Abstract
Field experiments were designed to quantify N2O emissions from corn fields after the application of different types of nitrogen fertilizers. Plots were established in South Kalimantan, Indonesia, and given either urea (200 kg ha−1), urea (170 kg ha−1) + dicyandiamide ([DCD] 20 kg ha−1) or controlled-release fertilizer LP-30 (214 kg ha−1) prior to the plantation of corn seeds (variety BISI 2). Each fertilizer treatment was equivalent to 90 kg N ha−1. Plots without chemical N fertilizer were also prepared as a control. The field was designed to have three replicates for each treatment with a randomized block design. Nitrous oxide fluxes were measured at 4, 8, 12, 21, 31, 41, 51, 72 and 92 days after fertilizer application (DAFA). Total N2O emission was the highest from the urea plots, followed by the LP-30 plots. The emissions from the urea + DCD plots did not differ from those from the control plots. The N2O emission from the urea + DCD plots was approximately one thirtieth of that from the urea treatment. However, fertilizer type had no effect on grain yield. Thus, the use of urea + DCD is considered to be the best mitigation option among the tested fertilizer applications for N2O emission from corn fields in Kalimantan, Indonesia.
INTRODUCTION
In recent years, concern over global warming and consequent climate change has led to a worldwide interest in the processes involved. It is necessary to examine ways to mitigate greenhouse gas emissions, including nitrous oxide (N2O). Soils contribute 70% to the global budget of N2O emissions (CitationBouwman 1990). In soils, N2O is formed by bacterial action during both nitrification and denitrification (CitationPaul and Clark 1996). CitationWatsuji et al. (2003) showed that fungal denitrification can also produce N2O. Indeed some studies have demonstrated that nitrification can be the main source of N2O emissions from soils (CitationBremmer and Blackmer 1979; CitationFreney et al. 1979; CitationInubushi et al. 1996). Similar results have been reported by CitationNakajima et al. (1992) from tropical upland soils.
Nitrification is carried out by a great number of microbes, but the two most classically recognized genera of bacteria are Nitrosomonas and Nitrobacter. Nitrosomonas as well as Nitrosospira and other ammonium oxidizers convert NH+ 4 to NO− 2 and Nitrobacter facilitates a further step by converting NO− 2 to NO− 3 (CitationBremmer and Blackmer 1981). The N2O is released as a by-product of nitrification. However, more information is required to mitigate N2O emissions, especially from tropical soils.
In tropical agricultural soils, nitrogen (N) fertilization is often considered to be the main source of N2O emissions, as in temperate soils. CitationCrill et al. (2000) found that N2O emissions from fertilized plots were approximately fivefold greater than those from unfertilized plots in Costa Rican soils. CitationMosier and Delgado (1997) reported that nearly 4% of applied N was lost in the form of N2O in Puerto Rican soils. Therefore, efficient agricultural practices, including the use of appropriate N fertilizer, are needed if the N2O emissions from tropical soils are to be minimized.
In South Kalimantan, Indonesia, urea is the most widely used N fertilizer, accounting for more than 95% of N application (CitationDewan Ketahanan Pangan Kalsel 2002). Urea is mainly applied to commonly cultivated annual crops, such as paddy, corn and soybean. CitationKhalil et al. (2002) measured N2O emissions during an annual groundnut–corn crop rotation on the Malaysian peninsula and found the highest N2O emissions during the corn part of the cropping sequence. However, there are no reports regarding the effect of urea application and mitigation options on the emission of N2O from corn fields in Kalimantan or from other Indonesian regions.
Table 1 Soil properties of the plough layer of the soils used
Dicyandiamide (DCD) is widely used in temperate regions to moderate N loss by leaching. In addition, the effectiveness of DCD in reducing N2O emissions has been reported mostly from temperate zones (CitationAmberger 1989; CitationDi and Cameron 2006). Dicyandiamide is made by dimerization of cyanamide and contains approximately 67% N (CitationAmberger 1989). Many different types of controlled-release fertilizers with various characteristics have been developed in Japan, including LP-30, a urea fertilizer coated with thermoplastic polyolifin (CitationShoji and Gandeza 1992). However, the possible uses of these sources of N in corn fields in the tropics are poorly understood. Therefore, this study was designed to quantify N2O emissions from a tropical agricultural field after the application of different types of N fertilizers. The objective of the study was to determine which fertilizers, if any, can mitigate N2O emissions from corn fields in Kalimantan, Indonesia.
MATERIALS AND METHODS
Twelve plots measuring 4 m × 5 m each were established in the Cempaka sub-district, South Kalimantan, Indonesia (03°28′45″S; 114°50′47″E). The plots were ploughed as per the farmers’ practice. The soil used was an Ultisol. The properties of the plough layer soil are given in .
Lime and cow dung were applied at rates of 2 and 4 ton ha−1, respectively, 3 weeks before planting. The plots received either urea (200 kg ha−1), urea (170 kg ha−1) + DCD (20 kg ha−1) or controlled-release fertilizer LP-30 (214 kg ha−1); each fertilizer treatment was equivalent to 90 kg N ha−1. Plots without N fertilizer were used as control plots. The controlled-release fertilizer, LP-30, is urea coated with thermoplastics polyolefin (CitationShoji and Gandeza 1992). The number “30” indicates that the fertilizer is designed to release 80% of the N at 25°C in the 30 days after application. Dyciandiamide (H2NC(=NH)NHCN) is a nitrification inhibitor that blocks the activity of ammonia monooxygenase enzyme, thus inhibiting the oxidation of ammonia to nitrite (CitationSylvia et al. 2005).
All N fertilizers were applied by top dressing once, on the day of planting, except for the urea treatment. Two-thirds of the urea was applied on the day of planting and the remaining one-third was applied 35 days after planting using a similar method to the other N fertilizers. The field was designed to have three replicates for each treatment using a randomized block experimental design. Super phosphate, 250 kg ha−1 (39.3 kg P ha−1), and KCl, 100 kg ha−1 (39.3 kg K ha−1), were applied as basal fertilizers. Basal fertilizers were placed 10 cm from a plant at a depth of 5 cm as a band application on the day of planting.
Corn seeds (variety BISI 2) were planted (9 November 2004) at a rate of three seeds per hole, with a spacing of 20 cm × 75 cm in a west–east orientation. Pest and disease controls were carried out when necessary as per the farmers’ practice until harvest. Harvest was done manually by picking the ear of the stem and removing the grain. The plants were cut at the soil surface and dried at 70°C for 48 h to measure above-ground biomass.
Nitrous oxide flux (from the soil surface to the atmosphere) was determined at 4, 8, 12, 21, 31, 41, 51, 72 and 92 days after fertilizer application (DAFA) using a closed chamber as described by CitationHadi et al. (2000). In brief, the chamber was constructed of a polyvinylchloride pipe (21 cm in diameter and 14 cm in length) that was inserted into the band of fertilizer in between the plants. Gas samples (inside the chamber) were collected by inserting a 35 mL syringe into a tube attached to the chamber and then sucking the air out. Four gas samples were collected from each chamber at 0, 5, 10 and 15 min after the enclosure was established. The concentration of N2O in each chamber was measured using a gas chromatograph equipped with an electron capture detector (Shimadzu, Kyoto, Japan). The flux was then calculated from the temporal increase in gas concentration inside the chamber per unit time (CitationYagi 1997).
Table 2 Nitrous oxide emission, number of ear of stem, grain yield and above-ground biomass as affected by fertilizer type in South Kalimantan
Figure 1 Nitrous oxide (N2O) emissions as affected by fertilizer application in a corn field in South Kalimantan. Error bars are standard deviation of the mean. DCD, dicyandiamide; LP-30, controlled-release fertilizer.
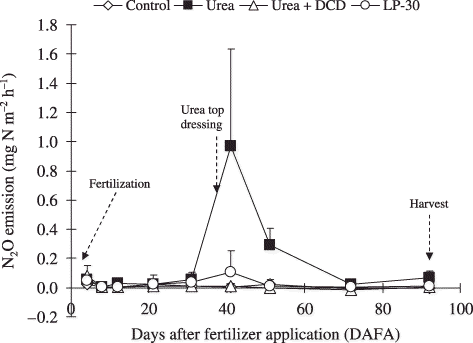
Along with the gas flux measurements on days when N transformations were expected to be high (i.e. 31 and 51 days after fertilizer application), soil-pore water was collected by inserting a water sampler (DAIKI-8390; Daiki Physichochemical Company, Tokyo, Japan) to a soil depth of 25 cm at the center of four hills of corn plants and sucking water into a 50 mL syringe. The water was then analyzed for inorganic N concentrations (i.e. NO− 3-N and NH+ 4-N). The NO− 3-N was reduced to nitrite by hydrazine sulfate under alkaline conditions and then analyzed colorimetrically (CitationHayashi et al. 1997). The NH+ 4-N was determined colorimetrically using a modified Berthelot reaction (CitationPage et al. 1982).
Soil samples were taken up to a depth of 30 cm at 4 and 41 DAFA (when N transformations were expected to be high) and analyzed for inorganic N concentrations, urease enzyme activity and microbial biomass C and N. The NO− 3 and NH+ 4 concentrations were determined by extraction of the soil with 1 mol L−1 KCl at an extractant : sample ratio of 10:1 (v/w). The extracts were filtered through Whatman 42 ash-free filter paper (Whatman International, Midstone, UK). Measurements were carried out colorimetrically as described previously for the soil-pore water. Urease enzyme activities were estimated using the method described by CitationPage et al. (1982), while biomass C and N were estimated using the chloroform fumigation–extraction method described by CitationInubushi et al. (1989).
All statistical analyses were carried out using SPSS 11.0 software for Windows (SSPS, Chicago, IL, USA). The frequency distributions of all data were first tested for normality using Kolmogorov–Smirnov tests. If the data were normally distributed, differences between treatments were determined by anova and least significant differences (LSD) tests. A simple linear correlation between the measured parameters was carried out. Results were considered significant at P < 0.05.
RESULTS
Patterns of N2O emissions were affected by the type of fertilizer (). The urea plots started to release considerable amounts of N2O at 31 DAFA and continued to release until 41 DAFA, which was 6 days after top dressing. The LP-30 plots released considerable amounts of N2O at 41 DAFA. In contrast, N2O emission from the urea + DCD and control plots remained low during the crop season.
Total N2O emission during the cultivation period was highest from the urea plots, followed by the LP-30 plots. Emissions from the urea + DCD plots did not differ significantly from the control plots (). The N2O emission from the urea + DCD plots was approximately one thirtieth of that from the urea treatment.
Fertilizer type affected the NH+ 4-N concentration, but not the NO− 3-N concentration of soil-pore water (). At 31 DAFA, the lowest NH+ 4-N of soil-pore water was observed in the urea plots, which did not differ from the control and urea + DCD plots. The highest NH+ 4-N of soil-pore water was observed in the LP-30 plots. At 51 DAFA, the lowest NH+ 4-N in soil-pore water was observed in the urea + DCD plots, which did not differ from the LP-30 and control plots. The highest NH+ 4-N of soil-pore water was observed in the urea plots. The proportions of NH+ 4-N in inorganic N were generally more than NO− 3-N in soil-pore water.
Figure 2 Inorganic N contents of soil-pore water in South Kalimantan. Different lowercase letters indicate significant differences in nitrate concentrations among the treatments at P < 0.05. Different capital letters indicate significant differences in ammonium concentrations among the treatments at P < 0.05. Error bars are standard deviation of the mean. DCD, dicyandiamide; LP-30, controlled-release fertilizer.
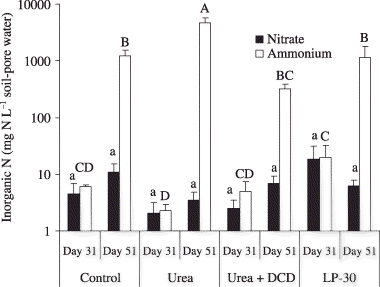
Figure 3 Inorganic soil N contents in South Kalimantan. Different lowercase letters indicate significant differences in nitrate concentrations among the treatments at P < 0.05. Different capital letters indicate significant differences in ammonium concentrations among the treatments at P < 0.05. Error bars are standard deviation of the mean. DCD, dicyandiamide; LP-30, controlled-release fertilizer.
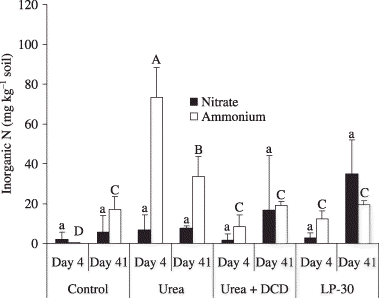
Fertilizer type affected the soil NH+ 4-N concentration, but not the NO− 3-N concentration at 4 DAFA (). Soil NH+ 4-N concentration in the urea plots was the highest at 4 DAFA. The lowest NH+ 4-N was detected in the control plot at 4 DAFA, when the ammonium concentration was negligible. No significant differences in soil inorganic N were recorded in the other treatments.
The type of fertilizer had no effect on either urease enzyme activity or microbial biomass C and N at the corn harvest stage. Urease enzyme activity ranged from 18.7 ± 9.4 (mean ± standard deviation) µg N g−1 soil 2 h−1 in the urea plots to 59.8 ± 9.3 µg N g−1 soil 2 h−1 in the control plots. The microbial biomass C ranged from 62.2 ± 21.6 mg C kg−1 soil in the control plots to 211.5 ± 70 mg C kg−1 soil in the urea plots, while biomass N ranged from 0.1 mg N kg−1 soil in the control plots to 1.1 mg N kg−1 soil in the urea plots.
Neither number of ear of stem nor grain yield was affected by fertilizer application (). Above-ground plant biomass was the highest in the LP-30 plots, which was not different from that in the urea + DCD plots. The lowest above-ground plant biomass was observed in the control plots.
There was a significant correlation between the NH+ 4-N contents of soil-pore water and N2O flux at 51 DAFA (r = 0.92). Total N2O emission (i.e. over the 4–92-day period) was also significantly correlated with gas fluxes at 41 (r = 0.99), 51 (r = 0.88) and 92 (r = 0.66) DAFA. The total emission (Y, mg N m−2) can be estimated by N2O fluxes at 41, 51 or 92 DAFA using the following regression equations: Y = 298.25 × flux at 41 DAFA + 27.13, Y = 1,930.5 × flux at 51 DAFA + 38.913 and Y = 4,631.1 × flux at 92 DAFA + 109.1, respectively. However, neither soil-pore water N, soil N nor N2O flux were correlated with plant production.
DISCUSSION
The patterns of N release are specific for a particular fertilizer when the soil properties and conditions are similar among the different fertilizer treatments. It is likely that the different patterns of N2O emissions observed in the current study can be attributed to N release patterns from the different treatments. Urea is considered to be a fast-release N fertilizer and started to release considerable amounts of N2O earlier than the other fertilizers (i.e. 21 DAFA; ), although active nutrient uptake by corn suppressed the N2O emissions after fertilizer application.
Table 3 Nitrous oxide emission indices
It appears that the release of N from LP-30 in this study met the N needs of the corn. This was also reflected in the N2O pattern, which remained negligible until 41 DAFA when some N2O were released from the plot.
The presence of DCD seemed to delay the formation of N2O. This may be because of an inhibition effect on nitrification processes. The result obtained in the present study, that N2O from the urea + DCD treatment was lower than the other N fertilizer treatments, has also been reported previously by CitationMosier et al. (1996) and CitationBronson and Singh (1995). Nitrogen loss in the form of N2O from the urea + DCD plots was only one thirtieth of that from the urea plot, suggesting that the use of urea + DCD was effective in minimizing N2O emissions from corn fields in the tropics.
The concentrations of inorganic N in the soil may also be explained by the release patterns of the N contained in each fertilizer. Urea in the urea plots was possibly hydrolyzed soon after the application of urea, and resulted in a flush of NH+ 4 into the soil () with relatively low cation exchange capacity (). In the urea + DCD and LP-30 plots, the release of NH+ 4 was delayed as a result of the protection effect of DCD or the coating material of LP-30 (CitationPaul and Clark 1996; CitationShoji and Gandeza 1992).
A significant positive correlation between total N2O emission and NH+ 4-N concentrations in soil-pore water suggest that soil-pore water plays an important role in N2O formation and emission. CitationYan et al. (2000) also reported a strong relationship between NH+ 4-N concentration in soil water and N2O emissions from paddy fields.
The lack of statistical significance between soil or water parameters and plant production (data not shown) indicated that the plant had not been determined only by the observed parameters. Light density, temperature and precipitation are major environmental factors controlling plant growth and development. Similar environmental factors at the experimental sites might result in similar grain yields among treatments ().
Significant correlations between total N2O emission and N2O fluxes at 41, 51 and 92 DAFA suggest that the N2O fluxes at 41, 51 and 92 DAFA are an important part of the measurements to determine total N2O emissions from corn fields in the tropics. This might be related to the generative growth of corn when less N was taken up by the plants. Thus, any additional mitigation option should be done on these days if the total N2O emission is to be reduced.
Emission indices (ratio of greenhouse gas emission over crop production) should be assessed when deciding on agricultural technology (CitationKimura et al. 1991; CitationNugroho et al. 1994). This notion is in line with the mitigation concept, which is defined as a reduction in gas emission without any reduction in crop production. Considering the emission indices, the presence of DCD along with urea lowered the value compared with urea alone (). This suggests that the addition of DCD can obtain the same corn product as urea while reducing the environmental pollution caused by N2O emission. The use of urea + DCD also results in labor cost reduction with a single basal application. Moreover, DCD can minimize NO− 3 leaching losses, avoid NO− 3 stress and the associated increased susceptibility of the plant to fungal infection (CitationAmberger 1989). Therefore, the use of urea + DCD can be considered to be the best mitigation option for N2O emission from corn fields in Kalimantan, Indonesia.
ACKNOWLEDGMENTS
This study was managed under the project “SSCP-3a: Development and evaluation of mitigation technologies for CH4 and N2O emissions from agroecosystems” supported by the Global Environmental Fund, the Ministry of the Environment, Government of Japan (B-S-2-3a). This paper was presented at the “International Workshop on Monsoon Asia Agricultural Greenhouse Gas Emissions (MAGE-WS)”, 7–9 March 2006, Tsukuba, Japan. We thank Dr[i1]. ME. Tachibana of Chissoasahi Fertilizer Co., Ltd. for providing the fertilizer tested, and Miss ER Terawatt Magnate and Miss Witan Dalianis of Lambung Mangkurat University for help with the sample collections.
References
- Amberger , A . 1989 . "Research on DCD as a nitrification inhibitor and future outlook . Comm. Plant Soil Anal. , 20 : 1933 – 1955 .
- Bouwman , AF . 1990 . "Introduction ” . In Soils and the Greenhouse Effect , Edited by: Bouwman , AF . 25 – 35 . New York : John Wiley & Sons .
- Bremmer , JM and Blackmer , AM . 1979 . "Effect of acetylene and soil water content on emission of nitrous oxide from soil . Nature , 280 : 380 – 381 .
- Bremmer , JM and Blackmer , AM . 1981 . "Terrestrial nitrification as a source of atmospheric nitrous oxide ” . In Denitrification, Nitrification and Atmospheric Nitrous Oxide , Edited by: Delwiche , CC . 151 – 170 . New York : Wiley and Son .
- Bronson , KF and Singh , U . 1995 . "Nitrous oxide emission from flooded rice ” . In Climate Change and Rice , Edited by: Peng , S , Ingram , KT , Neue , H-U and Ziska , LH . 116 – 121 . Berlin : IRRI-Springer .
- Crill , PM , Keller , M , Weitz , A , Grauel , B and Veldkamp , E . 2000 . "Intensive field measurement of nitrous oxide emissions from a tropical agricultural soil . Global Biochem. Cycles , 14 : 85 – 95 .
- Dewan Ketahanan Pangan Kalsel . 2002 . Food Tenacity Development Project Progress Report Banjarbaru
- Di , HJ and Cameron , KC . 2006 . "Nitrous oxide emissions from two dairy pasture soils as affected by different rates of a fine particle suspension nitrification inhibitor, dicyandiamide . Biol. Fertil. Soils , 42 : 472 – 480 .
- Freney , JR , Denmead , OT and Simpson , JR . 1979 . "Nitrous oxide emission from soil at low moisture contents . Soil Biol. Biochem. , 11 : 167 – 173 .
- Hadi , A , Inubushi , K , Purnomo , E , Frazie , F , Yamakawa , K and Tsurata , H . 2000 . "Effect of land-use changes on nitrous oxide (N2O) emission from tropical peatlands . Chemosphere – Global Change Sci. , 2 : 347 – 358 .
- Hayashi , A , Sakamoto , K and Yoshida , T . 1997 . "A rapid method for determination of nitrate in soil by hydrazine reduction procedure . J. Soil Sci. Plant Nutr. , 68 : 322 – 326 .
- Inubushi , K , Brookes , PC and Jenkinson , DS . 1989 . "Soil microbial biomass C, N and ninhydrin-N in aerobic and anaerobic soils measured by the fumigation–extraction method . Soil Biol. Biochem. , 23 : 737 – 741 .
- Inubushi , K , Naganuma , H and Kitahara , S . 1996 . "Contribution of denitrification and autotrophic and heterotrophic nitrification to nitrous oxide production in andosol soils . Biol. Fertil. Soil , 23 : 292 – 298 .
- Khalil , MI , Rosenani , AB , Van-Cleemput , O , Shamshuddin , Y and Fauziah , CI . 2002 . "Nitrous oxide emissions from an Ultisol of the humid tropics under maize–groundnut rotation . J. Environ. Qual. , 31 : 1071 – 1078 .
- Kimura , M , Miura , Y , Watanabe , A , Katoh , T and Haraguchi , H . 1991 . "Methane emission from paddy field (part 1), Effect of fertilization, growth stage and midsummer drainage: Pot experiment . Environ. Sci. , 4 : 265 – 271 .
- Mosier , AR and Delgado , JA . 1997 . "Methane and nitrous oxide fluxes in grasslands in western Puerto Rico . Chemosphere , 35 : 2059 – 2082 .
- Mosier , AR , Duxbury , JN , Freney , JR , Heinemeyer , O and Minami , K . 1996 . "Nitrous oxide emission from agriculture fields: assessment, measurement and mitigation . Plant Soil , 181 : 95 – 108 .
- Nakajima , Y , Ishizuka , S , Tsuruta , H , Iswandi , A and Murdiyarso , D . "Main microbial processes for nitrous oxide emissions from soils in different land-use pattern in Sumatra, Indonesia . Program and Abstract of International Workshop on Land-use Change and Greenhouse Gases . February 18–22 , Tsukuba, Japan. Soil C and Nutrient Cycling in the Tropics .
- Nugroho , SG , Lumbanraja , J Suprapto , H . 1994 . "Effect of intermittent irrigation of methane emission from an Indonesian paddy field . Soil Sci. Plant Nutr. , 42 : 609 – 615 .
- Page , AL , Miller , RH and Keeny , DR , eds. 1982 . Method of Soil Analysis , Madison : American Agronomy .
- Paul , EA and Clark , FE . 1996 . Soil Microbiology and Biochemistry , San Diego : Academic Press .
- Shoji , S and Gandeza , AT . 1992 . Controlled-Release Fertilizer with Polyolefin Resin Coating: Development, Properties and Utilization , Sendai : Konno Printing .
- Sylvia , DM , Hartel , PG , Furhmann , JJ and Zuberer , DA . 2005 . Principle and Application of Soil Microbiology , New Jersey : Pearson Education .
- Watsuji , TO , Takaya , N , Nakamura , A and Shoun , H . 2003 . "Denitrification of nitrate by the fungus Cylindrocarpon tonkinense . Biosci. Biotechnol. Biochem. , 67 : 1115 – 1120 .
- Yagi , K . 1997 . "Greenhouse gases emission and absorption ” . In Methods of Soil Environment Analysis , Edited by: Konno , T , Anzai , T Onikura , Y . 129 – 138 . Tokyo : Hakuyusha . (in Japanese)
- Yan , X , Du , L , Shi , S and Xing , G . 2000 . "Nitrous oxide emission from wetland rice soil as affected by the application of controlled-availability fertilizers and mid-season aeration . Biol. Fertil. Soil , 32 : 60 – 66 .