Abstract
The effects of soil management on seasonal changes in soil microbial communities were examined in a continuously cropped field of cabbage (Brassica oleracea var. capitata) using phospholipid fatty acid (PLFA) analysis. Soil samples from each plot treated with cattle manure compost (CMC), grass compost (GRC) and chemical fertilizers (CHF) were taken at monthly intervals from April 2003 to March 2004. The results showed that microbial biomass was smaller in the CHF-treated plot than in the other plots. The mole-percentage contributions of biomarkers for Gram-negative bacteria slightly decreased from April to September and increased from September to March in all plots. The mole-percentage contributions of biomarkers for fungi and actinomyces were greater in the CHF-treated plot compared with the other plots. The mole-percentage contributions of biomarkers for Gram-positive bacteria increased in the order of CMC > GRC > CHF. Higher ratios of cyclopropyl fatty acids to their precursors and lower ratios of monounsaturated fatty acids to saturated fatty acids suggested greater stress to bacteria in the CHF-treated plot than in the other plots. Principal component analysis of the PLFA profiles indicated that soil microbial communities exhibited similar shifts in response to seasonal changes irrespective of the treatment.
INTRODUCTION
The influence of soil microorganisms on ecosystem processes, such as organic matter decomposition and nutrient cycling, nitrogen fixation, aggregate formation and stabilization, and the promotion and suppression of soil-borne disease, is very important to agriculture (CitationAlabouvette et al. 1996; CitationAlexander 1977). However, the utilization of microbial community structure as an indicator of soil quality is still limited. We believe that it can be very important in the evaluation of organically managed agricultural systems, such as organic farming, natural farming or alternative agriculture (e.g. CitationElliott et al. 1996; CitationMäder et al. 2002).
The size and composition of microbial populations have been used to assess the impacts of soil management practices on the biological properties of the soil. Total populations of microorganisms or populations of specific groups have been used in a number of studies for this purpose (e.g. CitationKale and Raghu 1989; CitationMaltby 1975; CitationNishio and Kusano 1980; CitationSuzuki et al. 1969).
Much of this research was done using in vitro culture methods because characterization of soil microbial communities using culture-independent approaches have not yet been developed. Molecular methods, such as analyses of microbial DNA (CitationBorneman et al. 1996) and phospholipid fatty acid (PLFA) analysis (CitationVestal and White 1989; CitationZelles 1999), have been widely used to monitor changes in soil microbial community structure resulting from different management practices on agricultural soil, floodwater of paddy fields or decomposition of organic matter (e.g. CitationHoshino and Matsumoto 2007; CitationKimura et al. 2001; CitationOkabe et al. 2000a,Citationb). Among them, PLFA analysis can provide detailed information about the structure of active microbial communities, including viable but non-culturable microorganisms. The PLFA profiles were examined in farming systems that received different amounts of organic inputs (CitationBossio and Scow 1998; CitationBossio et al. 1998; CitationOkabe et al. 2000a; CitationPeacock et al. 2001; CitationSchutter et al. 2001). These researchers observed changes in PLFA composition following different management practices over a winter season, a spring season or a cropping season. However, these observations were limited to periods of only 1–6 months and in general there are not enough reports detailing the response of soil microbial communities to seasonal variation over a year.
The aim of our investigation was to monitor changes in the community structures of different groups of soil microbial populations using PLFA analysis across a seasonal gradient in plots continuously cropped with cabbage (Brassica oleracea var. capitata) and amended with two types of compost or chemical fertilizer.
MATERIALS AND METHODS
Study site and experimental design
The experimental field was located in the Ohito Research Farm (Izu-no-kuni, Shizuoka, Japan) at latitude 35°3′Ν, longitude 139°1′Ε. The soil of the field was Light Colored Andosol with the following properties at the start of this field experiment in 1996: total C content 41.0 g kg−1; total N content 3.5 g kg−1; available P2O5 content 0.041 g kg−1; P absorption 24.7 g kg−1; pH(H2O) 6.1. The field had been subjected to a continuous fertilizer experiment since 1996 and the fertilizers used were chemical fertilizer (CHF), cattle manure compost (CMC) and grass compost (GRC). Three replicate plots measuring 4 m × 7 m were used for each treatment. The experimental design is shown in . The CMC was made from cattle manure over a 12-month fermentation. The GRC was made from grass and fallen leaves (1:1) and fermented for 6 months. In the GRC-treated plot, organically fertilized soil, which was made from soil, rice husk, rice bran and rapeseed meal (volume rate: 1.5, 0.6, 0.6 and 0.24 m3) over a 9-month fermentation, was applied to achieve the same level of nitrogen among the three treatments. In the CMC-treated and GRC-treated plots, cattle manure compost and grass compost were applied, respectively, at a rate of 30 Mg ha−1 year−1 until 1999 because of a consideration of low nitrogen mineralization of compost. The amount was decreased to 20 Mg ha−1 year−1 from 2000 onwards to obtain a corresponding nitrogen rate of 382–384 kg N ha−1 for all treatments. Chemical fertilizers were applied to the plots 2 weeks prior to transplanting of cabbage and compost was applied 1 month prior to transplanting. Cabbage cultivars “Kinkei201” and “Kosui” were cultivated in spring and autumn, respectively. The average yields of cabbage in 1996–2003 were 25,500 kg ha−1, 24,400 kg ha−1 and 25,300 kg ha−1 in CHF, CMC and GRC, respectively.
Soil sampling
Soil was sampled from the plots once per month from 2 April 2003 to 3 March 2004 in duplicate. Five surface (at a depth of 5–15 cm) soil samples were collected from locations halfway between the cabbage plants and the edge of the raised cabbage beds of each block, approximately 30 cm from the cabbage plant. After mixing, soils were sieved through a 2-mm sieve and stored at –30°C until community analyses were carried out. Some chemical properties of the soils of the plots determined in August 2002 are shown in .
Analysis of the PLFA
The PLFAs were analyzed in duplicate using 10 g (wet weight) of soil according to the one-phase extraction method of CitationBligh and Dyer (1958) and CitationArao et al. (1998) as modified by CitationKato et al. (2005). In the present study, L-α-lecithin-dihenicosanoyl (21:0) was used as an internal standard because 21:0 does not obstruct the detection of other PLFAs in the gas chromatograph (GC) analysis. The fatty acid methyl esters (FAMEs) of the phospholipids were identified and quantified with a flame ionization detector (FID)-GC (Hewlett Packard 6890 series; Hewlett Packard, Wilmington, DE, USA) and MIDI system developed by Microbial I. D., Newark, DE, USA (Sherlock Microbial Identification System 1996). The system consisted of a FID-GC (Hewlett Packard 6890), HP-IB communications, HP3365 ChemStation software and computer (Hewlett Packard). The HP3365 ChemStation software operates the sampling, analysis and integration of the chromatograms. The amount of FAMEs was quantified by analysis of the chromatographic data using the MIDI system and internal standard data of the chromatography.
Table 1 Experimental design
Table 2 Some chemical properties of the soils in each plot
Nomenclature of the fatty acids
Fatty acid was designated based on the total number of C atoms: number of double bonds followed by the position of the double bond from the aliphatic end (ω) of a molecule. Cis and trans configurations are indicated by c and t, respectively. The prefixes “i” and “a” indicate iso- and anteiso-branching, respectively. The fatty acids with a hydroxyl group and a methyl group are respectively presented as OH and Me, with the preceding number indicating their position from the carboxyl end of a molecule. The cyclopropyl fatty acid is designated as “cyc”.
Statistical analysis
Principal component analysis (PCA) and cluster analysis were completed using the mole percents of individual FAMEs. The PCA was carried out with the covariance matrix using the statistical software EXCEL STATISTICS 2000 for Windows (SSRI, Tokyo, Japan). Cluster analysis was carried out using normalized data by the statistical program Kyplot for Windows, version 3 (Kyence, Tokyo, Japan). The Ward method was used for cluster analysis.
RESULTS AND DISCUSSION
Amount of phospholipid fatty acids
The amounts of PLFAs ranged from 34.1 to 60.4 (48.7 ± 7.6, mean ± standard deviation [SD]) µg g−1 dry soil for the CHF plot, from 48.4 to 101.7 (70.9 ± 14.9) µg g−1 dry soil for the CMC plot, and from 56.7 to 100.7 (78.6 ± 15.0) µg g−1 dry soil for the GRC plot (). Total amounts of PLFAs and total carbon were clearly smaller in the CHF-treated plot than in CMC-treated and GC-treated plots (). Season, time of treatment imposition by application of fertilizer or compost and the growth of cabbage had no consistent effect on the amount of PLFAs observed. As the amounts of PLFAs are known to correlate to viable microbial biomass (CitationArao et al. 1998; CitationZelles et al. 1995), it was observed that microbial biomass was smaller in the CHF-treated plot than in those treated with CMC and GRC. Many studies have reported that the application of compost increased the amount of PLFAs (CitationArao et al. 1998; CitationPeacock et al. 2001).
Figure 1 Content of phospholipid fatty acids in the soil of cattle manure compost (CMC, ▀), grass compost (GRC, ▵) and chemical fertilizer (CHF, ○) plots. Error bars indicate the standard deviation. The cultivation managements are shown as follows: arrow, application of fertilizer (closed) or compost (open); •, transplanting; □, harvesting.
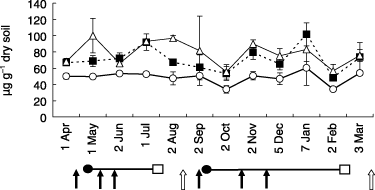
Seasonal variation in PLFA patterns
The PLFAs are classified into five groups as follows: (1) straight, saturated PLFAs, (2) straight, monounsaturated PLFAs (biomarker of Gram-negative bacteria [CitationWilkinson 1988]), (3) straight, polyunsaturated PLFAs including 18:2ω6,9c (biomarker of eukaryotes including fungi [CitationZelles 1999]), (4) iso- and anteiso-branched, saturated PLFAs (biomarker of Gram-positive bacteria [CitationHaack et al. 1994]), (5) 10-methyl, saturated PLFAs (biomarker of actinomycetes [CitationKroppenstedt 1992]).
shows the mole-percentage composition of PLFAs in the CHF, CMC and GRC plots throughout the year as summarized for four periods. The summary of monthly data in the four periods was on the basis of the PCA and cluster analysis described later and made it easy to understand the change in the PFLA patterns in the four seasons: spring, summer, autumn and winter. The major PLFAs in the soil of the cabbage field were 16:0, 18:0, 16:1ω7c, 18:1ω7c, 18:1ω9c, i15:0, a15:0 and 16:0 10Me, especially 16:0, 16:0 10Me and i15:0.
As shown in and , the mole-percentage of straight, monounsaturated PLFAs ranged from 22.5 to 28.8 mol%. It decreased from April to September and increased from September to March, and there were no differences in the proportion of the PLFAs between the CHF, CMC and GRC plots. The mole-percentage contributions of 10-methyl and 18:2ω6,9c PLFAs were greater in the CHF plots (from 10.5 to 12.6 mol%, and from 1.4 to 2.2 mol%, respectively) compared with the CMC (from 8.9 to 10.4 mol%, and from 1.0 to 1.5 mol%, respectively) and the GRC (from 9.3 to 11.4 mol%, and from 0.8 to 1.8 mol%, respectively) plots. The mole-percentage contributions of iso- and anteiso-branched PLFAs became greater in the order CMC > GRC > CHF. However, there were no clear tendencies in seasonal variation with the mole-percentage of 18:2ω6,9c PLFAs, iso- and anteiso-branched PLFAs, and 10-methyl PLFAs.
Table 3 Percentage distribution of phospholipid fatty acids (PLFAs) in the soil
From these results, it was noted that the ratio of Gram-negative bacteria was higher in winter and lower in summer, and the ratios of actinomycetes and fungi were lower in CMC and GRC than in CHF. The relative proportion of Gram-positive bacteria was greater in CMC and GRC than in CHF throughout the year.
Several studies of soil PLFAs have documented an increase in monounsaturated PLFAs with increases in available organic substrates, and increases in the mole-percentage of iso- and anteiso-branched PLFAs, and 10-methyl PLFAs with an increase in inorganic fertilizers (CitationBååth et al. 1995; CitationBossio and Scow 1998; CitationPeacock et al. 2001). Our results do not agree with these reports except with regard to 10-methyl PLFAs. Other studies with increasing levels of organic inputs have yielded results that are similar to our results on iso- and anteiso-branched PLFAs (CitationArao et al. 1998). CitationKato et al. (2005) reported that the proportion of branched PLFAs gradually increased as the composting process progressed and could be used as a tool for evaluating the maturity of manure compost. These differences in the results may result from differences in the soil type, the type of carbon source or the maturity of the manure.
Statistical analysis of the PLFA composition
The PCA of the PLFA composition showed that the contribution percentages of the first two principal components (PC1 and PC2) were 45.5% and 30.0%, respectively (). The PLFAs with highly positive loadings to PC1 were 19:1ω6c, 2OH 16:1, 16:0, 16:1ω9c and 10Me 17:0. The PLFAs with highly negative loadings to PC1 were i15:1ω9, a15:0, 16:1ω11c, i14:0, 16:1ω5c, 16:1ω7c alcohol and i15:0. Among these PLFAs, 16:0, a15:0 and i15:0 were major components of straight, saturated PLFAs, and iso- and anteiso-branched PLFAs, respectively, the percentage contributions of 16:0 occupying 57–65 mol% of straight chain, saturated PLFAs. The contributions of a15:0, i14:0 and i15:0 occupied 57–66 mol% of the iso- and anteiso-branched PLFAs. The other PLFAs with highly positive and negative loadings to PC1 were minor elements of each group of PLFAs. The PLFAs with highly negative loadings to PC1 contained major iso- and anteiso-branched PLFAs, a biomarker of Gram-positive bacteria. Based on these observations, it was suggested that plotting on the left side in means an increased ratio of Gram-positive bacteria.
Figure 2 Seasonal changes in the proportion of biomarker phospholipid fatty acids (PLFAs) in the soil of cattle manure compost (CMC, ▀), grass compost (GRC, ▵) and chemical fertilizer (CHF, ○) plots. Error bars indicate the standard deviation. The cultivation managements are shown as follows: arrow, application of fertilizer (closed) or compost (open); •, transplanting; □, harvesting.
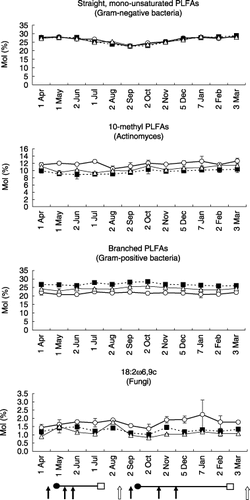
The PLFAs with highly positive loadings to PC2 were 16:1ω7c, 10Me 16:0, 18:1ω7c and a15:1. The PLFAs with highly negative loadings to PC2 were i17:0, 15:0, i16:0 and 18:1ω5c. Among these PLFAs, 16:1ω7c, 18:1ω7c and 10Me 16:0 were major components in straight, monounsaturated PLFAs and 10-methyl PLFAs, respectively. These values suggest that the contributions of 16:1ω7c and 18:1ω7c were 49–59 mol% of straight, monounsaturated PLFAs. The contributions of 10Me 16:0 were 78–85 mol% of 10-methyl PLFAs. The other PLFAs with highly positive and negative loadings to PC2 were minor components of each group of PLFAs. The PLFAs with highly positive loadings to PC2 contained major straight, monounsaturated PLFAs and 10-methyl PLFAs, which are biomarkers of Gram-negative bacteria and actinomycetes, respectively. From these, it was inferred that plotting on the upper side of means an increased ratio of Gram-negative bacteria and actinomycetes.
The PLFAs of the soil collected from the CHF plot were distributed mainly on the right-upward side in , and those from the CMC plot and GRC plot on the left side in , indicating the relative predominance of Gram-positive bacteria in the CMC plot or GRC plot compared with the CHF plot. The PLFAs of the soil were shifted upward from December to June, and shifted downward from August to October in every plot as shown in , indicating the relative predominance of Gram-negative bacteria and actinomycetes in the winter and spring seasons regardless of the soil treatments. These results agreed well with the results shown in and . The relative abundances of 10Me 16:0 and 10Me 17:0 were consistently higher if the plot was treated with CHF than if the plot was treated with the organic composts (). Similar results have been obtained without organic matter input compared with the results obtained when organic matter was added (CitationBossio and Scow 1998; CitationBossio et al. 1998).
16:1ω7c alcohol, one component with highly negative loadings to PC1, was a long-chain alcohol with one double bond, and was detected as a constituent of the FAMEs layers of soil phospholipids in the GC analysis (, ). The genus Bacillus and its relatives exhibits a profile that is characterized by a high proportion of branched-chain PLFAs and the presence of 16:1ω7c alcohol and 16:1ω11c (CitationAmoozegar et al. 2003; CitationHaack et al. 1994; CitationHeyrman et al. 2004, Citation2005; CitationRoberts et al. 1996). The percentage contribution of both 16:1ω7c alcohol and 16:1ω11c was greater in the CMC and GRC-treated plots compared with the CHF-treated plot, and it increased in autumn and winter (). It appears that the percentage contribution of the genus Bacillus and its relatives was greater in the CMC-treated and GRC-treated plots compared with the CHF-treated plots. However, it is necessary to confirm the validity and origin of this biomarker further.
Figure 3 Principal component analysis of the phospholipid fatty acids composition in the soil of cattle manure compost (CMC, ▀), grass compost (GRC, ▵) and chemical fertilizer (CHF, ○) plots.
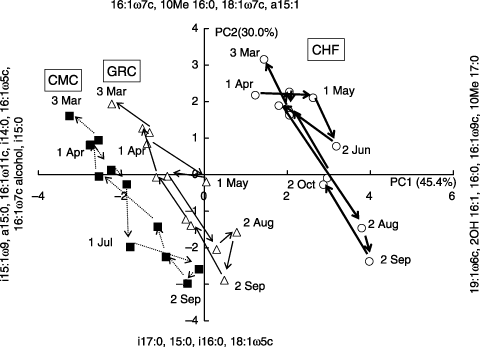
Figure 4 Cluster analysis of the phospholipid fatty acids composition in the soil of cattle manure compost (CMC), grass compost (GRC) and chemical fertilizer (CHF) plots. The Ward method was adopted for the cluster analysis.
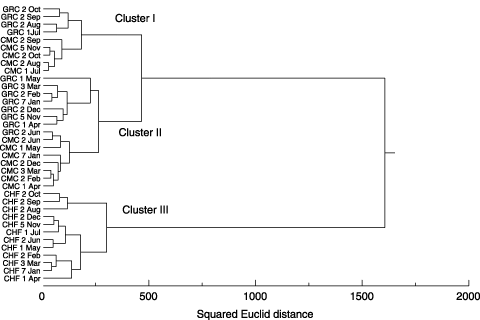
Cluster analysis of the composition of PLFAs revealed that the PLFAs in the soil were divided into three large clusters (): (1) in the summer and autumn seasons (from July to October/November) in the CMC-treated and GRC-treated plots (cluster I), (2) winter and spring seasons (from November/December to June) in the CMC and GRC plots (cluster II), (3) the CHF plots (cluster III). Furthermore, clusters I and II are subdivided into the GRC-treated plot and the CMC-treated plot, respectively, except for the sample taken from GRC on 2 June. The third cluster was further subdivided into (3-1) summer and autumn seasons (from August to October) and (3-2) winter and spring seasons (from November to July) in the CHF plots.
These results of the statistical analysis of the PLFA composition indicate that different microbial communities were established in the CHF CMC and GRC plots, but there were common seasonal successions or dynamics in the microbial community in the plots. Although there have been some reports on the seasonal effects on PLFA profiles in farming systems treated with different amounts of organic inputs, the sampling period in these reports was limited only to the cropping period or to a few sampling intervals, and these reports did not included results or discussions related to the PLFA biomarker (CitationBossio et al. 1998; CitationSchutter et al. 2001).
Stress factors
The ratio of cyclopropyl fatty acids to their precursor 16:1 and 18:1 fatty acids increased under conditions of low carbon, high acidity, low O2 and high temperature, and this was interpreted as a stress marker (CitationKaur et al. 2005; CitationKnivett and Cullen 1965; CitationThomas and Batt 1969). The ratio of monounsaturated PLFAs/straight, saturated PLFAs (monounsat./sat.) fatty acids has been shown to be related to the nutrient availability of the microorganisms. In pure culture studies, the ratio of monounsaturated to saturated PLFAs decreased, and the ratio of cyclopropyl to monoenoic precursor fatty acids increased in Gram-negative bacteria subjected to starvation (CitationKief et al. 1997).
Two PLFA ratios, 19:0cyc/18ω7c and monounsat/sat., are shown in . The percentage contributions of 19:0cyc/18ω7c were greater in the CHF plots than in the CMC and GRC plots, and these ratios increased in summer and decreased in winter. The percentage contributions of monounsat/sat. were lower in the CHF plots than in the CMC and GRC plots except for 1 July, and these ratios decreased in summer and increased in winter.
The results suggested that there was greater stress to bacteria in the chemical fertilizer plot than in the CMC and GRC plots, and the stress increased in summer and decreased in winter. Similar results have been found with increasing organic input in a tomato field with a 4-year rotation (CitationLundquist et al. 1999) and in a rice farming system (CitationBossio and Scow 1998). The stress factors for bacteria might be high temperature in summer, low nutrient supply from low levels of organic matter and higher acidity in the soil of the CHF plot. In the future, we should confirm the validity of the stress indicators using other methods or by more detailed analysis.
The results of our study indicated that there were similar seasonal successions or dynamics in microbial communities in all plots, the proportion of Gram-negative bacteria and actinomycetes increased during winter and spring and decreased during summer and autumn. It is reasonable to assume that the main reason for the seasonal changes in the microbial communities is soil temperature because the patterns of seasonal changes in the biomarker for Gram-negative bacteria and actinomycetes were inversely related to the patterns of temperature changes. There was no obvious response to the cultivation management calendar, for example, the effect of the timing of compost application and the growth of cabbage on the total amount of PLFAs or the mole-percentage contributions of any type of PLFA. In this study, the same fertilizer or compost was continuously applied over 12 times for 6 years in each plot and non-rhizosphere soil was used. These factors may be the reason why no clear trends were observed between the amount or mole-percent of PLFAs and the cultivation management calendar.
Figure 5 19:0cyc/18ω7c ratio and monounsaturated phospholipid fatty acids (PLFAs)/saturated PLFAs ratio in the soil of cattle manure compost (CMC, ▀), grass compost (GRC, ▵) and chemical fertilizer (CHF, ○) plots. Error bars indicate the standard deviation. Monounsaturated PLFAs: 16:1ω5c, 16:1ω7c, 16:1ω11c, 17:1ω9c and 18:1ω7c. Saturated PLFAs: 15:0, 16:0, 17:0, 18:0 and 20:0 (Bossio and Scow 1998). The cultivation managements are shown as follows: arrow, application of fertilizer (closed) or compost (open); •, transplanting; □, harvesting.
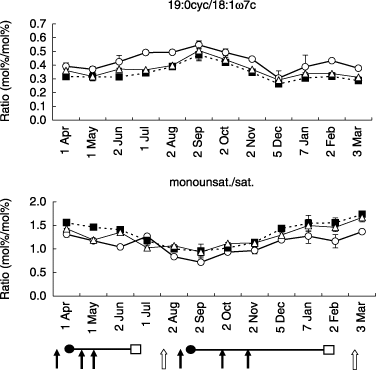
In conclusion, the application of cattle manure compost or grass compost over a 6-year period to a field continuously planted with cabbage resulted in significant increases in C, N and soil microbial biomass, as well as to changes in the microbial community structure. The use of PLFA analysis, coupled with multivariate statistical analysis, allows for better characterization of the changes that occur in response to different soil management practices. The results of this study also indicated that different microbial communities were established in plots that received different treatments, and there were common seasonal successions or dynamics in the microbial communities in any plot. Several studies of soil PLFAs have documented an increase in the ratio of Gram-negative bacteria with increases in the availability of organic substrates. Our contrasting results may have resulted from differences in soil type, the type of carbon source and the maturity of the manure. Therefore, further studies focusing on these aspects should be carried out.
ACKNOWLEDGMENTS
We thank Dr Tomohito Arao, National Institute of Agro-Environmental Sciences, for his technical advice on the measurement of PLFAs, and Mr Naruhiko Sugitani and Mr Kazuhiro Kikegawa, and Mrs Sanae Yunoki, Institute for Agro-microbiology, for analysis of the soil chemical properties and for assistance with the PLFA analysis, respectively. We are grateful to Professor Mitiku D. Habte, University of Hawaii, for his suggestions for improving the manuscript.
REFERENCES
- Alabouvette , C , Hoeper , H , Lemanceau , P and Steinberg , C . 1996 . “ Soil suppressiveness to diseases induced by soilborne plant pathogens ” . In Soil Biochemistry , Edited by: Stotzky , G and Bollag , JM . vol. 9 , 371 – 413 . New York : Marcel Dekker .
- Alexander , M . 1977 . Introduction to Soil Microbiology , New York : John Wiley .
- Amoozegar , MA , Malekzadeh , F , Malik , KA , Schumann , P and Spröer , C . 2003 . Halobacillus karajensis sp. nov., a novel moderate halophile . Int. J. Syst. Evol. Microbiol , 53 : 1059 – 1063 .
- Arao , T , Okano , S and Kanamori , T . 1998 . Analysis of the phospholipid fatty acids of upland Light Colored Andosol and the relationship among the size of biomass based on phospholipid fatty acid analyses, microscopical counts and chloroform fumigation-incubation . Jpn. J. Soil Sci. Plant Nutr , 69 : 38 – 46 . (in Japanese with English summary)
- Bååth , E , Frostegård , A , Pennanen , T and Fritze , H . 1995 . Microbial community structure and pH response in relation to soil organic matter quality in wood-ash fertilized, clear-cut or burned coniferous forest soils . Soil Biol. Biochem , 2 : 229 – 240 .
- Bligh , EG and Dyer , WJ . 1958 . A rapid method of total lipid extraction and purification . Can. J. Biochem. Physiol , 37 : 911 – 917 .
- Borneman , J , Skroch , PW and O'Sullivan , KM . 1996 . Molecular microbial diversity of an agricultural soil in Wisconsin . Appl. Environ. Microbiol , 62 : 1935 – 1943 .
- Bossio , DA and Scow , KM . 1998 . Impacts of carbon and flooding on soil microbial communities: phosopholipid fatty acid profiles and substrate utilization patterns . Microbial. Ecol , 35 : 265 – 278 .
- Bossio , DA , Scow , KM , Gunapala , N and Graham , KJ . 1998 . Determinants of soil microbial communities: effects of agricultural management, season, and soil type on phospholipid fatty acid profiles . Microbial. Ecol , 36 : 1 – 12 .
- Elliott , LF , Lynch , JM and Papendick , RI . 1996 . “ The microbial component of soil quality ” . In Soil Biochemistry , Edited by: Stotzky , G and Bollag , JM . vol. 9. , 1 – 21 . New York : Marcel Dekker .
- Haack , SK , Garchow , H , Odelson , DA , Forney , LJ and Klug , MJ . 1994 . Accuracy, reproducibility, and interpretation of fatty acid methyl ester profiles of model bacterial communities . Appl. Environ. Microbiol , 60 : 2483 – 2493 .
- Heyrman , J , Rodríguez-Díaz , M , Devos , J , Felske , A , Logan , NA and Vos , PD . 2005 . Bacillus arenosi sp. nov., Bacillus arvi sp. nov. and Bacillus humi sp. nov., isolated from soil . Int. J. Syst. Evol. Microbiol , 55 : 111 – 117 .
- Heyrman , J , Vanparys , B Logan , NA . 2004 . Bacillus novalis sp. nov., Bacillus vireti sp. nov., Bacillus soli sp. nov., Bacillus bataviensis sp. nov. and Bacillus drentensis sp. nov., from the Drentse A grasslands . Int. J. Syst. Evol. Microbiol , 54 : 47 – 57 .
- Hoshino , YT and Matsumoto , N . 2007 . Changes in fungal community structure in bulk soil and spinach rhizosphere soil after chemical fumigation as revealed by 18S rDNA PCR-DGGE . Soil Sci. Plant Nutr , 53 : 40 – 55 .
- Kale , SP and Raghu , K . 1989 . Relationship between microbial numbers and other microbial indices in soil . Bull. Environ. Contamin. Toxicol , 43 : 941 – 945 .
- Kato , K , Miura , N , Tabuchi , H and Nioh , I . 2005 . Evaluation of maturity of poultry manure compost by phospholipid fatty acids analysis . Biol. Fertil. Soils , 41 : 399 – 410 .
- Kaur , A , Choudhary , A , Kaur , A , Choudhary , R and Kaushik , R . 2005 . Phospholipid fatty acid—A bioindicator of environment monitoring and assessment in soil ecosystem . Curr. Sci , 89 : 1103 – 1112 .
- Kief , TL , Wilch , E , O’Connor , K , Ringelberg , DB and White , DC . 1997 . Survival and phospholipid fatty acid profiles of surface and subsurface bacteria in natural sediment microcosms . Appl. Environ. Microbiol , 63 : 1531 – 1542 .
- Kimura , M , Miyaki , M , Fujinaka , K and Maie , N . 2001 . Microbiota responsible for the decomposition of rice straw in a submerged paddy soil estimated from phospholipid fatty acid composition . Soil Sci. Plant Nutr , 47 : 569 – 578 .
- Knivett , VA and Cullen , J . 1965 . Some factors affecting cyclopropane acid formation in Escherichia coli . Biochem. J , 96 : 771 – 776 .
- Kroppenstedt , RM . 1992 . “ The genus Nocardiopsis ” . In The Prokaryotes , Edited by: Balows , A , Trüper , HG , Dworkin , M , Harder , W and Schleifer , KH . vol. 2 , 1139 – 1156 . Berlin : Springer .
- Lundquist , EJ , Scow , KM , Jackson , LE , Uesugi , SL and Johnson , CR . 1999 . Rapid response of soil microbial communities from conventional, low input, and organic farming systems to a wet/dry cycle . Soil Biol. Biochem , 31 : 1661 – 1675 .
- Mäder , P , Flieβbach , A , Dubois , D , Gunst , L , Fried , P and Niggli , U . 2002 . Soil fertility and biodiversity in organic farming . Science , 296 : 1694 – 1697 .
- Maltby , E . 1975 . Numbers of soil microorganisms as ecological indicators of changes resulting from moorland reclamation on Exmoor, UK . J. Biogeography , 2 : 117 – 136 .
- Nishio , M and Kusano , S . 1980 . Function patterns of microbial numbers in soil applied with compost . Soil Sci. Plant Nutr , 26 : 581 – 593 .
- Okabe , A , Oike , H , Toyota , K and Kimura , M . 2000a . Seasonal variations of phospholipid fatty acid composition in floodwater of a Japanese paddy field under a long-term fertilizer trial . Soil Sci. Plant Nutr , 46 : 177 – 188 .
- Okabe , A , Toyota , K and Kimura , M . 2000b . Comparison of phospholipid fatty acid composition in floodwater and plow layer soil during the rice cultivation period in a Japanese paddy field . Soil Sci. Plant Nutr , 46 : 893 – 904 .
- Peacock , AD , Mullen , MD , Ringelberg , DB , Tyler , DD , Hedrick , DB , Gale , PM and White , DC . 2001 . Soil microbial community responses to dairy manure or ammonium nitrate applications . Soil Biol. Biochem , 33 : 1011 – 1019 .
- Roberts , MS , Nakamura , LK and Cohan , FM . 1996 . Bacillus vallismortis sp. nov., a close relative of Bacillus subtilis, isolated from soil in Death Valley, California . Int. J. Syst. Bacteriol , 46 : 470 – 475 .
- Schutter , ME , Sandeno , JM and Dick , RP . 2001 . Seasonal, soil type, and alternative management influences on microbial communities of vegetable cropping systems . Biol. Fertil. Soils , 34 : 397 – 410 .
- Suzuki , T , Tokunaga , Y and Watanabe , I . 1969 . Effect of the difference of tillage operations on microbial properties of soil layers . Soil Sci. Plant Nutr , 15 : 280 – 291 .
- Thomas , TD and Batt , RD . 1969 . Degradation of cell constituents by starved Streptococcus lactis in relation to survival . J. Gen. Microbiol , 58 : 347 – 362 .
- Vestal , JR and White , DC . 1989 . Lipid analysis in microbial ecology: quantitative approaches to the study of microbial communities . BioScience , 39 : 535 – 541 .
- Wilkinson , SG . 1988 . “ Gram-negative bacteria ” . In Microbial Lipids , Edited by: Ratledge , C and Wilkinson , SG . vol. 1 , 299 – 488 . London : Academic Press .
- Zelles , L . 1999 . Fatty acid patterns of phospholipids and lipopolysaccharides in the characterization of microbial communities in soil: a review . Biol. Fertil. Soils , 29 : 111 – 129 .
- Zelles , L , Bai , QY , Rackwitz , R , Chadwick , D and Besse , F . 1995 . Determination of phospholipid- and lipopolysaccharide-derived fatty acids as an estimate of microbial biomass and community structures in soils . Biol. Fertil. Soils , 19 : 115 – 123 .