Abstract
Barley (Hordeum vulgare L.) nicotianamine synthase gene (HvNAS1) expression in barley is strongly induced by Fe deficiency in the roots and rice (Oryza sativa L.) nicotianamine synthase gene (OsNAS1) expression in rice is induced by Fe deficiency both in the roots and in the shoots. In dicots, NAS genes are not strongly induced by Fe deficiency, and they function to maintain Fe homeostasis. Rice OsNAS1promoter::GUS or barley HvNAS1promoter::GUS was introduced into tobacco (Nicotiana tabacum L.) and tissue specificities and systemic regulation of their expression were compared. A split-root experiment revealed that the HvNAS1 promoter exhibited functions similar to those of Fe-acquisition-related genes in tobacco roots, suggesting that this promoter responded to certain Fe-deficiency systemic signals and to the Fe concentration in the rhizosphere. The HvNAS1 promoter might harbor a type of universal system of gene expression for Fe acquisition. However, the OsNAS1 promoter did not respond to local application of Fe to the roots and induced GUS activities in mature leaves in response to Fe deficiency. This promoter might possess numerous types of cis-acting sequences that are involved in Fe metabolism.
INTRODUCTION
Ferric iron (Fe3+) is generally precipitated in soil and plant bodies under aerobic or alkaline pH conditions; thus, Fe3+ exhibits low availability. In contrast, ferrous iron (Fe2+) is more likely to produce toxic radicals (CitationMarschner 1995). Therefore, plants have acquired chelation strategies for the solubilization or detoxification of Fe.
In Strategy I plants, which includes all dicots and non-graminaceous monocots, the reduction of ferric to ferrous ions at the root surface is an essential step in Fe uptake. The ferric-chelate reductase gene FRO has been cloned from dicots (CitationRobinson et al. 1999; CitationWaters et al. 2002). Following reduction, Fe2+ is absorbed via an iron-regulated transporter (IRT) (CitationVert et al. 2002). These mechanisms are induced by Fe deficiency. Nicotianamine, which chelates the divalent cation of transition metals, is constitutively essential for Fe distribution in Strategy I plants (CitationScholz et al. 1992). Grasses are Strategy II plants and use a chelation mechanism for Fe uptake. Strategy II plants synthesize mugineic acid family phytosiderophores (MAs), compounds that have a high affinity for Fe3+, and secrete these cpmpounds into the rhizosphere to chelate Fe3+. Nicotianamine is a key precursor of MAs in Strategy II plants (CitationShojima et al. 1990). The ferric iron–MA complex is absorbed via a specific transporter, namely, YS (CitationCurie et al. 2001). These mechanisms are induced by Fe deficiency. In addition, although rice has been categorized as a Strategy II plant it takes up Fe2+ via an IRT transporter, similar to the mechanism observed in Strategy I plants (CitationIshimaru et al. 2006). Thus, nicotianamine synthesized from S-adenosyl methionine by nicotianamine synthase (NAS) (CitationHiguchi et al. 1999) plays a key role in Fe nutrition in all higher plants. However, the expression patterns of NAS genes in response to the Fe nutritional status differ between plant species (CitationIto et al. 2007), and this difference might be correlated with the difference in the physiological functions of nicotianamine. Nicotianamine synthase might function in both Fe uptake and distribution in rice, and this represents an intermediate between Strategy I and Strategy II plants.
Recently, cis-acting elements and trans-acting factors regulating the expression of Fe-deficiency-related genes have been revealed. Several basic helix-loop-helix transcription factors, such as FER from tomato, OsIRO2 from rice and FIT from Arabidopsis, have been cloned (CitationColangelo and Guerinot 2004; CitationJakoby et al. 2004; CitationLing et al. 2002; CitationOgo et al. 2006), and the recognition sequence of OsIRO2 has also been revealed (CitationOgo et al. 2006). The expression of OsNAS genes is affected by the level of OsIRO2 expression in rice (CitationOgo et al. 2007). The regulatory components of OsIRO2, Fe-deficiency-responsive cis-acting elements IDE1 and IDE2, and the IDE1-binding and IDE2-binding trans-acting factors IDEF1 and IDEF2 have been discovered (CitationKobayashi et al. 2003, Citation2007; CitationOgo et al. 2007, Citation2008). In general, IDE1 and IDE2 are found in higher plants, and they are also found in the promoter regions of NAS genes. However, the complicated regulatory pathways for the expression of multiple NAS genes have not been fully elucidated.
We previously reported that the barley (Hordeum vulgare L.) HvNAS1 and rice (Oryza sativa L.) OsNAS1 promoters drove gene expression differently in transgenic tobacco (Nicotiana tabacum L.) (CitationIto et al. 2007). When fused to the β-glucuronidase (GUS) gene and introduced into tobacco, the HvNAS1 promoter induced GUS activity only in Fe-deficient roots; this is similar to its action in barley and rice. However, the OsNAS1 promoter induced GUS activity more strongly in Fe-deficient shoots than in Fe-deficient roots. This differs slightly from its action in rice; that is, the OsNAS1 promoter induced OsNAS1 expression in both the roots and shoot of rice, but it was considerably stronger in the roots than in the shoots (CitationInoue et al. 2003). This difference in the level of gene expression might not result solely from a difference in the affinity between cis-acting elements and the postulated trans-acting factors. The OsNAS1 promoter could respond to a regulatory system other than Fe acquisition in tobacco.
Recently, it has been revealed that the expression of Fe-acquisition-related genes is regulated by both systemic and local signals (CitationEnomoto et al. 2007; CitationVert et al. 2003). In the present study, we utilized the HvNAS1promoter:: GUS (HvNAS1pro::GUS) and OsNAS1promoter::GUS (OsNAS1pro::GUS) transgenic tobacco lines to compare the tissue specificities and systemic regulation of the expression patterns of the HvNAS1 and OsNAS1 promoters in detail in transgenic tobacco.
MATERIALS AND METHODS
Preparation of the transgenic tobacco
Homozygous T2 transformants of the HvNAS1pro::GUS transgenic tobacco plant were derived from transgenic tobacco harboring a 1.7-kb promoter fragment, which was amplified by polymerase chain reaction (PCR) from an HvNAS1 genomic clone template (CitationHiguchi et al. 2001a). Homozygous T2 transformants of the OsNAS1 pro::GUS transgenic tobacco plant were derived from transgenic tobacco harboring a 1.6-kb promoter fragment, which was amplified using PCR from a rice genomic DNA template (CitationInoue et al. 2003; CitationIto et al. 2007). These transformants were then cultured in a growth chamber at a temperature of 25 ± 1°C under a 16-h light (300 µmol s−1 m−2 µA−1)/8-h dark cycle.
Split-root experiment
Non-transformants or T2 transformants were cultured on Murashige and Skoog (MS) medium containing 0.2% gellan gum. We then transplanted 14-day-old seedlings into Petri dishes containing the split-root system. Each of the four compartments of the Petri dishes (100 mm × 15 mm Stackable Quad-Plate; Kord-Valmark, Brampton, Canada) was filled with MS medium with or without 100 µmol L−1 Fe-ethylenediaminetetraacetic acid (). The cotyledons of the seedlings were removed and the roots were washed with sterile water. The roots were divided into two groups and each group was placed in a different compartment (). The roots on the medium were covered and fixed with 1% agarose containing MS nutrients, except Fe. This dish containing the plants was placed in a large, sterile, glass Petri dish (120 mm × 60 mm); the dish was covered and then sealed with adhesive tape. The roots of two plantlets were harvested 3, 5 or 7 days after each treatment and used for the GUS assay or for the northern blot analysis.
Northern blot analysis
The partial Fe(III)-chelate reductase gene fragment from tobacco (NtFRO) was used for the northern blot analysis (CitationIto et al. 2007). The probe for NtFRO was labeled with [32P]dCTP using a Random Primer Labeling Kit Ver. 2 (TaKaRa, Shiga, Japan). Total RNA was extracted from the roots and leaves of the plant using the RNeasy Plant Mini Kit (Qiagen K.K., Tokyo, Japan). As described in CitationHiguchi et al. (2001b), 15 µg of the total RNA was separated, blotted and hybridized at 65°C using the probes. Radioactivity was then detected using a BAS-5000 image analyzer (Fuji Film, Tokyo, Japan).
Figure 1 Apparatus for the split-root experiment. Each compartment was filled with medium with or without Fe.
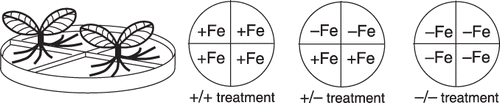
Figure 2 Northern blot analysis for the detection of NtFRO expression in the roots of non-transformants 7 days after the split-root treatment. Lane 1, +/+ treated roots; lane 2, Fe-supplied split roots of the +/– treated plant; lane 3, Fe-deficient split roots of the +/– treated plant; lane 4, –/– treated roots. Each lane was loaded with 15 µg of total RNA.
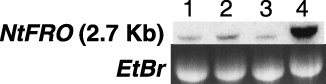
Fluorometric analysis of the GUS activity
The GUS activity was assayed according to the method of CitationKobayashi et al. (2003). Enzyme activity was fluorometrically measured using 4-methylumbelliferyl-β-d-glucuronide as the substrate, and the reaction product, namely, 4-methylumbelliferone (MU), was detected. The protein concentration was determined using a Bio-Rad Protein Assay Kit (Bio-Rad Laboratories K.K., Tokyo, Japan).
Histochemical analysis
Histochemical analysis was conducted according to the method of CitationJefferson et al. (1987) modified by CitationKosugi et al. (1991). Fragments of the leaf or root were embedded in 4% agar and then cut into 100-µm sections using a DTK-100 microslicer (Dosaka EM, Kyoto, Japan). The leaves, roots or sections of the transgenic tobacco were incubated at 37°C for 3 h or overnight in the GUS reaction buffer, which was composed of 1 mmol L−1 5-bromo-5-chloro-3-indolyl-β-d-glucuronide, 3 mmol L−1 K5[Fe(CN)6], 0.5 mmol L−1 K3[Fe(CN)6], 50 mmol L−1 sodium phosphate buffer (pH 7.0) and 20% (v/v) methanol. After staining, the plantlets were washed in 70% ethanol to remove the chlorophyll. The GUS staining was then observed using a BZ-8000 microscope (Keyence, Osaka, Japan).
RESULTS AND DISCUSSION
Different expression patterns of OsNAS1pro::GUS and HvNAS1pro::GUS in transgenic tobacco plants after the split-root treatment
We carried out a split-root experiment to investigate the effect of systemic and local Fe signals on regulation of the HvNAS1 and OsNAS1 promoters in tobacco (). First, we verified the consistency of the split-root experiment using northern blot analysis with the NtFRO probe, which is a fragment of the tobacco FRO gene (CitationIto et al. 2007). The image obtained from northern blot analysis of non-transformant roots is shown in . NtFRO mRNA accumulation was strongly induced in the roots of –/– plants 7 days after the treatment, and in the +/– plants it was weakly induced in Fe-supplied split roots, but not in Fe-starved split roots. These results were consistent with the expression patterns of AtFRO2 and AtIRT1 in Arabidopsis (CitationVert et al. 2003). Ferric reductase activities in cucumber and tomato were also induced in the Fe-supplied region of split roots, when the other region of the root was subjected to Fe starvation (CitationLi et al. 2000; CitationSchikora and Schmidt 2001). Thus, the regulation of Fe-acquisition-related genes by systemic and local signals is generally observed in higher plants, such as tobacco.
The GUS activities of the transgenic tobacco roots subjected to the split-root experiment were measured (). GUS activity of HvNAS1pro::GUS was significantly induced in Fe-supplied split roots, but not in Fe-starved split roots in the +/– treated HvNAS1pro::GUS tobacco plants. This expression pattern was similar to that of NtFRO (). We observed that 3–7 days after the treatment, GUS activities were not induced in either Fe-supplied or Fe-starved split roots in the +/– treated OsNAS1pro::GUS tobacco. This expression pattern was different from that of NtFRO.
Models for the regulatory mechanisms of Fe-acquisition-related genes under conditions of Fe deficiency have been proposed: systemic signals of the Fe nutritional status are transmitted from the shoot to the roots, following which Fe-acquisition-related gene expression is induced in the Fe-supplied roots (CitationEnomoto et al. 2007; CitationGrusak and Pezeshgi 1996; CitationVert et al. 2003). Our results suggest that in response to certain systemic signals and the Fe concentration of the rhizosphere, the HvNAS1 promoter from barley exhibited functions similar to those of Fe-acquisition-related genes in tobacco. In contrast, the OsNAS1 promoter in transgenic tobacco plants does not seem to respond to the Fe concentration of the rhizosphere.
Figure 3 Expression of OsNAS1promoter::GUS and HvNAS1promoter::GUS in transgenic plants after the split-root treatment. The GUS activities in the roots of the transgenic tobacco plants are shown (A) 3 days after the treatment, (B) 5 days after the treatment and (C) 7 days after the treatment. Os, OsNAS1promoter::GUS; Hv, HvNAS1promoter::GUS. The numbers indicate the relative ratio of GUS activity to that in the +/+ treated plants. MU, 4-methylumbelliferone.
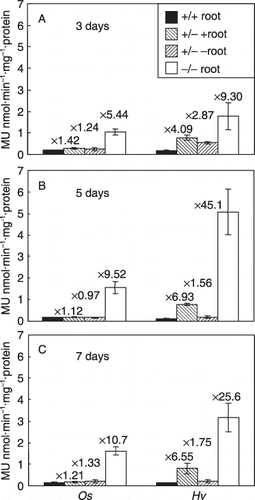
Localization of the GUS activity in transgenic tobacco
Histochemical staining was used to compare the tissue specificity of the promoter activity between the HvNAS1 pro::GUS and OsNAS1pro::GUS tobacco lines (). In our previous work, GUS activities were predominantly observed in the epidermis of Fe-deficient roots in HvNAS1 pro::GUS tobacco plants (CitationHiguchi et al. 2001a). In the present experiment, however, the cortex and endodermis of the Fe-deficient roots of HvNAS1pro::GUS tobacco were stained after incubation for 3 h (), and all the root cells of the section were stained after overnight incubation (data not shown). Although these results are partially inconsistent with those of our previous study (CitationHiguchi et al. 2001a), we discuss the results obtained in the present study with the T2 homozygous lines. GUS expression in the Fe-supplied split roots of the +/– treated HvNAS1pro::GUS tobacco was also dominant in the cortex (). GUS expression in the Fe-supplied split roots of the +/– treated HvNAS1pro::GUS tobacco and in the cortex and endodermis of its roots suggests that the HvNAS1 promoter might be regulated by mechanisms similar to those of genes related to Fe acquisition in tobacco roots.
The stele in the Fe-deficient roots of the OsNAS1 pro::GUS tobacco was stained after incubation for 3 h (), and all root cells in the section were stained after overnight incubation (data not shown). These results indicated that the OsNAS1 promoter did not drive GUS activity in response to the presence of Fe in the rhizosphere () and that it drove GUS activity in the stele of Fe-deficient roots; this suggests that the OsNAS1 promoter might be regulated by mechanisms similar to those of genes related to the long-distance transport of Fe in transgenic tobacco. The fact that the expression of endogenous OsNAS1 is induced by Fe deficiency in the vascular bundle of the rice leaf suggests the involvement of OsNAS1 in the long-distance transport of Fe within the plant body (CitationInoue et al. 2003).
The leaves of the HvNAS1pro::GUS tobacco were hardly stained regardless of the Fe nutritional status (). This is consistent with data from our previous study (CitationIto et al. 2007). In contrast, the inter-vein region of the mature leaves of OsNAS1pro::GUS tobacco was stained and GUS expression was induced by Fe deficiency (), that is, mature leaves were predominantly stained compared with young leaves that required more Fe. In addition, GUS activity was predominantly observed in mesophyll cells (). These results suggest that OsNAS1 promoter activity might be regulated by the mechanism for the expression of Fe-retranslocation-related genes in transgenic tobacco. Although it is well known that the extent of remobilization of Fe is relatively low in higher plants, substantial retranslocation of Fe from old leaves to young leaves has been reported in certain plant species (CitationMaruyama et al. 2005; CitationUauy et al. 2006; CitationZhang et al. 1995). Under Fe-deficient conditions, certain active mechanisms for Fe retranslocation from old leaves to young leaves might function in tobacco, and OsNAS1 promoter activity might be regulated by such a mechanism. GUS activity in the stele of the Fe-deficient roots of OsNAS1pro::GUS tobacco might also suggest that OsNAS1 promoter activity might be regulated by some of the mechanisms that remobilize the Fe deposited in the vascular bundle.
Figure 4 Histochemical localization of GUS activities in OsNAS1promoter::GUS and HvNAS1promoter::GUS transgenic plants. Transformants were subjected to histochemical staining 7 days after the onset of the Fe-deficiency treatment. A and C were stained for 3 h and B, D–G were stained overnight. (A) Cross-section of Fe-deficient HvNAS1promoter::GUS roots, (B) cross-section of Fe-supplied split roots of the +/– treated HvNAS1promoter::GUS roots and (C) cross-section of Fe-deficient OsNAS1promoter::GUS roots. (D–F) The leaves are arranged by age from young (left) to old (right) leaves. (D) Fe-deficient HvNAS1promoter::GUS leaves, (E) OsNAS1promoter::GUS leaves with sufficient Fe, (F) Fe-deficient OsNAS1promoter::GUS leaves and (G) cross-section of Fe-deficient OsNAS1promoter::GUS leaves. ep, epidermis; me, mesophyll.
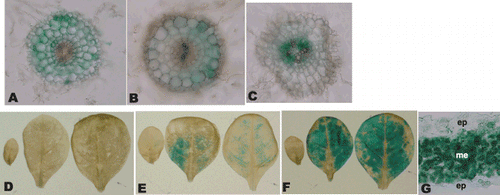
Conclusions
Unexpectedly, OsNAS1pro::GUS in transgenic tobacco drove the expression of GUS in a manner that was considerably different from HvNAS1pro::GUS in transgenic tobacco and endogenous OsNAS1 in rice, although it also induced expression in response to Fe deficiency. The expression pattern of OsNAS1pro::GUS in transgenic tobacco appears to mimic the pattern of some unknown Fe-retranslocation-related genes. Both universal components and a complicated cascade in the regulatory mechanisms for Fe-deficiency responses have been revealed in higher plants (CitationKobayashi et al. 2007). The OsNAS1 promoter might be unique in that it possesses numerous types of cis sequences involved in Fe metabolism.
Notes
Present address: †Plant Disease Resistance Research Unit, Division of Plant Sciences, National Institute of Agrobiological Sciences, 2-1-2 Kannondai, Tsukuba, Ibaraki 305-8602, Japan.
REFERENCES
- Colangelo , EP and Guerinot , ML . 2004 . The essential basic helix-loop-helix protein FIT1 is required for the iron deficiency response . Plant Cell , 16 : 3400 – 3412 .
- Curie , C , Panaviene , Z , Loulergue , C , Dellaporta , SL , Briat , JF and Walker , EL . 2001 . Maize yellow stripe 1 encodes a membrane protein directly involved in Fe(III) uptake . Nature , 409 : 346 – 349 .
- Enomoto , Y , Hodoshima , H , Shimada , H , Shoji , K , Yoshihara , T and Goto , F . 2007 . Long-distance signals positively regulate the expression of iron uptake genes in tobacco roots . Planta , 227 : 81 – 89 .
- Grusak , MA and Pezeshgi , S . 1996 . Shoot-to-root signal transmission regulates root Fe(III) reductase activity in the dgl mutant of pea . Plant Physiol , 110 : 329 – 334 .
- Higuchi , K , Suzuki , K , Nakanishi , H , Yamaguchi , H , Nishizawa , NK and Mori , S . 1999 . Cloning of nicotianamine synthase genes, novel genes involved in the biosynthesis of phytosiderophores . Plant Physiol , 119 : 471 – 479 .
- Higuchi , K , Tani , M , Nakanishi , H , Yoshihara , T , Goto , F , Nishizawa , NK and Mori , S . 2001a . The expression of a barley HvNAS1 nicotianamine synthase gene promoter-gus fusion gene in transgenic tobacco is induced by Fe-deficiency in roots . Biosci. Biotechnol. Biochem , 65 : 1692 – 1696 .
- Higuchi , K , Watanabe , S , Takahashi , M , Kawasaki , S , Nakanishi , H , Nishizawa , NK and Mori , S . 2001b . Nicotianamine synthase gene expression differs in barley and rice under Fe-deficient conditions . Plant J , 25 : 159 – 168 .
- Inoue , H , Higuchi , K , Takahashi , M , Nakanishi , H , Mori , S and Nishizawa , NK . 2003 . Three rice nicotianamine synthase genes, OsNAS1, OsNAS2, and OsNAS3are expressed in cells involved in long-distance transport of iron and differentially regulated by iron . Plant J , 36 : 366 – 381 .
- Ishimaru , Y , Suzuki , M Tsukamoto , T . 2006 . Rice plants take up iron as an Fe3+-phytosiderophore and as Fe2+ . Plant J , 45 : 335 – 346 .
- Ito , S , Inoue , H , Kobayashi , T , Yoshiba , M , Mori , S , Nishizawa , NK and Higuchi , K . 2007 . Interspecies compatibility of NAS1 gene promoters . Plant Physiol. Biochem , 45 : 270 – 276 .
- Jakoby , M , Wang , HY , Reidt , W , Weisshaar , B and Bauer , P . 2004 . FRU (BHLH029) is required for induction of iron mobilization genes in Arabidopsis thaliana . FEBS Lett , 577 : 528 – 534 .
- Jefferson , RA , Kavanagh , TA and Bevan , MW . 1987 . GUS fusions: -glucuronidase as a sensitive and versatile gene fusion marker in higher plants . EMBO J , 6 : 3901 – 3907 .
- Kobayashi , T , Nakayama , Y , Itai , RN , Nakanishi , H , Yoshihara , T , Mori , S and Nishizawa , NK . 2003 . Identification of novel cis-acting elements, IDE1 and IDE2, of the barley IDS2 gene promoter conferring iron-deficiency-inducible, root-specific expression in heterogeneous tobacco plants . Plant J , 36 : 780 – 793 .
- Kobayashi , T , Ogo , Y , Itai , RN , Nakanishi , H , Takahashi , M , Mori , S and Nishizawa , NK . 2007 . The transcription factor IDEF1 regulates the response to and tolerance of iron deficiency in plants . Proc. Natl Acad. Sci. USA , 104 : 19150 – 19155 .
- Kosugi , S , Suzuka , I , Ohashi , Y , Murakami , T and Arai , Y . 1991 . Upstream sequences of rice proliferating cell nuclear antigen (PCNA) gene mediate expression of PCNA-GUS chimeric gene in meristems of transgenic tobacco plants . Nucleic Acids Res , 19 : 1571 – 1576 .
- Li , CJ , Zhu , XP and Zhang , FS . 2000 . Role of shoot in regulation of iron deficiency responses in cucumber and bean plants . J. Plant Nutr , 23 : 1809 – 1818 .
- Ling , HQ , Bauer , P , Bereczky , Z , Keller , B and Ganal , M . 2002 . The tomato Fer gene encoding a bHLH protein controls iron-uptake responses in roots . Proc. Natl Acad. Sci. USA , 99 : 13938 – 13943 .
- Marschner , H . 1995 . Mineral Nutrition of Higher Plants , 2nd edn , London : Academic Press .
- Maruyama , T , Higuchi , K , Yoshiba , M and Tadano , T . 2005 . Comparison of iron availability in leaves of barley and rice . Soil Sci. Plant Nutr , 51 : 1035 – 1042 .
- Ogo , Y , Itai , RN , Nakanishi , H , Inoue , H , Kobayashi , T , Suzuki , M , Takahashi , M , Mori , S and Nishizawa , NK . 2006 . Isolation and characterization of IRO2, a novel iron-regulated bHLH transcription factor in graminaceous plants . J. Exp. Bot , 57 : 2867 – 2878 .
- Ogo , Y , Itai , RN , Nakanishi , H , Kobayashi , T , Takahashi , M , Mori , S and Nishizawa , NK . 2007 . The rice bHLH protein OsIRO2 is an essential regulator of the genes involved in Fe uptake under Fe-deficient conditions . Plant J , 51 : 366 – 377 .
- Ogo , Y , Kobayashi , T , Itai , RN , Nakanishi , H , Kakei , Y , Takahashi , M , Toki , S , Mori , S and Nishizawa , NK . 2008 . A novel NAC transcription factor, IDEF2, that recognizes the iron deficiency-responsive element 2 regulates the genes involved in iron homeostasis in plants . J. Biol. Chem , 283 : 13407 – 13417 .
- Robinson , NJ , Procter , CM , Connolly , EL and Guerinot , ML . 1999 . A ferric-chelate reductase for iron uptake from soils . Nature , 397 : 694 – 697 .
- Schikora , A and Schmidt , W . 2001 . Iron stress-induced changes in root epidermal cell fate are regulated independently from physiological responses to low iron availability . Plant Physiol , 125 : 1679 – 1687 .
- Scholz , G , Becker , R , Pich , A and Stephan , UW . 1992 . Nicotianamine: a common constituent of strategies I and II of iron acquisition by plants: a review . J. Plant Nutr , 15 : 1647 – 1665 .
- Shojima , S , Nishizawa , NK , Fushiya , S , Nozoe , S , Irifune , T and Mori , S . 1990 . Biosynthesis of phytosiderophores: in vitro biosynthesis of 2′-deoxymugineic acid from l-methionine and nicotianamine . Plant Physiol , 93 : 1497 – 1503 .
- Uauy , C , Distelfeld , A , Fahima , T , Blechl , A and Dubcovsky , J . 2006 . A NAC gene regulating senescence improves grain protein, zinc, and iron content in wheat . Science , 314 : 1298 – 1301 .
- Vert , G , Grotz , N , Dédaldéchamp , F , Gaymard , F , Guerinot , ML , Briat , JF and Curie , C . 2002 . IRT1, an Arabidopsis transporter essential for iron uptake from the soil and for plant growth . Plant Cell , 14 : 1223 – 1233 .
- Vert , GA , Briat , JF and Curie , C . 2003 . Dual regulation of the Arabidopsis high-affinity root iron uptake system by local and long-distance signals . Plant Physiol , 132 : 796 – 804 .
- Waters , BM , Blevins , DG and Eide , DJ . 2002 . Characterization of FRO1, a pea ferric-chelate reductase involved in root iron acquisition . Plant Physiol , 129 : 85 – 94 .
- Zhang , C , Römheld , V and Marschner , H . 1995 . Retranslocation of iron from primary leaves of bean plants grown under iron deficiency . J. Plant Physiol , 146 : 268 – 272 .
- Present address: †Plant Disease Resistance Research Unit, Division of Plant Sciences, National Institute of Agrobiological Sciences, 2-1-2 Kannondai, Tsukuba, Ibaraki 305-8602, Japan.