Abstract
To evaluate the relationship between the amount of available Silicon (Si) in paddy soils and their mineral properties on the Shounai Plain in Japan, which is formed from several parent materials, we evaluated the amount of available Si, the particle size distribution, the oxide composition of crystalline minerals and the amount of oxalate-extractable Si (Sio), iron (Feo) and aluminum (Alo) in the soil. The amount of available Si in the soil and the oxide content of the crystalline minerals differed among four soil groups that were distinguished by their clay mineral composition. There was no difference in the particle size distribution among the soil groups. The amount of available Si was positively related to the SiO2/Al2O3 ratio of clay, the CaO concentration of silt and fine sand, and the amounts of Sio, Feo and Alo in the soil. The amount of available Si in the soils was negative correlated with the Na2O and K2O concentrations of silt, the K2O concentration of fine sand, and the coarse sand content. These results suggest that the amount of available Si in soils is affected by the weathering resistivity of their minerals and that the particle size distribution and mineral composition are related to the available Si of the soils. Mineralogical properties, including the particle size distribution and mineral composition such as the SiO2/Al2O3 ratio × clay fraction content and the amounts of CaO and MgO in silt-sized particles, were positively correlated with the amount of available Si in the soil, but these correlations were not found for fine sand-sized particles. The Sio, Feo and SiO2/Al2O3 ratio × clay fraction contents contributed approximately 50% to the amount of available Si in the soils. The amount of available Si in the soil was divided into two groups according to the location of the paddy field. The amount of soil-available Si in the alluvial plain was affected by the geology upstream through the mineral composition.
INTRODUCTION
The application of silicon (Si) fertilizer to rice encourages dry matter production and increases the grain yield by increasing photosynthesis in the leaves (CitationAgarie et al. 1992) and improving plant growth (CitationAndo et al. 2002). In addition, Si increases the resistance or tolerance of rice to biotic and abiotic stresses (CitationMa 2004). The Si content in the rice leaves and stem at harvest varies greatly, ranging from 22 to 75 g kg−1. The application of Si to rice plants containing < 51 g kg−1 Si in the leaves and stems improves the yield and/or the resistance or tolerance to stresses (CitationImaizumi and Yoshida 1958). Rice plants obtain natural Si from irrigation water and soil; > 70% of the Si in rice is obtained from the soil (CitationHanzawa et al. 2002; CitationImaizumi and Yoshida 1958; CitationKurashima and Kohno1973; CitationSumida 1992). Therefore, the rate of application of Si to rice should be determined in terms of the amount of soil Si available to plants.
The Si concentration in the soil solution is affected by soil Si solubility and environmental conditions, such as pH, redox potential and temperature (CitationSumida 1992). Most Si in soil is in the form of amorphous or crystalline clay minerals and primary minerals and complexes with sesquioxides. These minerals release Si to the soil solution through chemical weathering. The rate of chemical weathering of minerals is affected by the type and particle size of the minerals (CitationCarroll 1974; CitationDrever 1994; CitationJackson et al. 1948, Citation1952). The weathering resistivity of 1:1-type clay minerals is higher than that of 2:1-type clay minerals (CitationJackson et al. 1948, Citation1952). The weathering resistivity of primary minerals, such as alkaline feldspar and biotite, in which the potassium (K) and sodium (Na) content are relatively high, is greater than that of other primary minerals, such as plagioclases, amphibole and pyroxene, which contain calcium (Ca) and/or magnesium (Mg) (CitationCarroll 1974). Silicon combined with free iron oxide dissolves easily when the redox potential of the soil is reduced (CitationSchwertmann 1991). Therefore, sesquioxides, such as Al and Fe oxides, and hydroxides play an important role in controlling the Si concentrations in the soil solution (CitationJones and Handreck 1963; CitationMcKeague and Cline 1963). There are also reports that the amount of available Si in soils is affected by the clay content (CitationSchwandes et al. 2001; CitationTakahashi and Sato 2000) and by the parent material of the soil (CitationImaizumi and Yoshida 1958), suggesting that the amount of available Si could be influenced by the mineral composition and particle size. However, there is no information on the relationship between available Si and particle size distribution with reference to the mineral composition of the soil.
Figure 1 Location of the Shounai Plain experimental site and the distribution of the soil sampling points.
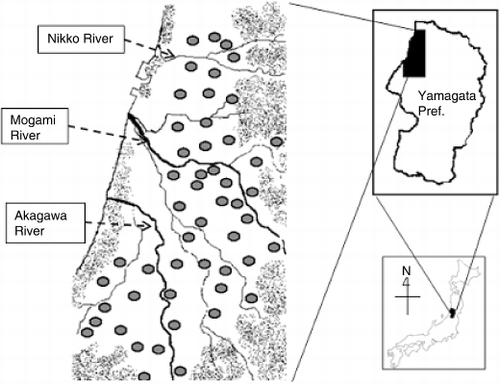
The Shounai Plain, Japan, is a region that has one of the highest yields of rice cultivation; 87% of the cultivated land is used as paddy fields (CitationTohoku Regional Agricultural Administration Office 2007). However, there are differences in the parent materials among the paddy soils of the Shounai Plain because the soils originate from several rivers that have different upstream geological features, which affect the mineral composition and particle size distribution of the soils. Thus, we investigated the relationship between the amount of available Si in soil and the particle size distribution with reference to the mineral composition of alluvial paddy soils on the Shounai Plain.
MATERIALS AND METHODS
Experimental site
Soil samples were collected from paddy fields on the Shounai Plain (38°36′–39°4′N and 139°42′–140°1′E), located in north-west Yamagata Prefecture, Japan. The alluvial plain and paddy soils originate from the Nikko River, the Mogami River and the Akagawa River deposits. The main parent materials of the Nikko, Mogami and Akagawa river basins are classified as andesite and tuff, tuff, and granite, respectively (CitationDepartment of Mining 1965).
Soil samples
Topsoil samples (0–10 cm) were collected from 51 alluvial paddy fields (49 Aquent and two Fuluvent) before the cropping season of 2003 (). The soil samples were air-dried and passed through a 2-mm sieve. The chemical properties of the air-dried soils were: soil pH (1:1 soil : water) 4.7–6.2 (mean 5.4); total C and N contents (assessed using a CN Corder, MT-700, Yanaco Analytical Instruments Co., Kuze, Kyoto, Japan) 15.7–50.6 and 1.6–4.2 g kg−1 (mean 33.4 and 2.9 g kg−1), respectively; available phosphorus content (CitationTruog 1930) 27.9–204.8 mg kg−1 (mean 83.5 mg kg−1). The number of soils belonging to each textural class (CitationGee and Bauder 1982) was 30 Light clay, 14 Clay loam, five Silty clay, one Heavy clay and one Sandy loam.
Soil analysis
The amount of available Si (mg kg−1) in the paddy soils was evaluated using the 0.02 mol L−1 phosphate buffer extraction method (CitationShigezumi et al. 2002). Four grams of soil and 40 mL of phosphate buffer solution (NaH2PO4 : Na2HPO4 = 1:1, pH 6.9) were placed into a 50-mL centrifuge tube, which was then placed into a water bath at 40°C. The tubes were shaken by hand at 0, 0.5, 1.0, 2.0, 3.0, 4.0 and 5.0 h from the start of the incubation. After 5 h of incubation, the tubes were centrifuged at 900 g for 5 min and then filtered to obtain the supernatant. The concentration of Si in the supernatant was determined by colorimetric analysis (CitationYanai et al. 1996).
To evaluate soil sesquioxides and Si combined with sesquioxides, the amounts of oxalate-extractable silicon (Sio), aluminum (Alo) and iron (Feo) were determined. Four hundred milligrams of soil and 40 mL of 0.2 mol L−1 oxalate solution ((NH4)2C2O4·H2O-H2C2O4·2H2O, pH 3.0) were placed in a 50-mL centrifuge tube. After shaking in the dark for 4 h at room temperature, the tube was centrifuged at 900 g for 5 min to obtain the supernatant (CitationBlakemore et al. 1981). The concentrations of Si, Al and Fe in the supernatant were determined by inductively coupled plasma atomic emission spectrometry (ICP-AES).
The pipette method was used to assess the particle size distribution of the soil (CitationGee and Bauder 1982). The content of each particle size fraction was calculated using the following equation:
The mineral composition of the clay fraction (< 2 µm) was evaluated by X-ray diffraction (XRD) using the CuKα radiation and orientation method (CitationWada 1966). The primary mineral composition of the fine sand fraction was determined by powder XRD analysis.
Chemical composition of the crystalline minerals of each particle size fraction
The chemical composition of the crystalline minerals of the clay (< 2 µm), silt (2–20 µm) and fine sand (20–200 µm) fractions were obtained using the following methods. Organic matter, free Fe and amorphous minerals in the soils were removed using peroxide and dithionite citrate–sodium bicarbonate (CitationBlakemore et al. 1981). The clay size fraction was separated by sedimentation and the silt and fine-sand size fractions were collected by sieving (mesh size: 20 and 212 µm, respectively). After drying, the samples from each fraction were digested with hydrofluoric acid, perchloric acid, hydrochloric acid and nitric acid (CitationChin and Marion 1982). The concentrations of aluminum (Al), Fe, Ca, Mg, Na and K in the digested solutions were evaluated by ICP-AES. These element concentrations were expressed as oxides. The silica (SiO2) concentration (g kg−1) of each particle size fraction was estimated by subtraction from the sum of the content of Al2O3, Fe2O3, CaO, MgO, Na2O and K2O (g kg−1) in each fraction. The oxide content of each crystalline particle size fraction in the soil was calculated from the following equation:
RESULTS
Physicochemical and mineralogical properties of the soil
Paddy soils of the Shounai Plain were divided into four groups (A–D) according to their clay mineral composition. The main clay mineral of the A, B and C groups was smectite, whereas smectite and chlorite were the main clay minerals in group D. Subordinate clay minerals differed among the A, B and C groups, except for the mixed layer minerals and chlorite. The B group was characterized by kaolinite and illite. Zeolite was found only in the C group. The distribution of soil groups was as follows: A-group soils were located north of the Nikko River (apart from one sample); B-group soils occurred between the Nikko River and the Mogami River; C-group soils were distributed around the Mogami River; and D-group soils were located around the Akagawa River ().
The amount of available Si in the soils ranged from 26.8 to 92.5 g kg−1 (mean 51.9 g kg−1; ). The mean amounts of available Si in soils in the A and B groups (65.8 and 73.5 g kg−1, respectively) were significantly higher than the amounts in the C and D groups (49.2 and 40.7 g kg−1, respectively; ).
The amounts of Sio, Alo and Feo in the soils ranged from 0.26 to 1.15 g kg−1, from 1.94 to 6.50 g kg−1 and from 2.1 to 27.9 g kg−1, respectively (). The amounts of Sio were significantly higher in the A and B groups than in the C and D groups (P < 0.05). The Alo content of the A group was significantly higher than that of the C group (P < 0.05). The Feo content in the B group was significantly higher than that in the D group (P < 0.05).
The amount of clay, silt, fine sand and coarse sand in the soils was 133–499, 125–444, 93–544 and 9–280 g kg−1, respectively (). There were no differences in the amount of each particle size fraction among the soil groups ().
The oxide concentrations in the clay, silt and fine-sand fractions of each group are shown in . In the clay fraction, the silica : alumina ratio (SiO2/Al2O3) ranged from 2.12 to 4.33, and the mean SiO2/Al2O3 ratio was significantly lower in the D group (2.65) than in the other three groups (3.4–3.59; P < 0.05). In the silt fraction, the ranges and mean oxide concentrations (g kg−1) were: CaO 8.5–30.2 (15.2); MgO 8.46–34.3 (18.6); K2O 12.2–37.34 (19.2); and Na2O 12.3–30.9 (23.8). The concentrations of CaO and MgO were significantly higher in the A group than in the other three groups (P < 0.05), and the concentrations of Na2O and K2O were significantly higher in the C and D groups than in group A (P < 0.05). In the fine-sand fraction, the ranges and mean oxide concentrations (g kg−1) were: CaO 19.0–58.9 (31.1); MgO 4.2–42.3 (18.1); Na2O 20.1–48.2 (34.7); and K2O 12.5–31.3 (19.2). The concentration of CaO was significantly higher in group A than in the other three groups (P < 0.05). A higher concentration of Na2O and K2O in Group D than in C group (P < 0.05) was observed. There was no difference in MgO concentration among the four soil groups.
Relationship between available Si and the mineralogical properties of the soils
There was a negative correlation between the coarse-sand content and the amount of available Si in the soil (r = –0.389, P < 0.01; ). There were no correlations between particle size content and the amount of available Si in the soil for the other particle size fractions. Thus, the amount of available Si decreased with an increase in coarse-sand content, suggesting that the available Si in the soil did not originate from the coarse-sand fraction.
There were positive correlations between the contents of Sio and Feo in the soil and the amount of available Si (P < 0.001; ). The content of Alo in the soil was also positively correlated with the amount of available Si (P < 0.05). Thus, the amounts of sesquioxides and Si, which makes complexes with sesquioxides, were strongly related to the amount of available Si in the soil.
The amount of available Si in the soil was positively correlated with the SiO2/Al2O3 ratio of the clay fraction (). The concentration of CaO in the silt fraction was positively correlated with the amount of available Si in the soils. There were negative correlations between the concentrations of Na2O and K2O in the silt fraction and the amount of available Si in the soil. The K2O and CaO concentrations of the fine sand fraction were negatively and positively correlated, respectively, with the amount of available Si in the soil. Thus, the amount of available Si in the soil is related to the crystalline mineral composition in each particle size fraction, and the available Si increases with an increase in the amount of low-weathering-resistivity clay and primary minerals in each fraction.
To consider both the particle size fraction content and the weathering resistivity of each particle size fraction, we multiplied the oxide concentration of the particle size fraction by the particle size fraction content of the soil. The SiO2/Al2O3 ratio of the clay fraction × the clay fraction content and the amount of silt-sized CaO and MgO were significantly correlated with the amount of available Si in the soil (). There were no significant correlations between any oxide content in the fine sand-sized fraction and the amount of available Si in the soil (). Thus, the amount of available Si in the soil is related to the crystalline mineral composition of the finer particle size fractions in the soil.
Table 1 Physico-chemical and mineralogical properties of each soil group
We developed a correlation matrix of soil properties and the amount of available Si in the soil (). Coarse sand was negatively related to soil properties without Sio and Alo. High correlations were observed between the amounts of Sio and Alo, and between the amounts of silt-sized CaO and silt-sized MgO in the soil, and between the amount of Feo in the soil and the SiO2/Al2O3 ratio × clay fraction content.
The factors relating significantly to available Si (,,) were used as explanatory variables in forward regression analysis (). The adjusted multiple correlation coefficient was high (0.737, P < 0.001). The partial correlation coefficients of the Sio, Feo and SiO2/Al2O3 ratio × clay fraction content were 0.601, 0.288 and 0.285, respectively, but the coefficient of silt-sized CaO was not significant (P > 0.05). This suggests that approximately 50% of the amount of available Si can be attributed to the Sio and Feo contents in the soil and the SiO2/Al2O3 ratio × clay fraction content.
Table 2 Correlation coefficients between the particle size fraction and the available silicon in the soil
Table 3 Correlation coefficients between the extractable oxalate content and the available silicon in the soil
Table 4 Correlation coefficients between the oxide concentration of each particle size fraction and the available silicon in the soil
DISCUSSION
The amount of available Si in the soil decreased with increases in the coarse-sand fraction, but was unrelated to the amounts of the clay and silt fractions (). CitationTakahashi and Sato (2000) reported that the amount of available Si in paddy soils in the Hachirogata Reclaimed Land area of Japan was positively correlated with the amount of clay when the clay fraction was <290 g kg−1. CitationSchwandes et al. (2001) reported that the amount of available Si in soil in Ultisols in Florida, USA, was affected by the size of the clay fraction if the clay fraction was <150 g kg−1. These reports indicate that the size of the clay fraction is related to the amount of available Si in the soil, although the origins and clay mineral compositions of the soils were not clear. The clay fraction in Shounai Plain soils ranged from 133 to 498 g kg−1, and 61% of the soils investigated had < 290 g kg−1 clay content (data not shown). Nevertheless, the amount of available Si in these soils was not affected by the clay content (). However, the amount of available Si in the soils differed among the soil groups classified by the clay mineral composition (). Moreover, the clay, primary and sesquioxide compositions differed among the soil groups, but the particle size distribution did not (). The differences in the crystalline mineral and sesquioxide compositions were strongly related to the amount of available Si in the soils (, ). Therefore, the amount of available Si in Shounai Plain soils was more affected by differences in the mineral composition of each soil group than by the particle size distribution.
The weathering resistivity of 1:1-type clay minerals is higher than that of 2:1-type clay minerals (CitationJackson et al. 1948, Citation1952). The positive correlation between the SiO2/Al2O3 ratio of the clay fraction and the amount of available Si in the soil () might be affected by the weathering resistivity of the clay minerals. Alkaline feldspar and biotite have high K and Na contents, whereas plagioclases, amphibole and pyroxene are characterized by high Ca and Mg contents. The former minerals have higher weathering resistivity than the latter (CitationCarroll 1974). The weathering resistivity of these two groups was reflected in positive or negative correlations between available Si and the primary mineral composition of the silt and fine-sand fractions (). Consequently, the weathering resistivity of crystalline clay and primary minerals appears to affect the amount of available Si in the soil.
Because the oxide concentration of crystalline minerals in each particle size fraction indicates differences in the weathering resistivity of the mineral composition, both the oxide concentration of crystalline minerals in the particle size fraction and the content of the particle size fraction in the soil should be considered in evaluating the amount of available Si in the soil. The amount of available Si in the soil is related to the SiO2/Al2O3 ratio × clay fraction content, the silt-sized CaO and the silt-sized MgO content, but is not related to any fine sand-sized oxide content in the soil (). Because the MgO concentration was not correlated with the amount of available Si in the soil and silt-sized MgO was closely related to silt-sized CaO (), the significant correlation between silt-sized MgO content and the amount of available Si in the soil was interpreted as a spurious correlation. Consequently, the amount of available Si in the soil is strongly related to the weathering resistivity of the crystalline minerals in the finer fractions of the soil, and the contribution of the mineral composition of the larger particle size fraction to the amount of available Si is lower than that of the finer particle size fraction.
Table 5 Correlation coefficients between the available silicon in the soil and the crystalline mineral properties, including both the mineral composition and the particle content of particle size
Table 6 Correlation matrix of the soil properties and the available silicon in the soil
Table 7 Results of the regression analysis for available silicon and mineral properties of the soil
According to the regression analysis, the amount of available Si in the soil was regulated by the amounts of Sio and Feo and the SiO2/Al2O3 ratio × clay fraction content (). The largest contribution of Sio to the amount of available Si in the soil was found among explanatory variables. Si combine with sesquioxides is released to soil solution by desorption since Si concentration in soil solution is controlled by sesquioxides through adsorption and desorption of Si. Si combined with Feo is dissolved into soil solution with dissolution of Feo when soil redox potential reduced. Therefore, Sio is dissolved easier than Si in crystalline minerals. The maximum content of Sio in the top soil used in our study is 1.15 g kg−1 (; Group A). Therefore, the maximum amount of Sio is 1380 kg ha−1, assuming that the depth of the Ap layer is 12 cm and the bulk density is 1.0 g cm−3. The amount of Si in rice that originates from the soil is 350 kg ha−1 (yield of rice is 6 t ha−1, total amount of Si uptake by rice is 500 kg ha−1 and 70% of the Si in the rice comes from the soil (CitationImaizumi and Yoshida 1958). Because approximately 45–69% of the total Si in rice plants at harvest comes from the top soil (∼0–12 cm depth) (CitationSato 2002), it is estimated that the amount of Si in rice plants that comes from the top soil is approximately 158–242 kg ha−1 (350 kg ha−1× ∼0.45–0.69). This value is approximately one-fifth to one-ninth of the maximum content of Sio in the top soil. However, the amount of available Si in paddy soil don't decline for 5∼9 years in general. CitationSugiyama and Ae (2000) reported that some crops that have a high potassium uptake ability absorbed an insoluble form of potassium from the soil and the absorption of an insoluble form of potassium by the crops might involve the solubilization of soil Si under upland conditions. The present study suggested that Si in the crystal lattice of crystalline minerals was solubilized under upland conditions. The contribution and mechanism of crystalline minerals to the amount of available Si in the soil needs to be studied further under submerged conditions.
Free iron oxides adsorb Si (CitationJones and Handreck 1963; CitationMcKeague and Cline 1963). CitationTakahashi and Sato (2000) reported that a high content of free iron oxides in soil is advantageous for the retainment of Si. These reports agree with our results (). However, Feo was not correlated with Sio in our soils, hence, the role of free iron oxides on the availability of Si requires further study.
The mineral composition and particle size distribution of alluvial paddy soil are affected by the geology upstream of the river and the topography of the sedimentation (CitationShoji et al. 1973b). The parent materials of the A and B groups were andesite and tuff, the parent materials of the C group were tuff and granite and the parent material of the D group was granite (). The mineral composition was different among the soil groups, but the particle size distribution was not affected by the clay mineral composition or the soil groups (). The amount of available Si affected by the mineral composition of the A and B groups was higher than that of the C and D groups (). The Si concentrations in the stem and leaves of rice plants at the harvest stage in the A and B groups and in the C and D groups were approximately 56–65 g kg−1 and 42–51 g kg−1, respectively (unpubl. data; S. Makabe 2007). Consequently, the available Si in the soil, which affects the Si concentration of rice plants, is classified in these two areas by the clay mineral compositions of the alluvial paddy field in Shounai Plain. It might be said that distribution of soil available Si in alluvial plain formed by plural rivers was affected by the geology upstream of each river through mineral composition.
A negative correlation was found between the amounts of the coarse-sand fraction and the amounts of available Si in the soil using whole soil samples (), whereas soil texture was not related to the amounts of available Si in each soil group (data not shown). The distance from the river affects the soil texture and larger amounts of coarse sand are found in soils located further from the river (CitationShoji et al. 1973a). However, there was no obvious distribution pattern with regard to the amounts of the coarse-sand fraction in our experiment (data not shown). This might be attributed to the topography of the Shonai Plain, which is alluvial fan. Further studies need to be conducted to examine the relationship between the amounts of available Si in soil and soil texture within the same soil group.
Notes
Present address: †Industrial and Economic Affairs Department, Shonai Area General Branch Administration, Yamagata Prefectural Government 19-1, Sodehigashi, Yokoyama, Mikawa-mach, Higashitagawa-gun, 997-1392, Japan.
REFERENCES
- Agarie , S , Agata , W , Kubota , F and Kaufman , PB . 1992 . [Physiological roles of silicon in photosynthesis and dry matter production in rice plants. 1. Effect of silicon and shading treatments.] . Jpn. J. Crop Sci , 61 : 200 – 206 . (in Japanese with English summary)
- Ando , H , Kakuda , K , Fujii , H , Suzuki , K and Ajiki , T . 2002 . Growth and canopy structure of rice plants grown under field conditions as affected by Si application . Soil Sci. Plant Nutr , 48 : 429 – 432 .
- Blakemore , LC , Searle , PL and Daly , BK . 1981 . “ Methods for chemical analysis of soils ” . In New Zealand Soil Bureau Scientific Report 10A , Wellington, , New Zealand : Department of Scientific and Industrial Research .
- Carrol , D . 1974 . Rock Weathering , New York : Plenum Press .
- Chin , HL and Marion , LJ . 1982 . “ Dissolution for total elemental analysis ” . In Methods of Soil Analysis. Part 2: Chemical and Microbiological Properties , 2nd edn. , Edited by: Page , AL , Miller , RH and Keeney , DR . 1 – 11 . Madison : American Society of Agronomy, Inc.-Soil Science Society of America, Inc. .
- Department of Mining . 1965 . Geologic Map of Yamagata Prefecture , Yamagata, Japan : Department of Mining, Yamagata Prefecture .
- Drever , JI . 1994 . The effect of land plants on weathering rates of silicate minerals . Geochim. Cosmochim. Acta , 58 : 2325 – 2332 .
- Gee , GW and Bauder , JW . 1982 . “ Particle-size analysis ” . In Methods of Soil Analysis. Part 1: Physical and Mineralogical Methods , 2nd edn. , Edited by: Klute , A . 383 – 409 . Madison : American Society of Agronomy, Inc.-Soil Science Society of America, Inc. .
- Hanzawa , K , Suzuki , M , Yaginuma , T and Nogi , T . Dynamics of silicon in paddy fields with the reference to the amount of silicon in irrigation water . Proc. of the Second Silicon in Agriculture Conference . Aug 22–26 . Japan : Tsuruoka .
- Imaizumi , K and Yoshida , S . 1958 . [Edaphological studies on silicon-supplying power of paddy soils.] . Bull. Natl Inst. Agric. Sci , B8 : 261 – 304 . (in Japanese with English summary)
- Jackson , ML , Hseung , Y , Corey , RB , Evans , EJ and Vanden Heuvel , RC . 1952 . Weathering sequence of clay-size minerals in soils and sediments. II. Chemical weathering of layer silicates . Soil Sci. Soc. Am. Proc , 16 : 3 – 6 .
- Jackson , ML , Tyler , SA , Willis , AL , Bourbeau , GA and Pennington , RP . 1948 . Weathering sequence of clay-size minerals in soils and sediments. I. Fundamental generalizations . J. Phys. Coll. Chem , 52 : 1237 – 1260 .
- Jones , LHP and Handreck , KA . 1963 . Effect of iron and aluminum oxides on silica in solution in soils . Nature , 198 : 852 – 853 .
- Krashima , K and Kohno , M . 1973 . [Effect of underdrainage on the growth and yield of rice plant in ill-drained paddy fields.] . Bull. Hokuriku Agri. Exp. Stn , 15 : 27 – 75 . (in Japanese with English summary)
- Ma , JF . 2004 . Role of silicon in enhancing the resistance of plants to biotic and abiotic stresses . Soil Sci. Plant Nutr , 50 : 11 – 18 .
- McKeague , JA and Cline , MG . 1963 . Silica in soil solution. 2. The adsorption of monosilicic acid by soil and by other substances . Can. J. Soil Sci , 43 : 83 – 96 .
- Sato , N . Effects of cultivated condition on Si uptake from subsoil by rice plant . Proc. of the Second Silicon in Agriculture Conference . Aug. 22–26 . Japan : Tsuruoka .
- Schnitzer , M . 1982 . “ Organic matter characterization ” . In Methods of Soil Analysis. Part 2: Chemical and Microbiological Properties , 2nd edn. , Edited by: Page , AL , Miller , RH and Keeney , DR . 581 – 593 . Madison : American Society of Agronomy, Inc.-Soil Science Society of America, Inc. .
- Schwandes , LP , Snyder , GH and Wilkerson , J . 2001 . Plant-available silicon in selected alfisols and ultisols of Florida . Soil Crop Sci. Soc. Florida. Proc , 60 : 57 – 59 .
- Schwertmann , U . 1991 . Solubility and dissolution of iron-oxides . Plant Soil , 130 : 1 – 25 .
- Shigezumi , M , Kitta , Y , Kubo , S and Mizuochi , T . 2002 . [The evaluation of the available silica in paddy soil by the phosphate buffer extraction method.] . Jpn. J. Soil Sci. Plant Nutr , 73 : 383 – 390 . (in Japanese with English summary)
- Shoji , S , Ando , H and Masui , J . 1973b . [Clay minerals of paddy soils in Tohoku Districts (Part 3).] . Jpn. J. Soil Sci. Plant Nutr , 44 : 197 – 203 . (in Japanese)
- Shoji , S , Kaneko , S and Masui , J . 1973a . [Clay minerals of paddy soils in Tohoku Districts (Part 2).] . Jpn. J. Soil Sci. Plant Nutr , 44 : 81 – 88 . (in Japanese)
- Sugiyama , M and Ae , N . 2000 . [Uptake response of crops to potassium from andosol and potassium-bearing minerals applied to andosol.] . Jpn. J. Soil Sci. Plant Nutr , 71 : 786 – 793 . (in Japanese with English summary)
- Sumida , H . 1992 . Silicon-supplying capacity of paddy soils and characteristics of silicon uptake by rice in cool regions in Japan . Bull. Tohoku Agri. Exp. Stn , 85 : 1 – 46 . (in Japanese with English summary)
- Takahashi , T and Sato , A . 2000 . [Silicon fertility of paddy fields in Hachirogata Reclaimed Land, Akita Prefecture.] . Jpn. J. Soil Sci. Plant Nutr , 71 : 881 – 883 . (in Japanese)
- Tohoku Regional Agricultural Administration Office2007 Statistics of Agricultural, Forestry, and FisheriesAvailable from URL: http://www.maff.go.jp/tohoku/stinfo/toukei/ (http://www.maff.go.jp/tohoku/stinfo/toukei/)
- Truog , E . 1930 . The determination of the easily available phosphorus of soils . J. Am. Soc. Agron , 22 : 874
- Wada , K . 1966 . [Methods of analysis of soil. Qualitative and quantitative analysis of clay minerals.] . Jpn. J. Soil Sci. Plant Nutr , 37 : 9 – 17 . (in Japanese)
- Yanai , M , Yoshida , Y and Shimizu , Y . 1996 . [Colorimetric determination of acetate buffer extractable silicic acid with Imaizumi Yoshida method.] . Jpn. J. Soil Sci. Plant Nutr , 67 : 69 – 70 . (in Japanese)
- Present address: †Industrial and Economic Affairs Department, Shonai Area General Branch Administration, Yamagata Prefectural Government 19-1, Sodehigashi, Yokoyama, Mikawa-mach, Higashitagawa-gun, 997-1392, Japan.