , SJ . 1996 . Influence of N and P status of plant material and of added N and P on the mineralization of C from 14C-labelled ryegrass in soil . Biol. Fertil. Soils , 21 : 166 – 170 .
- Chidthaisong , A , Inubushi , K , Muramatsu , Y and Watanabe , I . 1996a . Production and emission of methane in flooded rice soil microcosms after continuous application of straws . Microbes Environ , 11 ( 3 ) : 73 – 78 .
- Chidthaisong , A , Inubushi , K and Watanabe , I . 1996b . Methanogenic characteristics of flooded rice soils in response to glucose amendment . Soil Sci. Plant Nutr , 42 ( 3 ) : 645 – 649 .
- Devevre , OC and Horwath , WR . 2000 . Decomposition of rice straw and microbial carbon use efficiency under different soil temperatures and moistures . Soil Biol. Biochem , 32 : 1773 – 1785 .
- Ding , WX , Cai , ZC , Tsuruta , H and Li , XP . 2002 . Effect of standing water depth on methane emissions from freshwater marshes in northeast China . Atmospheric Environ , 36 : 5149 – 5157 .
- Dubois , M , Gilles , KA , Hamilton , JK , Rebers , PA and Smith , F . 1956 . Colorimetric method for determination of sugars and related substances . Anal. Chem , 28 : 350 – 356 .
- Fox , TR . 1995 . “ The influence of low-molecular-weight organic acids on properties and processes in forest soils ” . In Carbon Forms and Functions in Forest Soils , Edited by: McFee , WW and Kelly , JM . 43 – 62 . Madison : Soil Science Society of America .
- Gotoh , S and Onikura , Y . 1971 . Organic acids in a flooded soil receiving added rice straw and their effect on the growth of rice . Soil Sci. Plant Nutr , 17 : 1 – 8 .
- Gottschalk , G . 1986 . “ Bacterial Fermentations ” . In Bacterial Metabolism , 2nd edn , Edited by: Gottschalk , G . 208 – 282 . New York : Springer-Verlag .
- Inubushi , K , Wada , H and Takai , Y . 1984 . Easily decomposable organic matter in paddy soil IV. Relationship between reduction process and organic matter decomposition . Soil Sci. Plant Nutr , 30 ( 2 ) : 189 – 198 .
- Jones , DL . 1998 . Organic acids in the rhizosphere – a critical review . Plant Soil , 205 : 25 – 44 .
- Jones , DL and Darrah , PR . 1994 . Role of root derived organic acids in the mobilization of nutrients from the rhizosphere . Plant Soil , 166 : 247 – 257 .
- Jones , DL , Dennis , PG , Owen , AG and van Hees , PAW . 2003 . Organic acid behavior in soils – misconceptions and knowledge gaps . Plant Soil , 248 : 31 – 41 .
- Krylova , NI , Janssen , PH and Conrad , R . 1997 . Turnover of propionate in methanogenic paddy soil . FEMS Microbiol. Ecol , 23 : 107 – 117 .
- Lynch , JM , Hall , KC , Anderson , HA and Hepburn , A . 1980 . Organic acid from the anaerobic decomposition of Agropyron repens rhizomes . Phytochemistry , 19 : 1846 – 1847 .
- Oades , JM . 1988 . The retention of organic matter in soils . Biogeochemistry , 5 : 35 – 80 .
- Paul , EA and Clark , FE . 1996 . Soil Microbiology and Biochemistry , San Diego : Academic Press .
- Rao , DN and Mikkelsen , DS . 1977a . Effect of acetic, propionic and butyric acids on young rice seedlings growth . Agronomy J , 69 : 923 – 928 .
- Rao , DN and Mikkelsen , DS . 1977b . Effect of rice straw additions on production of organic acids in a flooded soil . Plant Soil , 47 : 303 – 311 .
- de Sousa , RO , Peralba , MCR and Meurer , EJ . 2002 . Short chain organic acid dynamics in solution of flooded soil treated with ryegrass residues . Commun. Soil Sci. Plant Anal , 33 : 779 – 787 .
- Strobel , BW . 2001 . Influence of vegetation on low-molecular-weight carboxylic acids in soil solution – a review . Geoderma , 99 : 169 – 198 .
- Takijima , Y . 1965 . Status of roots in the rhizosphere and the occurrence of root damage . Soil Sci. Plant Nutr , 11 : 204 – 211 .
- Tanaka , A and Navasero , SA . 1967 . CO2and organic acids in relation to growth of rice . Soil Sci. Plant Nutr , 13 : 25 – 30 .
- Tanaka , F and Nishida , M . 1996 . Inhibition of nitrogen uptake by rice after wheat straw application determined by tracer NH+4-15N . Soil Sci. Plant Nutr , 42 ( 3 ) : 587 – 591 .
- Tanaka , F and Nishida , M . 1998 . Inhibitory effects of aromatic acids on nitrogen uptake and transport in rice (Oryza Sativa L.) plants cultured on hydroponics . Soil Sci. Plant Nutr , 44 ( 4 ) : 691 – 694 .
- Tsutsuki , K . 1984 . “ Volatile products and low molecular weight phenolic products of the anaerobic decomposition of organic matter ” . In Organic Matter and Rice , Edited by: Ponnamperuma , FN and Banta , S . 329 – 343 . Los Banos : International Rice Research Institute .
- Wakimoto , K and Seino , K . 1983 . Methods of controlling initial growth inhibition of rice plant caused by wheat straw application to paddy soil . Bull. Kyushu Natl Agric. Exp. Stn , 22 ( 4 ) : 569 – 589 .
- Wang , TS , Cheng , SY and Tung , H . 1967 . Dynamics of soil organic acids . Soil Sci , 104 : 138 – 144 .
- Yamane , I and Sato , K . 1967 . Effect of temperature on the decomposition of organic substances in flooded soil . Soil Sci. Plant Nutr , 13 ( 4 ) : 94 – 100 .