Abstract
Radioisotope techniques are well known as methods for evaluating symplastic ion absorption in roots. In the present study, a new method for evaluating symplastic cadmium (Cd) absorption in plant roots was developed using the enriched isotopes 113Cd and 114Cd. Seedlings of Solanum melongena were exposed to an enriched isotope solution of 113Cd at 25°C for 30 min. The roots were excised from each seedling and were then immersed in a cold buffer solution without Cd at 2°C for 120 min to suppress the metabolic activity of the roots. Finally, the roots were treated with a cold buffer solution containing enriched stable isotope 114Cd at 2°C for 120 min, whereby the apoplastically bound 113Cd was desorbed. We tested the validity of our method for evaluating symplastic Cd in roots compared with the conventional method based on differences in the amount of Cd absorbed at 2°C and 25°C using unlabeled Cd. There was no difference in the symplastic Cd content of the roots between the two methods. These results indicate that it is possible to evaluate the symplastic Cd content in roots using the enriched isotopes 113Cd and 114Cd.
Key words:
INTRODUCTION
Intensive studies on the absorption mechanisms of various elements by plant roots have been conducted. It is well known that ion absorption in plant roots shows a saturated curve in kinetics experiments, indicating that a type of proteinaceous transporter mediates ion absorption (CitationEpstein and Hagen 1952). Plant physiologists examining ion absorption in plant roots have given much attention to ion transport via the symplast across the plasma membrane (CitationEpstein 1973). However, when ion absorption experiments were conducted, it was found that the apoplastically absorbed ions needed to be washed out of the apoplast to determine the symplastically absorbed ions across the plasma membrane or the determination of absorption is overestimated (CitationGlass 2007). Therefore, it is necessary to eliminate the apoplastically bound ions to evaluate the symplastically absorbed ion content in the roots. To evaluate symplastic cadmium (Cd) absorption in roots, two methods have generally been used in the past: (1) expose the plant material to Cd radioisotopes and subsequent desorption using unlabeled Cd in the root apoplast (CitationHart et al. 1998, Citation2002, Citation2006), (2) plant material is exposed to Cd radioisotopes under conditions at 2°C and 22°C (CitationZhao et al. 2002). These methods have merits and demerits.
Using a radioisotope method allows the researcher to evaluate unidirectional ion absorption in roots. However, there is a radioisotope administrative restriction and a restrictive radioisotope half time. In addition, CitationZhao et al.'s (2002) method requires different seedlings in the absorption experiment at the different temperatures (2°C and 22°C), which is twice the number required for the other method. An enriched stable isotope could be a useful tool to overcome the faults of these methods. First, there is no restriction on the use of an enriched stable isotope in tracer experiments. Second, similar to a radioisotope experiment, it is possible to evaluate simultaneously the symplastic Cd using the same seedlings. For analysis, inductively coupled plasma-mass spectroscopy (ICP-MS) has now become the preferred technique. Tracer experiments, using a stable isotope, are very similar to those using a radioisotope on Cd to analyze plant mechanisms.
An isotope tracer technique with 113Cd has been used in pot and field experiments (CitationKawasaki and Oda 2004; CitationKawasaki et al. 2004; CitationStürup et al. 2008). In addition, Cd hyperaccumulator plants have been grown hydroponically with a highly enriched 113Cd isotope to investigate the form of Cd in the leaves using 113Cd nuclear magnetic resonance (NMR) spectroscopy (CitationUeno et al. 2005). Thus, the 113Cd isotope will become a new tool to evaluate Cd behavior in plants. The purpose of the present study was to devise a new and alternative radioisotope technique to evaluate the amount of symplastic Cd in the roots of Solanum melongena cv. Senryou2 using enriched isotopes of 113Cd and 114Cd. Although 113Cd is a radioisotope with a half-life of 7.7 × 1015 year−1, 113Cd is considered to be a fairly stable isotope. The 113Cd isotope is a long-lived nuclide and there is no administrative restriction in Japan. In Japan, S. melongena (eggplant) is one crop species in which the Cd content is higher than the permissible limit (maximum concentration of 0.05 mg Cd kg−1 fresh weight in fruiting vegetables) defined by CitationCodex Alimentarius Commission (2005). CitationTakeda et al. (2007) reported that the Cd concentration in eggplant fruits could be drastically reduced by grafting with Solanum torvum rootstock. In addition, CitationArao et al. (2008) found that although the Cd concentration in the shoot of S. torvum was lower than that found in S. melongena, there was no difference in the Cd content in the roots of each of these species when grown in culture solution. This result suggests that differential symplastic Cd absorption in roots might account for the difference in Cd translocation to the stem of the rootstocks, and to the stem and leaves of the scions.
Therefore, it is important to elucidate the mechanisms of Cd absorption in the roots and the translocation from the roots to the shoots in these plants. The method proposed in the present study can help to elucidate the mechanisms of Cd absorption in plant species that have a Cd content higher than the permissible limit.
MATERIALS AND METHODS
Plant culture
Solanum melongena seeds were germinated and grown on moist pearlite for 14 days. Then, seedlings of S. melongena were grown on moist pearlite containing half-strength Enshi standard nutrient solution for 14 days. Forty seedlings were then transferred to 30 L containers containing Enshi standard nutrient solution and grown for another 14 days. The composition of the nutrient solution was as follows: 1.5 mmol L−1 Ca(NO3)2, 3.4 mmol L−1 KNO3, 1 mmol L−1 MgSO4, 1 mmol L−1 NH4H2PO4, 4.6 × 10−2 mmol L−1 H3BO3, 9.1 × 10−3 mmol L−1 MnSO4, 7.6 × 10−4 mmol L−1 ZnSO4, 3.1 × 10−4 mmol L−1 CuSO4, 1.0 × 10−4 mmol L−1 NaMoO4 and 5.3 × 10−2 mmol L−1 FeSO4. The nutrient solution was exchanged every 7 days with fresh solution. The pH of the nutrient solution was adjusted to 5.5 using 0.1 mol L−1 NaOH. After preculture for 14 days, uniformly sized seedlings were selected and used in the Cd absorption experiment. Growth conditions were controlled at 25°C under natural light with a relative humidity of 60–80%.
Absorption experiments
The enriched isotopes of 113Cd (106Cd, 0.16%; 108Cd, 0.135%; 110Cd, 0.81%; 111Cd, 2.53%; 112Cd, 2.61%; 113Cd, 93.29%; 114Cd, 0.46%; 116Cd, 0.01%) and 114Cd (106Cd, 0.05%; 108Cd, 0.05%; 110Cd, 0.05%; 111Cd, 0.05%; 112Cd, 0.05%; 113Cd, 5.6%; 114Cd, 93.6%; 116Cd, 0.8%) used in the present study were purchased from Isoflex (San Francisco, CA, USA) in metallic form and dissolved in diluted HNO3. The procedure for evaluating symplastic Cd absorption in the roots, using enriched isotopes 113Cd and 114Cd, is illustrated in . The roots of intact seedlings were rinsed in ultrapure water for 2 min and then exposed to a 500 mL 113Cd solution containing 0.5 mmol L−1 CaCl2 and 2 mmol L−1 2-morpholinoethanesulfonic acid monohydrate–Tris(hydroxymethyl)aminomethane (MES–Tris) (pH 6.0) at 25°C for 30 min (). The levels of 113Cd were 40 nmol or 400 nmol in the 113Cd treatment. The roots were excised from each seedling and immersed in a cold Cd-free buffer solution (2 mmol L−1 MES–Tris [pH 6.0], 0.5 mmol L−1 CaCl2) at 2°C for 120 min to suppress metabolically dependent symplastic absorption from the apoplast (). The apoplastic-bound 113Cd in the roots from each 113Cd treatment (40 or 400 nmol) was then desorbed by immersing the roots in the same cold buffer solution at 2°C containing a 50-fold concentration of 114Cd (2 or 20 µmol) for 120 min (). The excised roots were then rinsed in ultrapure water for 2 min. Harvested samples were dried in an oven at 75°C for 3 days until dry.
The validity of this method was confirmed by comparing our Cd absorption results with the Cd absorption results obtained at 25°C and 2°C using unlabeled CdCl2 reagent. The experimental procedure was as follows. The Cd-absorption experiments were conducted for 30 min using 500 mL solutions containing 2 mmol L−1 MES–Tris (pH 6.0), 0.5 mmol L−1 CaCl2 and different concentrations of Cd (40 or 400 nmol) at 25°C. After the absorption experiment, the excised roots from each seedling were rinsed with ultrapure water for 2 min. For the Cd-absorption experiment at 2°C, plants were transferred to an ice-cold pretreatment solution containing 2 mmol L−1 MES–Tris (pH 6.0) and 0.5 mmol L−1 CaCl2 for 120 min. The Cd-absorption experiment at 2°C was conducted for 30 min. In the unlabeled Cd-absorption experiment at different temperatures, the amount of Cd reportedly absorbed into roots at 2°C was estimated to be apoplastically bound Cd on the assumption that metabolically dependent absorption would be suppressed at low temperature. Therefore, the difference in the amount of Cd absorbed at 2°C and at 25°C represents symplastic Cd absorption depending on metabolic energy. All absorption experiments were replicated three times.
Determination of 113Cd, 114Cd and the Cd contents in the roots
Approximately 0.05–0.1 g of dried roots was transferred and digested in a 10 mL Teflon tube containing 3 mL HNO3. After digestion, the digested solution was diluted and 10 ng mL−1 of indium (In) was added to each diluted solution as an internal standard for 114Cd determination. For 113Cd determination, 10 ng mL−1 of tellurium (Te) was added as an internal standard. The concentrations of 113Cd and 114Cd in the digested solutions were determined by ICP-MS (ELAN DRC-e; Perkin Elmer SCIEX, Concord, ON, Canada). The concentrations of Cd in the digested solutions from the Cd-absorption experiment using unlabeled CdCl2 reagent were determined by ICP-atomic emission spectroscopy (VISTA-PRO; Varian, Palo Alto, CA, USA).
It is well known that MoO interferes spectroscopically in determining the concentration of Cd in ICP-MS analysis (CitationKimura et al. 2003; CitationMay and Wiedmeyer 1998). In addition, it has been shown that it is necessary to remove Mo from the digested solution to avoid spectroscopic interference by molybdenum oxides (CitationOda et al. 2004; CitationYada et al. 2004). Therefore, for the 113Cd and 114Cd count intensities, we monitored the spectroscopic interference of the molybdenum oxides (97Mo16O and 98Mo16O) detected in the 10 ng mL−1 Mo standard solution. The contribution rate of spectroscopic interference of the putative 97Mo16O and 98Mo16O for 113Cd and 114Cd contents was negligibly small in both treatments (40 and 400 nmol). Therefore, we considered that we could ignore spectroscopic interference of oxidative molybdenum in determining the 113Cd and 114Cd contents in the ICP-MS analysis.
In addition, the 114Cd signal was corrected for isobaric interference from 114Sn using the 117Sn signal. The Cd content in roots grown in basic solution without Cd was negligible. Therefore, contamination of Cd in the roots does not affect our measurements.
We confirmed the accuracy of this research method by digesting the samples following the standard reference material (NIST SRM-1571 [Orchard Leaf, Washington, DC, USA]). The determined accuracy of the elemental concentrations measured in the NIST SRM-1571 were within the confidence interval (0.11 ± 0.01 µg Cd g−1).
Figure 2 Total 114Cd content, total 113Cd content, 113Cd content derived from enriched 114Cd and symplastic 113Cd contents in roots in the absorption experiment using the enriched isotopes 114Cd and 113Cd. DW, dry weight. Values are mean ± standard deviation (n = 3). The 113Cd content derived from the enriched stable isotope 114Cd was calculated from the total 114Cd content in the roots and the composition rate of 113Cd in the enriched stable isotope of 114Cd. The symplastic 113Cd content was calculated by subtracting the 113Cd content derived from the enriched stable isotope 114Cd from the total 113Cd content in the roots.
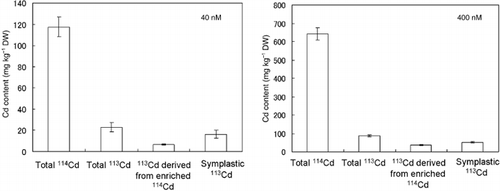
RESULTS AND DISCUSSION
Evaluation of symplastic Cd in roots using enriched isotopes of 113Cd and 114Cd
As shown in , the total 113Cd contents in the roots signifies the 113Cd contents in the roots after the desorption experiment (). The total 113Cd content in the roots at 40 and 400 nmol Cd was 23.0 ± 4.3 and 87.7 ± 5.6 mg kg−1 (dry weight), respectively (). In contrast, the 114Cd content at 40 and 400 nmol Cd was 117.3 ± 9.4 and 644.5 ± 33.7 mg kg−1 (dry weight), respectively (). The purification rate of the 114Cd-enriched stable isotope used in the present study was 93.60%; whereas, the composition rate of 113Cd in the 114Cd-enriched stable isotope was 5.6%. The total 114Cd content in the roots after desorption of 20 µmol 114Cd was approximately 5.5-fold higher than that using 2 µmol 114Cd (), suggesting that the apoplastically bound 113Cd content, derived from the enriched isotope 114Cd, increased with an increase in the concentration of 114Cd in the desorption solution. In actual fact, the apoplastically bound 113Cd contents, derived from the enriched isotope 114Cd (2 and 20 µmol) were 6.6 ± 0.5 and 36.6 ± 1.8 mg kg−1, respectively (); these values were calculated using EquationEq. 1. The contribution rate of 113Cd content derived from the enriched stable isotope of 114Cd for total 113Cd in the roots was 28.6% for the 40 nmol 113Cd treatment. In contrast, the contribution rate of 113Cd content derived from 114Cd for total 113Cd content in the roots was 41.8% for the 400 nmol 113Cd treatment (). These results indicate that the 113Cd derived from the enriched stable isotope of 114Cd must be subtracted from the total 113Cd content in the roots to evaluate the symplastic 113Cd in the roots. The symplastic 113Cd contents for the 40 and 400 nmol treatments, calculated using EquationEq. 2, were 16.4 ± 3.7 and 51.0 ± 3.8 mg kg−1, respectively ().
In the present study, we disregarded the contribution of 114Cd derived from the enriched isotope of 113Cd because the composition rate of 114Cd in the enriched isotope of 113Cd was considerably lower than that of 113Cd in the enriched isotope of 114Cd.
Comparison of the symplastic Cd contents in the roots between the two methods
To clarify the validity of the new method for evaluating the symplastic Cd content in roots using 113Cd and 114Cd enriched isotopes, we compared the symplastic Cd content in roots using differences in the amounts of Cd absorbed at 2°C and 25°C with unlabeled Cd with the results obtained in the present study using the new method. In conventional Cd-absorption experiments, the Cd contents in roots at 40 and 400 nmol Cd in a 25°C treatment were 19.2 ± 1.6 and 84.4 ± 3.4 mg kg−1 (dry weight), respectively (). In contrast, the Cd contents in roots at 40 and 400 nmol in the 2°C treatment were 4.1 ± 0.3 and 28.1 ± 0.73 mg kg−1 (dry weight), respectively. The symplastic Cd contents at 40 and 400 nmol were estimated to be 15.1 ± 1.3 and 56.4 ± 2.7 mg kg−1, respectively, which was evaluated using the difference in the amount of Cd absorbed at 2°C and at 25°C.
Figure 3 Comparison of the symplastic Cd content in the roots between the 113Cd-absorption experiment and the unlabeled Cd-absorption experiment. Values are mean ± standard deviation (n = 3).
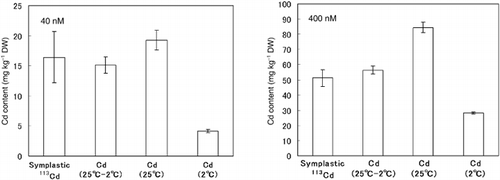
In the 113Cd-absorption experiment, the symplastic 113Cd contents in the roots following the 40 and 400 nmol 113Cd treatments were 16.4 ± 3.7 and 51.0 ± 3.8 mg kg−1, respectively (). Therefore, the symplastic 113Cd content after using the enriched isotopes was similar to the symplastic Cd content evaluated from the difference between the amount of Cd absorbed at 2°C and at 25°C. These results indicate that it is possible to evaluate the contents of symplastic Cd in roots using 113Cd and 114Cd enriched isotopes using the method proposed in the present study.
There have been many reports on Cd absorption in roots eliminating apoplastically bound Cd in Durum wheat, soybean and hyperaccumulator plants, such as Thlaspi caerulescens (CitationCataldo et al. 1983; CitationHart et al. 1998, Citation2002, Citation2006; CitationZhao et al. 2002). In these studies, the symplastic Cd content in the roots was determined by subtracting the Cd content in the roots at 2°C from the Cd content in the roots at 25°C; the Cd content was determined using a radioisotope of 109Cd or a metabolic inhibitor. These methods have frequently been used to evaluate nutrient element absorption in roots. Radioisotopes in solute were the most useful markers used in these studies because they are chemically similar to the solute and can be distinguished from non-labeled solutes already contained in the roots (CitationDavenport 2007). However, there are limitations to this method, including radioisotope administrative restriction and the restricted half-life of the radioisotope. Although the method involving a temperature difference between 2 and 25°C that was used in the present study is easy to handle because there is no radioisotope administrative restriction, there is, however, a limitation to this method: the symplastic Cd content in the roots cannot be evaluated using the same seedlings.
In the present study we have successfully used 113Cd and 114Cd enriched isotopes to detect symplastic Cd absorption in the roots of S. melongena. This method has the advantage of no radioisotope administrative restriction and no restrictive radioisotope half-lives. In addition, this method uses half the number of seedlings that are required for the method using the temperature difference between 2 and 25°C because the symplastically absorped Cd in the roots can be evaluated using roots from the same seedlings. In addition, the method proposed in the present study is applicable to other plants, not only S. melongena.
In conclusion, we examined a new method for estimating symplastic Cd content in roots using 113Cd and 114Cd enriched isotopes. We demonstrated that it is possible to evaluate symplastic Cd in roots using 113Cd and 114Cd enriched isotopes. The proposed method will contribute to research on symplastic ion absorption in plant roots.
ACKNOWLEDGMENTS
This work was supported by the Program for the Promotion of Basic Research Activities for Innovative Biosciences (PROBRAIN). We would like to thank Emeritus Professor T. Tadano of Hokkaido University for invaluable comments on the manuscript.
REFERENCES
- Arao , T , Takeda , H and Nishihara , E . 2008 . Reduction of cadmium translocation from roots to shoots in eggplant (Solanum melongena) by grafting onto Solanum torvum rootstock . Soil Sci. Plant Nutr , 54 : 555 – 559 .
- Cataldo , DA , Garland , TR and Wildung , RE . 1983 . Cadmium uptake kinetics in intact soybean plants . Plant Physiol , 73 : 844 – 848 .
- Codex Alimentarius Commission . 4–9 July 2005 . “ Joint FAO/WHO Food Standards ProgrammeTwenty-eighth session ” . 4–9 July , Rome, Italy Report of the 37th session of the Codex Committee on Food Additives and Contaminants, 2005 25–29 April 2005. Para. 175, Appendix XXVI. The Hague, the Netherlands. Available at URL: http://www.codexalimentarius.net/web/reports.jsp]ALINORM 05/28/12
- Davenport , RJ . 2007 . “ Ion uptake by plant roots ” . In Plant Solute Transport , Edited by: Yeo , AR and Flowers , TJ . 193 – 213 . Oxford : Blackwell Publishing .
- Epstein , E . 1973 . Mechanisms of ion transport through plant cell membranes . Int. Rev. Cytol , 34 : 123 – 168 .
- Epstein , E and Hagen , CE . 1952 . A kinetics study of the absorption of alkali cations by barley roots . Plant Physiol , 27 : 457 – 474 .
- Glass , ADM . 2007 . “ The apoplast: a kinetic perspective ” . In The Apoplast of Higher Plants: Compartment of Storage, Transport, and Reactions. The Significance of the Apoplast for the Mineral Nutrition of Higher Plants , Edited by: Sattelmacher , B and Horst , WJ . 87 – 96 . Dordrecht : Springer-Verlag .
- Hart , JJ , Welch , RM , Norvell , WA and Kochian , LV . 2002 . Transport interactions between cadmium and zinc in roots of bread and durum wheat seedlings . Physiol. Plant , 116 : 73 – 78 .
- Hart , JJ , Welch , RM , Norvell , WA and Kochian , LV . 2006 . Characterization of cadmium uptake, translocation and storage in near-isogenic lines of durum wheat that differ in grain cadmium concentration . New Phytol , 172 : 261 – 271 .
- Hart , JJ , Welch , RM , Norvell , WA , Sullivan , LA and Kochian , LV . 1998 . Characterization of cadmium binding, uptake, and translocation in intact seedlings of bread and durum wheat cultivars . Plant Physiol , 116 : 1413 – 1420 .
- Kawasaki , A and Oda , H . 2005 . Application of 113Cd as a tracer in evaluation of cadmium uptake by soybean under field conditions . Jpn. J. Soil Sci. Plant Nutr , 76 : 261 – 267 . (in Japanese with English summary)
- Kawasaki , A , Oda , H and Yamada , M . 2004 . Application of enriched 113Cd-tracer to the soil pot experiment of soybean plant . Jpn. J. Soil Sci. Plant Nutr , 75 : 667 – 672 . (in Japanese with English summary)
- Kimura , K , Yoshida , K , Sugito , T and Yamasaki , S . 2003 . Correction of interference by Mo oxide on Cd measurement by ICP-MS . Jpn. J. Soil Sci. Plant Nutr , 74 : 493 – 497 . (in Japanese with English summary)
- May , TW and Wiedmeryer , RH . 1998 . A table of polyatomic interferences in ICP-MS . At. Spectrosc , 19 : 150 – 154 .
- Oda , H , Yada , S and Kawasaki , A . 2004 . Uptake and transport of Cd supplied at different growth stages in hydroponically cultured soybean plants . Biomed. Res. Trace Elements , 15 : 289 – 291 . (in Japanese with English summary)
- Stürup , S , Hansen , HR and Gammelgaard , B . 2008 . Application of enriched stable isotopes as tracers in biological systems: a critical review . Anal. Bioanal. Chem , 390 : 541 – 554 .
- Takeda , H , Sato , A , Nishihara , E and Arao , T . 2007 . Reduction of cadmium concentration in eggplant (Solanum melongena) fruits by grafting with Solanum torvum rootstock . Jpn. J. Soil Sci. Plant Nutr , 78 : 581 – 586 . (in Japanese with English summary)
- Ueno , D , Ma , JF , Iwashita , T , Zhao , FJ and McGrath , SP . 2005 . Identification of the form of Cd in the leaves of a superior Cd-accumulating ecotype of Thlaspi caerulescens using 113Cd-NMR . Planta , 221 : 928 – 936 .
- Yada , S , Oda , H and Kawasaki , A . 2004 . Uptake and transport of Cd supplied at early growth stage in hydroponically cultured soybean plants . Biomed. Res. Trace Elements , 15 : 292 – 294 . (in Japanese with English summary)
- Zhao , FJ , Hamon , RE , Lombi , E , McLaughlin , MJ and McGrath , SP . 2002 . Characteristics of cadmium uptake in two contrasting ecotypes of the hyperaccumulator Thlaspi caerulescens . J. Exp. Bot , 53 : 535 – 543 .