Abstract
Hydroponically grown barley plants (Hordeum vulgare L. cv. Minorimugi) under iron-deficient (–Fe) and high phosphorus (P) conditions (500 µmol L−1) showed Fe chlorosis and lower growth compared with plants grown in –Fe and low P conditions (50, 5 and 0.5 µmol L−1). To understand the physiological role of P in regulating the growth of plants in –Fe medium, we carried out an Fe feeding experiment using four P levels (500, 50, 5 and 0.5 µmol L−1) and phytosiderophores (PS), mugineic acid. Our results suggest that plants grown in a high P medium had higher absorption activity of 59Fe compared with plants grown in low P media, irrespective of the presence or absence of added PS. Translocation of 59Fe from roots to shoots was not affected by the P level. The relative translocation rate of 59Fe increased with decreasing levels of P in the medium. In general, the addition of PS enhanced the absorption of 59Fe and its translocation. Taken together these results suggest that the lower relative translocation rate of Fe in high P plants may be induced by the physiological inactivation of Fe in the roots, and the higher absorption activity of Fe in high P conditions possibly results from the response of barley plants to Fe deficiency.
INTRODUCTION
Iron (Fe) and phosphorus (P) are two essential mineral elements for plant growth and development and deficiencies in these two elements play a major role in limiting crop yield worldwide. A deficiency in these elements in the soil may result from various reasons. For example, the chemical forms of Fe found in most soils are sparingly soluble in soil solution at biological pH, and in calcareous soils Fe concentrations in soil solution usually range from 0.1 to 10% of the total needed by the plants (CitationLindsay 1984). The formation of water-insoluble Fe oxides and oxyhydroxides in calcareous soils further affects the availability of Fe to plants, even when the total Fe concentration is relatively high (CitationHartwig and Loeppert 1993; CitationLindsay and Schwab 1982) in such soils. A deficiency of Fe in soil ultimately results in Fe chlorosis induced by high pH or carbonate (CitationRömheld and Marschner 1991). Similarly, a deficiency in P, which is the second most limited nutrient for plant growth after nitrogen (CitationVance et al. 2003), results from fixation of P by Fe and Al in acidic conditions and formation of insoluble complexes by calcium in alkaline or calcareous conditions (CitationMengel and Kirkby 2001).
Combined deficiencies of P and other micronutrients may potentially occur in calcareous and alkaline soils. For example, most of the chickpea-growing regions of India are deficient in P as well as other micronutrients (CitationSrinivasarao et al. 2006).
The ratio of Fe to P in plant tissues has been reported as one of the regulators of Fe chlorosis in some plants. Several reports have shown that a high Fe/P ratio reduces the expression of Fe chlorosis (CitationDeKock and Alexander 1955; CitationLadouceur et al. 2006; CitationPushnik et al. 1984). CitationLadouceur et al. (2006) found that the Fe concentration in shoots of plants grown under –Fe and high or low P conditions varied within the range of the critical deficiency level of 30–50 µg g−1 (CitationRömheld and Marschner 1991) or was lower than 65 µg g−1 dry matter (CitationTang et al. 1990). However, plants grown in low P conditions did not show Fe chlorosis (CitationLadouceur et al. 2006). It is known that –Fe symptoms in plants occur because of factors inhibiting Fe absorption and translocation or impairing its utilization in metabolic processes rather than as a result of scarcity in the environment (CitationBrown 1961; CitationWelch et al. 1991). Low P appears to activate Fe for chlorophyll synthesis in plant tissues under Fe-stressed conditions. The physiological mechanism of absence of chlorosis in low P conditions has not been well clarified.
The phytosiderophores (PS) released by graminaceous plants (Strategy II plants) are well known for their Fe acquisition capability in Fe-stressed conditions (CitationRömheld and Marschner 1986; CitationTakagi et al. 1984). CitationTakagi et al. (1984) reported that the addition of PS to the medium increased Fe uptake by rice seedlings, resulting in greening of the chlorotic leaves. High P concentration in –Fe medium enhanced the release and accumulation of PS in roots (CitationLadouceur et al. 2006). Furthermore, CitationAlam et al. (2005) showed that the addition of PS to the media resulted in an increase in 59Fe absorption and translocation from roots to shoots in barley. Taken together these results suggest that PS is an important regulator for Fe absorption and translocation in plants. However, the roles of PS and/or P on Fe absorption and translocation in Strategy II plants under Fe stress remain elusive. To clarify the role of PS in the absorption and translocation of Fe in Fe-stressed and low P conditions, a feeding experiment was conducted using radioactive 59Fe in barley plants in the presence or absence of PS in the growth medium.
MATERIALS AND METHODS
Plant culture and growth
Seedlings of barley plants (Hordeum vulgare L. cv. Minorimugi) were cultivated using a previously described method (CitationLadouceur et al. 2006). Plants were transplanted in bunches of three plants wrapped with sponge rubber and transferred to 10-L plastic buckets (16 bunches per bucket) filled with half-strength Hoagland–Arnon solution. After 2 days of growth in the above nutrient solution, the plants were transferred to –Fe half-strength modified Hoagland–Arnon solution (CitationTakagi 1993) with four P levels, 500 (original concentration in the Hoagland–Arnon solution), 50, 5 and 0.5 µmol L−1 P. Phosphorus was supplied as NaH2PO4. The plants were grown in a phytotron (day/night 14/10 h; temperature 17/10°C; light intensity 280 µmol m−2 s−1). The pH of the nutrient solutions was monitored daily and adjusted to 6.5. The nutrient solutions were renewed weekly. The plants were allowed to grow for 12 days after treatment (DAT) prior to the 59Fe feeding experiment.
Chlorophyll index of the leaves
Chlorophyll content in the fourth leaves was measured in three bunches of plants at harvest using a SPAD-502 chlo-rophyll meter (Minolta Camera Company, Tokyo, Japan).
Source of used phytosiderophores and 59Fe
Mugineic acid (one of the major PS released by the barley cultivar) was collected from the root washings of –Fe barley using a previously described method (CitationTakagi 1993). The 59Fe-radionuclide was purchased from Perkin Elmer Life and Analytical Sciences (Boston, MA, USA).
Feeding experiment with 59Fe
At 12 DAT, plant roots were washed with deionized water and transferred to feeding solutions with four P levels where 59Fe as 59FeCl3 (10 µmol L−1) was added with or without PS (10 µmol L−1), and the plants were fed for 4 h in the afternoon in beakers wrapped with aluminum foil containing 100 mL of feeding solution. Previously it has been shown that PS is not released during 4 h treatments in the afternoon (CitationKawai et al. 1988). The starting time of 59Fe feeding was 8 h after the onset of light in the phytotron. The radioactivity of 59Fe in each beaker was 37 kBq. The apoplastic 59Fe in the roots was solubilized and removed after feeding using the method of CitationBienfait et al. (1985). After that, the plants were thoroughly washed with tap water, divided into shoots and roots, oven-dried at 70°C for 1 day and weighed.
Measurement of 59Fe
Dried shoots and roots of the plants were digested in concentrated nitrate as described by CitationZarcinas et al. (1987). The radioactivity of 59Fe in the digested plant solutions or the root washings containing apoplastic 59Fe was determined using a gamma scintillation counter (Auto Well Gamma System, AccuFLEX ARC-7000, Aloka, Tokyo, Japan). The amount of the extracellular 59Fe in the root apoplast was not included in the 59Fe content in roots. The total absorption represents the sum of the amount of 59Fe in the shoots and roots, and the absorption activity per root dry weight (DW) represents the total amount of 59Fe in the plant divided by the root dry weight. The translocation per plant represents the shoot content of 59Fe, and the translocation per shoot DW was also calculated. The relative translocation rate represents the percentage of the 59Fe translocated from roots to shoot to the total absorption of 59Fe per plant.
Statistical analysis
The experiment was arranged in a completely randomized block design with three replicates. Data were subjected to an anova (CitationSAS Institute 1988). The treatment means were compared by Duncan's Multiple Range Test (P < 0.05) using the computer “Origin 5” at Iwate University.
Table 1 Dry weight and chlorophyll index in barley plants grown in Fe-deficient media with different P levels at 12 days after treatment
Figure 1 (a) Total absorption and (b) root absorption activity of 59Fe in barley plants grown in Fe-deficient media with different P levels, with or without phytosiderophores (PS) (small letters for plants without PS and capital letters for plants with PS). Different letters indicate significant differences (P < 0.05) among P levels. Asterisks indicate significant differences (P < 0.05) between treatments with or without PS at each P level. DW, dry weight.
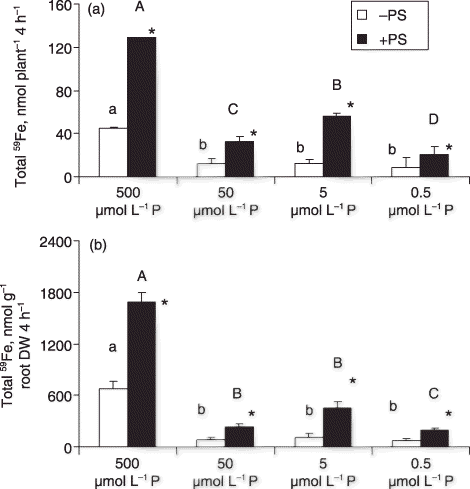
RESULTS AND DISCUSSION
Dry weight and chlorophyll index of the plants
The dry weights of the shoots and roots and the chlorophyll index were higher in plants grown under low P (50, 5 and 0.5 µmol L−1 P) and –Fe conditions compared with those grown in high P (500 µmol L−1 P) medium (). Consistent with a previous report, we found that the leaves of low P grown plants were green, while those of high P grown plants showed Fe chlorosis (CitationLadouceur et al. 2006).
Total absorption and root absorption activity of 59Fe in plants
The total absorption of 59Fe was found to be the highest in high P (500 µmol L−1 P) conditions even in the presence or absence of PS (). In contrast, the total absorption of 59Fe was reduced in low P (50, 5, and 0.5 µmol L−1 P) conditions. Phytosiderophores enhanced the absorption of 59Fe in all conditions. In the –PS condition, the total absorption of 59Fe was similar in plants grown in low P (50, 5, and 0.5 µmol L−1 P) media. The addition of PS to the feeding solution increased the total absorption of 59Fe in plants by 1.3–3.7-fold regardless of the P level of the medium. The absorption activity of 59Fe in plants per gram root DW showed a similar pattern (). It was clear that plants grown in low P showed a lower Fe uptake activity compared with plants grown in high P, despite the larger root system and greener leaves of the former (). We also found that low P inhibits the 59Fe absorption activity of plants irrespective of the presence or absence of PS in the medium. Previously, it has been shown that Fe chlorotic plants with high P concentrations in the shoot and roots had lower Fe content in the shoots than non-chlorotic plants with low P concentrations in the shoot and roots (CitationLadouceur et al. 2006). This higher absorption activity of 59Fe in the high P condition may result from a higher response to Fe deficiency in plants. Plants grown in –Fe and high P medium may express chlorosis and show higher absorption activity of Fe. The activity of the transporter for Fe in the root plasma membrane of plants might be enhanced by –Fe and high P.
The addition of PS to the medium enhanced both the total 59Fe absorption per plant and the 59Fe absorption activity per root DW () in all P levels. The release of phytosiderophores is dependent on ATP (CitationTakagi 1990). The ATP concentration in plant roots might be reduced by a low P level in the medium. Studies have shown that low P plants can release PS (CitationLadouceur et al. 2006); thus, ATP consumption for PS release might not be critical. It was noticeable that enhancement of the absorption of 59Fe by PS was found even under critical P-deficient conditions. In this experiment, although PS was equally fed to roots grown in high or low P conditions, 59Fe absorption by low P plants was still lower compared with that of plants grown in high P. The ATP concentration in roots may affect the absorption of the PS–59Fe complex. Further experiments are required to elucidate the relationship between PS–Fe absorption and ATP concentration in roots.
Figure 2 (a) Translocation, (b) concentration in shoots and (c) relative translocation rate of 59Fe to shoots in barley plants grown in Fe-deficient media with different P levels, with or without phytosiderophores (PS) (small letters for plants without PS and capital letters for plants with PS). DW, dry weight.
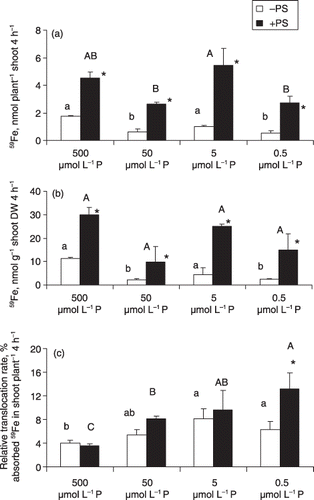
Translocation and the relative translocation rates of 59Fe to shoots
The translocation of 59Fe to the shoots in the –PS condition was not affected by low P levels (). The addition of PS significantly enhanced 59Fe translocation in each P level (). Translocation of 59Fe was enhanced 1.5-fold in high P (500 µmol L−1 P) plants and 3.2–4.3-fold in low P (50, 5 and 0.5 µmol L−1 P) plants. The concentration of 59Fe in shoots followed the trend of 59Fe translocation in shoots (). High P concentration in plant tissues may hamper Fe translocation to leaves (CitationHue and Nakamura 1988; CitationJones et al. 1972). Consistent with this result, we found that high P in plants decreased Fe mobilization in roots and, thus, decreased the rate of 59Fe translocation to the shoots.
Phytosiderophores-induced enhancement of 59Fe translocation was observed in all P levels. However, the translocation of 59Fe was enhanced at a higher ratio in low P media (). The PS–Fe3+ complex is first absorbed via a specific transporter in roots (CitationMurata et al. 2006) and then translocated to other parts of the plant (CitationRömheld and Marschner 1986; CitationTakagi et al. 1984). In barley, the addition of PS in Fe-stressed medium enhanced Fe translocation into the xylem tubes (CitationKawai and Alam 2006). Furthermore, it has been shown that the membrane protein that mediates the absorption of the PS–Fe3+ complex in maize (Zea mays L.) and the levels of ys1 mRNA (the gene encoding the membrane protein directly involved in the uptake of PS–Fe3+) increased in both shoots and roots under Fe-deficient conditions (CitationCurie et al. 2001). The activity for Fe translocation in plants at the loading site around the xylem tube may not be affected much by low P (50, 5 and 0.5 µmol L−1 P) conditions of the medium.
The relative translocation rate of 59Fe was higher in the low P (50, 5 and 0.5 µmol L−1 P) plants than in the high P (500 µmol L−1 P) plants with or without PS (). It appeared that the high P condition depressed the relative translocation rate of 59Fe to the shoots. This reduced relative translocation rate of 59Fe to shoots may result from the immobilization of 59Fe in the form of phosphate–iron complexes in root cells (CitationOh et al. 1996). Furthermore, it has also been shown that Fe forms insoluble oxides, makes complexes with phosphates and phytoferritin in older leaves and other plant parts, which also immobilize Fe (CitationOh et al. 1996). The immobilization of Fe may subsequently diminish its movement into the phloem for long-distance translocation (CitationTaiz and Zeiger 2002). The relatively low translocation of Fe under high P (500 µmol L−1 P) conditions may result in less availability of Fe in the sites of chlorophyll synthesis in leaves and subsequently induce leaf chlorosis. In contrast, the higher relative translocation rate of Fe in low P (50, 5 and 0.5 µmol L−1 P) conditions possibly enhances the supply of Fe to the sites of chlorophyll synthesis in leaves and reverts the leaf chlorosis phenotype.
Figure 3 (a) Total absorption and (b) root absorption activity of apoplastic 59Fe in roots in barley plants grown in Fe-deficient media with different P levels, with or without phytosiderophores (PS) (small letters for plants without PS and capital letters for plants with PS). DW, dry weight.
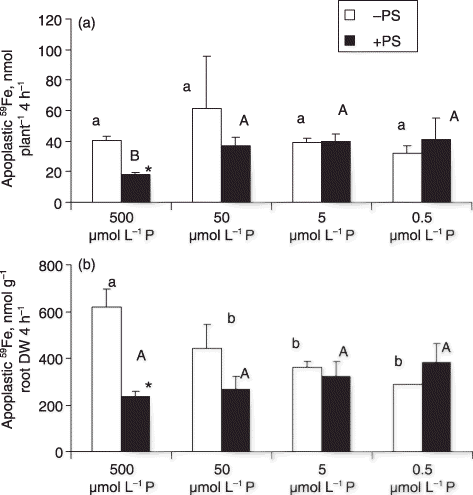
The effect of PS on the 59Fe relative translocation rate was observed only at the lowest P (0.5 µmol L−1 P) concentration (), suggesting that PS is more effective under severe P-deficient conditions. However, the physiological mechanism of this phenomenon is still unclear and needs to be further examined.
Apoplastic 59Fe in roots
The root apoplastic 59Fe per plant was not significantly affected by P levels in the –PS condition (). In the presence of PS, the apoplastic 59Fe per plant was higher in the low P (50, 5 and 0.5 µmol L−1 P) plants and lower in the high P (500 µmol L−1 P) plants (). We speculate that PS depressed the formation of apoplastic Fe–phosphate complexes in high P plants, probably through solubilization of Fe in the media, preventing adsorption of Fe to the cell wall or membrane. In the presence of high P (500 µmol L−1 P), apoplastic Fe forms an insoluble Fe–phosphate complex (CitationOh et al. 1996) and the presence of PS may depress the formation of such an Fe–phosphate complex. It has been shown that PS does not affect this parameter in low P media, but does decrease it in a high P (500 µmol L−1 P) medium.
The apoplastic 59Fe per root DW of the plants without PS was lower in low P (50, 5 and 0.5 µmol L−1 P) media than in the high P (500 µmol L−1 P) medium (). In the presence of PS, the concentration of apoplastic 59Fe was not significantly affected by the P level of the medium.
Without PS feeding, the ratio of the 59Fe content in root apoplast () to the total absorption of 59Fe per plant () was 0.9 in the high P (500 µmol L−1 P) condition, but was 5.2, 3.3 and 3.9 in the low P (50, 5 and 0.5 µmol L−1 P) conditions, respectively. With PS feeding, the ratio was 0.14 in the high P (500 µmol L−1 P) condition, while in the low P (50, 5 and 0.5 µmol L−1 P) conditions these values were 1.1, 0.7 and 2.0, respectively. In general, the ratio was higher in the –PS condition, suggesting that PS enhanced Fe absorption and prevented the formation of an apoplastic Fe complex containing phosphate in this short-term experiment. In addition, the ratio was much lower in the high P condition. We assume that there is a balance between the absorption and accumulation of apoplastic Fe. It appears that plants with a higher Fe demand, such as the plants in the 500 µmol L−1 P treatment, induce a lower ratio, indicating that Fe was preferentially absorbed rather than accumulated in apoplast. The mechanism of the formation of apoplastic Fe has not been clearly elucidated, and the function of apoplastic Fe has not been discussed sufficiently, other than playing a role as a reservoir of Fe in roots of wheat (CitationZhang et al. 1991). In soybeans, it has been shown that the accumulation of short-term Fe reserves in the root apoplast and the translocation of Fe in large quantities to the shoot may play an important role in making some cultivars resistant to Fe-deficiency induced chlorosis (CitationLongnecker and Welch 1990). In addition to the ratio of apoplastic Fe and total absorption of Fe, a low relative translocation rate in high P (500 µmol L−1 P) plants was shown (). This indicated that the high P condition immobilized more Fe in the roots. It will be interesting to investigate the spatial and chemical characteristics of the repression of Fe translocation in high P plant roots. Further investigation about the mechanism of the immobilization of Fe by P is necessary.
ACKNOWLEDGMENTS
A. Ladouceur thanks the Ministry of Education, Science and Culture of the Japanese Government for providing him with a scholarship that made possible this study. Thanks are due to Dr MD. Abul Kashem and Dr Abidur Rahman for their assistance with this manuscript.
REFERENCES
- Alam , S , Kamei , S and Kawai , S . 2005 . Effectiveness of phytosiderophores in absorption and translocation of 59Iron in barley in the presence of plant borne, synthetic, and microbial chelators . J. Plant Nutr , 28 : 1709 – 1722 .
- Bienfait , HF , van den Briel , W and Mesland-Mul , NT . 1985 . Free space iron pools in roots. Generation and mobilization . Plant Physiol , 78 : 596 – 600 .
- Brown , JC . 1961 . Iron chlorosis in plants . Adv. Agron , 13 : 323 – 369 .
- Curie , C , Panaviene , Z , Loulergue , C , Dellaporta , SL , Briat , J-F and Walker , EL . 2001 . Maize yellow stripe1 encodes a membrane protein directly involved in Fe(III) uptake . Nature , 409 : 346 – 349 .
- DeKock , PC and Alexander , H . 1955 . The phosphorus–iron relationship in genetical chlorosis . Plant Physiol , 30 : 293 – 296 .
- Hartwig , RC and Loeppert , RH . 1993 . “ Evaluation of soil iron ” . In Iron Chelation in Plants and Soil Microorganisms , Edited by: Barton , LL and Hemming , BC . 465 – 482 . San Diego : Academic Press .
- Hue , NV and Nakamura , ET . 1988 . Iron chlorosis in macadamia as affected by phosphate–iron interactions . J. Plant Nutr , 11 : 1635 – 1648 .
- Jones , RC , Shigeura , GT and Uehara , G . 1972 . Microprobe investigation of phosphorus-induced chlorosis in macadamia . Hawaii Farm Sci , 21 : 2 – 3 .
- Kawai , S and Alam , S . 2006 . “ Iron stress response and composition of xylem sap of strategy II plants ” . In Iron Nutrition in Plants and Rhizospheric Microorganisms , Edited by: Barton , LL and Abadia , J . 279 – 288 . Dordrecht : Springer .
- Kawai , S , Takagi , S and Sato , Y . 1988 . Mugineic acid-family phytosiderophores in root secretions of barley, corn and sorghum varieties . J Plant Nutr , 11 : 633 – 642 .
- Ladouceur , A , Tozawa , S , Alam , S , Kamei , S and Kawai , S . 2006 . Effect of low phosphorus and iron-deficient conditions on phytosiderophore release and mineral nutrition in barley . Soil Sci. Plant Nutr , 52 : 203 – 210 .
- Lindsay , WL . 1984 . Soil and plant relationship associated with iron deficiency with emphasis on nutrient interactions . J. Plant Nutr , 7 : 489 – 500 .
- Lindsay , WL and Schwab , AP . 1982 . The chemistry of iron in soils and its availability to plants . J. Plant Nutr , 5 : 821 – 840 .
- Longnecker , N and Welch , RM . 1990 . Accumulation of apoplastic iron in plant roots: a factor in the resistance of soybeans to iron-deficiency induced chlorosis . Plant Physiol , 92 : 17 – 22 .
- Mengel , K and Kirkby , EA . 2001 . “ Phosphorus and iron ” . In Principles of Plant Nutrition , 5th edn , Edited by: Mengel , K and Kirby , EA . 453 – 570 . Dordrecht : Kluwer Academic Publishers .
- Murata , K , Ma , JF , Yamaji , N , Ueno , D , Nomoto , K and Iwashita , T . 2006 . A specific transporter for iron(III)-phytosiderophore in barley roots . Plant J , 46 : 563 – 572 .
- Oh , SH , Cho , SW , Kwon , TH and Yang , MS . 1996 . Purification and characterization of phytoferritin . J. Biochem. Mol. Biol , 29 : 540 – 544 .
- Pushnik , JC , Miller , GW and Manwaring , JH . 1984 . The role of iron in higher plant chlorophyll biosynthesis, maintenance and chloroplast biogenesis . J. Plant Nutr , 7 : 733 – 758 .
- Römheld , V and Marschner , H . 1986 . Evidence for a specific uptake system for iron phytosiderophores in roots of grasses . Plant Physiol , 80 : 175 – 180 .
- Römheld , V and Marschner , H . 1991 . “ Function of micronutrients in plants ” . In Micronutrients in Agriculture , Edited by: Mortvedt , JJ , Cox , FR , Shuman , LM and Welch , RM . 297 – 328 . Madison : Soil Science Society of America .
- SAS Institute . 1988 . SAS/STAT User's Guide , No.1, anova, Version 6 Cary : Statistical Analysis System Institute .
- Srinivasarao , Ch , Ganeshamurthy , AN , Ali , M and Venkateswarlu , B . 2006 . Phosphorus and micronutrient nutrition of chickpea genotypes in a multi-nutrient-deficient typic ustochrept . J. Plant Nutr , 29 : 747 – 763 .
- Taiz , L and Zeiger , E . 2002 . “ Mineral nutrition ” . In Plant Physiology , 3rd edn , Edited by: Taiz , L and Zeiger , E . 67 – 86 . Sunderland : Sinauer Associates .
- Takagi , S . 1990 . “ The iron acquisition system in graminaceous plants and mugineic acids ” . In Neutriophysiology of Metal Related Compounds , Edited by: Japanese Society of Soil Science and Plant Nutrition . 6 – 51 . Tokyo : Hakuyusha .
- Takagi , S . 1993 . “ Production of phytosiderophores ” . In Iron Chelation in Plants and Soil Microorganisms , Edited by: Barton , LL and Hemming , BC . 111 – 131 . New York : Academic Press .
- Takagi , S , Nomoto , K and Takemoto , T . 1984 . Physiological aspect of mugineic acid, a possible phytosiderophore of graminaceous plants . J. Plant Nutr , 7 : 469 – 477 .
- Tang , C , Robson , AD and Dilworth , MJ . 1990 . The role of iron in nodulation and nitrogen fixation in Lupinus angustifolius L . New Phytol , 114 : 173 – 182 .
- Vance , CP , Uhde-Stone , C and Allan , DL . 2003 . Phosphorus acquisition and use, critical adaptation by plants for securing a non-renewable resource . New Phytol , 157 : 423 – 447 .
- Welch , RM , Allaway , WH , House , WA and Kubota , J . 1991 . “ Geographic distribution of trace element problems ” . In Micronutrients in Agriculture , Edited by: Mortvedt , JJ , Cox , FR , Shuman , LM and Welch , RM . 31 – 57 . Madison : Soil Science Society of America .
- Zarcinas , BA , Cartwright , B and Spouncer , LR . 1987 . Nitric acid digestion and multi-element analysis of plant material by inductively coupled plasma spectrometry . Commun. Soil Sci. Plant Anal , 18 : 131 – 146 .
- Zhang , F , Römheld , V and Marschner , H . 1991 . Role of the root apoplasm for iron acquisition by wheat plants . Plant Physiol , 97 : 1302 – 1305 .