Abstract
The term “co-situs application” refers to a technology that uses controlled-release fertilizer (CRF) attached with the seeds or seedlings as the single application for the entire growing season without causing salt injury. Using a co-situs application technique, a pot experiment was carried out to evaluate the importance of contact between rice roots and co-situs applied fertilizer granules on Fe absorption by paddy rice in a calcareous paddy soil. Oriza sativa cv. Hatajirushi was used as the test plant. The treatments were as follows: (1) co-situs application of CRF containing only NPK (CRFNPK), (2) co-situs application of CRF containing only NPK and broadcast application of micronutrients (CRFNPK + M), (3) co-situs application of CRF containing NPK and micronutrients without a barrier between the roots and the fertilizer (CRFM), (4) co-situs application of the same fertilizer as on the CRFM treatment with a barrier between the roots and the fertilizer (CRFM + barrier). In the final treatment, a barrier was formed by placing the fertilizer into a sealed nylon mesh (30 µm) bag to prevent contact with the rice roots. For all treatments, the soil was kept flooded during the experimental period. Plants in the CRFNPK treatment showed severe chlorosis in the young leaves and all died within 40 days after transplanting (DAT). The barrier decreased the concentrations of Fe, Zn and Mn in the rice leaves by 22.5, 32.7 and 48.5% respectively, relative to plants grown without a barrier (CRFM treatment); the barrier also decreased the total number of grains per hill, number of ripened grains, dry matter yield and grain yield by 34.5, 44.1, 41.5 and 73.2%, respectively. Plants in the CRFNPK + M treatment grew poorly and were shorter, had less tillers and showed yellowish leaf color than those in the CRFM and CRFM + barrier treatments. We conclude that contact between rice roots and fertilizer granules is of considerable importance because the use of a barrier to prevent such contact decreased the concentration of Fe and some other micronutrients in the leaves to levels close to those known to be critical for rice growth, as well as markedly reducing yield.
INTRODUCTION
Iron is one of three nutrients (the others being nitrogen and phosphorus) that most commonly limit plant growth (CitationMarshner 1995). Iron deficiency cannot be remedied through the use of traditional fertilizers because the problem is not one of abundance, but rather one of solubility (CitationGuerinot 2001). Thus, bioengineering of crops to release more Fe-solubilizing chelators has been the principal strategy for improving rice yield in calcareous soils. In this way, CitationTakahashi et al. (2001) engineered a transgenic rice to release increased levels of deoxymugineic acid, an Fe-solubilizing chelator. The transgenic plants exhibited enhanced growth in soils with low Fe availability. In contrast, we succeeded in growing paddy rice in a highly calcareous soil using co-situs application of Fe-containing controlled-release fertilizers (CRFs) (CitationMorikawa et al. 2004, Citation2005). However, the importance of contact between the rice roots and the CRF granules is unclear.
The term “co-situs application” refers to a technology that uses CRF along with the seeds or seedlings as the single application for the entire growing season without causing salt injury. This term was coined by CitationShoji and Gandeza (1992) to differentiate it from the contact placement of small amounts of conventional fertilizers (rapidly soluble fertilizers) to give crops a rapid start (CitationRandall and Hoeft 1988). This method has been studied mainly in relation to nitrogen nutrition. For example, CitationKaneta et al. (1994) reported that wetland rice absorbed approximately 80% of the N applied using co-situs placement, which is threefold more than the recovery of basal N applied using conventional fertilization.
CitationEaton (1936) observed that corn could be grown at a pH value of 9 without chlorosis provided that magnetite was mixed with sand to yield a final percentage of 0.1% in a sand culture experiment. CitationEaton (1936) concluded that corn was able to absorb the insoluble Fe directly from the solid particles. CitationChapman (1939) used the term “contact feeding” for the uptake of Fe by citrus roots from finely ground magnetite in a sand culture setup. Furthermore, CitationWallace (1962) argued that the more effective uptake of Fe when roots were in contact with solid substrate resulted from “contact chelation” and not just to a purely physical contact–exchange phenomenon. In the present study we attempted to evaluate the importance of physical contact between roots and co-situs-applied fertilizer granules on Fe absorption by paddy rice.
MATERIALS AND METHODS
A pot experiment was carried out at Tohoku University to evaluate the importance of the contact between rice roots and co-situs-applied fertilizer granules on Fe absorption by paddy rice in a calcareous paddy soil. Oriza sativa cv. Hatajirushi was used as the test plant. The soil used was collected from a mountainous area of Ishikawa prefecture, Japan. The soil was sieved to pass through a 2-mm mesh before use and, as shown in , it had a low organic matter content and a pH in water of 9.3 (the soil : water ratio was 1:2.5).
The experiment was conducted in pots with a soil capacity of 3 kg. The treatments were as follows: (1) co-situs application of CRF containing only NPK (CRFNPK), (2) co-situs application of CRF containing only NPK and broadcast application of micronutrients (CRFNPK + M), (3) co-situs application of CRF containing NPK and micronutrients without a barrier (CRFM), (4) co-situs application of the same fertilizer as in the CRFM treatment with a barrier (CRFM + barrier). In the final treatment, the barrier was created by placing the fertilizers into a nylon mesh (30 µm) bag to prevent contact with the rice roots. The nylon mesh bags were sealed to prevent the granules of fertilizer coming out. All treatments were made at transplanting time as co-situs application with the rice seedlings. is a schematic representation showing details of the fertilization placement and the separation of the root systems of the rice by the nylon mesh bags or their lack of separation.
Table 1 Selected chemical properties of the soil used
Table 2 Total amounts of nutrients applied in each treatment
The total amounts of nutrients applied in the treatments are shown in . All treatments received similar amounts of NPK and micronutrients. Each CRF was applied locally in contact with the roots of rice seedlings at a rate of 0.54 g N hill−1 (“co-situs application”) at transplanting time. The fertilizers were commercial formulas from Chisso Asahi (Tokyo, Japan). For the CRFNPK + M treatment, the readily soluble forms, FeSO4.7H2O, ZnSO4.H2O, MnCl2, H3BO3 and CuSO4 H2O were used as sources of Fe, Zn, Mn, B and Cu, respectively. The chemical composition of the CRFs was as follows (g kg−1): CRFNPK, total N 140, P2O5 120, K2O 140; CRFM, total N 130, P2O5 110, K2O 130, Mn 1.0, B 0.6, Fe 5.0, Cu 0.7, Zn 0.3. All CRFs used released 80% of the N content during 70 days at 25°C under moist conditions. For all treatments, the soil was kept flooded during the experimental period.
Figure 1 Schematic representation of the location of fertilization and the separation of the rice root systems using nylon mesh. CRFNPK, controlled-release fertilizer containing only NPK; CRFNPK + M, controlled-release fertilizer containing NPK and a broadcast application of micronutrients; CRFM, controlled-release fertilizer containing NPK and micronutrients without a barrier between the roots and the fertilizer; CRFM + barrier, controlled-release fertilizer containing NPK and micronutrients with a barrier between the roots and the fertilizer.
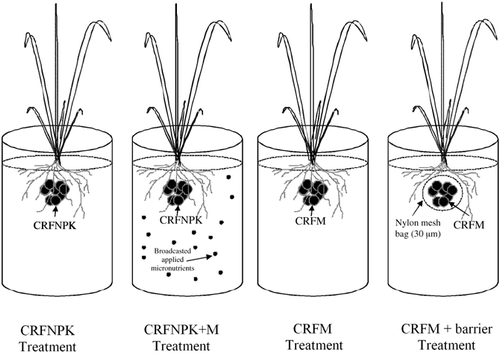
Rice seeds were soaked and incubated for 5–7 days at 20°C; pre-germinated seeds were sown in trays containing nursery soil and rice seedlings were grown for 1 month before being transplanted to the pots. The AB horizon of an Andisol soil with a pH 5.5 was used as the nursery bed, which was removed from the rice seedlings before transplanting by washing. The seedlings were transplanted (three seedlings per hill) when the number of leaves reached 4.2.
Soil Fe was extracted using acid ammonium oxalate (CitationMcKeague 1976), sodium pyrophosphate solution (0.1 mol L−1; pH 10) (CitationMcKeague 1967) and citrate–bicarbonate–dithionite (CitationHolmgreen 1967). The Fe in the extracts was measured using atomic absorption (Hitachi Z-6100; Hitachi, Tokyo, Japan) and the carbon content of the soil samples was measured with a Sumika NC-80S analyzer (Shimadzu, Kyoto, Japan). The organic matter content was calculated by multiplying the amount of organic carbon (%) by 1.724.
Soil pH was determined for a 1:2.5 soil : water suspension using a glass electrode. Ammonium bicarbonate diethylene triamine pentaacetic acid (AB-DTPA)-extractable Fe, Zn, Mn and Cu were analyzed according to the method of CitationSoltampour and Schwab (1977).
After harvesting, the rice plants were washed with distilled water and then dried at 70°C for 48 h in an oven before the dry yields were measured. The dried material was ground and ashed in a muffle furnace at 500°C and the ash was then treated with acid solution on a hot plate according to the method of CitationHowitz (1980). The Fe, Zn and Cu concentrations of the extracts were analyzed using atomic absorption spectrometry.
Data were analyzed by anova in a nested design and the means were separated using a Fisher's least significant difference test at P ≤ 0.05. The growth parameters and yield components were measured using nine and 18 hills in each plot, respectively.
Figure 2 Effect of treatments on plant length, number of tillers and leaf color. CRFNPK, controlled-release fertilizer containing only NPK; CRFNPK + M, controlled-release fertilizer containing NPK and a broadcast application of micronutrients; CRFM, controlled-release fertilizer containing NPK and micronutrients without a barrier between the roots and the fertilizer; CRFM + barrier, controlled-release fertilizer containing NPK and micronutrients with a barrier between the roots and the fertilizer.
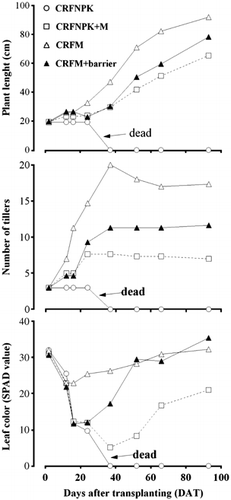
Table 3 Effect of the treatments on the concentrations of Fe, Zn, Cu and Mn in rice leaves at harvest
RESULTS AND DISCUSSION
shows the effect of the treatments on plant height, number of tillers and leaf color, which was measured as a soil and plant analyzer development (SPAD) value. The leaf color of the newest fully expanded leaf was measured using a SPAD meter (Konica Minolta, Tokyo, Japan). Plants in the CRFNPK treatment showed severe chlorosis in the young leaves, which was characteristic of Fe deficiency, and all died within 40 days after transplanting (DAT). Plants in the CRFNPK + M treatment grew poorly and were shorter, had less tillers and showed yellowish leaf color compared with those in the CRFM and CRFM + barrier treatments. The tallest plants and the highest number of tillers were found in the CRFM treatment. Except for the CRFNPK treatment, in the first days after transplanting the SPAD values decreased for all treatments and later recovered. However, treatments differed in the timing at which recovery began. The CRFNPK + M treatment took 40 DAT to recover the SPAD values, whereas the CRFM + barrier and CRFM treatments took 20 and 10 DAT, respectively. It was clear that the application of readily soluble forms of Fe fertilizer was unable to supply the plant demand for Fe over the entire growing season following a single application.
The effect of the treatments on the leaf concentrations of Fe, Zn, Cu and Mn at harvest time is shown in . The Fe, Zn and Mn concentrations in the CRFM treatment were significantly higher (P < 0.05) than those in the CRFM + barrier and CRFNPK + M treatments. No differences between treatments were found for the Cu concentration of rice leaves. The barrier decreased the leaf concentrations of Fe, Zn and Mn by 22.5, 32.7 and 48.5%, respectively, compared with the concentrations in plants grown without a barrier (CRFM treatment).
The Fe concentrations of leaves of plants in the CRF + M treatment were much lower than the 50 µg g−1 critical value for Fe deficiency in rice proposed by CitationDobermann and Fairhurst (2000), showing that Fe availability to rice plants is low if the Fe is applied as readily soluble fertilizers. In contrast, the barrier decreased the Fe concentrations of rice leaves from 75.9 to 58.8 µg g−1 and brought it close to the value considered critical for rice growth. Contact between rice roots and CRF granules appeared, therefore, to affect the absorption of Fe by the rice roots. For all treatments, the concentrations of Zn were lower than the 20 µg g−1 value considered to be critical for Zn deficiency in whole young rice plants by CitationDobermann and Fairhurst (2000). However, except for the CRFNPK treatment in which rice plants died, no visual symptoms of Zn deficiency were found for any of the other treatments. The Cu concentrations in the leaves were much higher than the 6 µg g−1 critical value for Cu deficiency proposed by CitationDobermann and Fairhurst (2000). The proportions of Mn found in the leaf tissues were high enough to be toxic, although no visual symptoms of Mn toxicity were observed during the growth period. It appears, therefore, that the soil used had enough Cu and more than enough Mn for paddy rice growth.
Table 4 Effect of the treatments on yield components
shows the effect of the treatments on yield components. Plants in the CRFM treatment had the highest (LSD0.05) values of number of grains per panicle, total number of grains per hill, ripened grains, dry matter of leaves and stems, and grain yield. Large differences between treatments were found for the percentage of ripened grains, which were reflected in the grain yields. The barrier decreased the total number of grains per hill, percentage of ripened grains, dry matter yield of leaves and stems and grain yield of rice by 34.5, 44.1, 41.5 and 73.2%, respectively, compared with the corresponding values in plants grown without a barrier (CRFM treatment).
The acquisition of water and nutrients is a major function of plant root systems. Plants have developed strategies for enhancing nutrient uptake from nutrient-poor soils. In our experiment, an increased number of fine roots were visually observed in plants growing on calcareous soil compared with plants growing on Andisol. The rice plants grown in calcareous soil probably increased the area of their superficial roots in contact with the fertilizer granules by increasing the number of fine roots. The exposure of plants to sub-optimal Fe availability is associated with several changes in root morphology. The most common and well-documented change is the formation of cluster roots. According to CitationLouis et al. (1990), the formation of cluster roots is favored for P deficiencies in some species such as Myrica cerifera. However, experiments carried out on three Casuarina species (Casuarina glauca, Casuarina cunninghamiana Miq and Casuarina equisetifolia L) indicated that Fe is involved in cluster root formation (CitationZaïd et al. 2003). Thus, it is well known that dicotyledonous plants respond to Fe deficiency by expressing specific physiological and morphological responses in their roots. However, no such relationships are apparent in the literature for gramineous plants. In this study, we microscopically observed fine rice roots in intimate contact with Fe-containing CRF in the CRFM treatment (). This phenomenon can be considered to be an ecophysiological response to a specific nutrient deficiency, enabling plants to enhance nutrient uptake and serving as evidence of the importance of contact between rice roots and nutrient sources for the absorption of nutrients by rice roots. The barrier decreased the dry weight of leaves and stems of rice by 41.5% and the Fe concentration in the leaves decreased by 22.5% compared with equivalent values in plants grown without a barrier (CRFM treatment).
Controlled-release fertilizers that contain Fe and other micronutrients improved production by supplying these nutrients to the crop. This is possible because CRFs deliver Fe to the plant gradually. The timing of nutrient release from the CRF granules is dependent on the soil temperature, the polymer coating thickness of the granules and soil moisture (CitationShoji and Gandeza 1992). Therefore, CRFs can be a more efficient method of fertilizing a crop than conventional fertilizers because more nutrients are taken up by the crop and fewer nutrients are lost in the calcareous soil.
Finally, we conclude that contact between rice roots and CRF granules is important for the supply of Fe to rice plants when the co-situs method of fertilizer application is used in calcareous paddy soils.
REFERENCES
- Chapman , HD . 1939 . Absorption of iron from finely ground magnetite by citrus seedlings . Soil Sci , 48 : 309 – 315 .
- Dobermann , A and Fairhurst , TH . 2000 . Rice: Nutritional Disorders and Nutrient Management , Singapore : International Rice Research Institute and Potash and Phosphate Institute .
- Eaton , FM . 1936 . Automatically operated sand-culture equipment . J. Agr. Res , 53 : 433 – 444 .
- Guerinot , LM . 2001 . Improving rice yields – ironing out the details: engineering crops to release more iron-solubilizing chelators may increase their yield in alkaline soils . Nat. Biotech , 19 : 417 – 418 .
- Holmgreen , GGS . 1967 . A rapid citrate–dithionite extractable iron procedure . Soil Sci. Soc. Am. Proc , 31 : 210 – 211 .
- Howitz , GW . 1980 . Official Methods of Analysis of the Association of Official Analytical Chemists , 13th edn , Washington : Association of Official Analytical Chemists .
- Kaneta , Y , Awasaki , H and Murai , Y . 1994 . The non-tillage rice culture by single application of fertilizer in a nursery box with controlled-release fertilizer . Jpn. Soil Sci. Plant Nutr , 65 : 385 – 391 .
- Louis , I , Racette , S and Torrey , JG . 1990 . Occurrence of cluster roots on Myrica cerifera L. (Myricaceae) in water culture in relation to phosphorus nutrition . New Phytol , 115 : 311 – 317 .
- Marshner , H . 1995 . Mineral Nutrition of Higher Plants , 2nd edn , New York : Academic Press .
- McKeague , JA . 1967 . An evaluation of 0.1 M pyrophosphate and pyrophosphate–dithionite in comparison with oxalate as extractants of the accumulation products in podzols and some other soils . Can. J. Soil Sci , 47 : 95 – 99 .
- McKeague , JA . 1976 . Manual on Soil Sampling and Methods of Analysis , Ottawa : Soil Research Institute, Agriculture Canada .
- Morikawa , CK , Saigusa , M , Nakanishi , H , Nishizawa , NK and Mori , S . 2004 . Co-situs application of controlled-release fertilizers to alleviate iron chlorosis of paddy rice grown in calcareous soil . Soil Sci. Plant Nutr , 50 ( 7) : 1013 – 1021 .
- Morikawa , CK , Saigusa , M , Nakanishi , H , Nishizawa , NK and Mori , S . Successful yield improvement of paddy rice in calcareous soils by new fertilization methodsInPlant Nutrition for Food Security, Human Health and Environmental Protection . Abstract from the Fifteenth International Plant Nutrition Colloquium . September 14–23 , Beijing, China.
- Randall , GW and Hoeft , RG . 1988 . Placement methods for improved efficiency of P and K fertilizers: a review . J. Prod. Agric , 1 : 70 – 79 .
- Shoji , S and Gandeza , AT . 1992 . Controlled-release Fertilizers with Polyolefin Resin Coating , Sendai : Konno Printing .
- Soltampour , PN and Schwab , AP . 1977 . A new soil test for simultaneous extraction of macro and micronutrients in alkaline soils . Comm. Soil Sci. Plant Anal , 8 : 195 – 207 .
- Takahashi , M , Nakanishi , H , Kawasaki , S , Nishizawa , NK and Mori , S . 2001 . Enhanced tolerance of rice to low iron availability in alkaline soils using barley nicotianamine aminotransferase genes . Nat. Biotech , 19 : 466 – 469 .
- Wallace , A . 1962 . “ Metal chelation and mechanism of metal function in biological systems ” . In Decade of Synthetic Chelating Agents in Inorganic Plant Nutrition , Edited by: Walace , A. 3 – 19 . Ann Arbor : Edwards Brothers .
- Zaïd , ElH , Arabou , M , Diem , HG and Morabet , REl . 2003 . Is Fe deficiency rather than P deficiency the cause of cluster root formation in Casuarina species? . Plant Soil , 248 : 229 – 235 .