Abstract
To better understand the behavior of metals in soil–plant systems, their physicochemical forms in rhizosphere soil should be elucidated. The dissolved organic matter (DOM) in a soil solution influences the mobility and bioavailability of metals in soil. The present study examined the effects of plant growth on DOM–metal complexes in a rhizosphere soil solution using size exclusion chromatography combined with an inductively coupled plasma-mass spectrometer system (SEC–ICP-MS) and an ultrafiltration technique. Humus-rich volcanic ash soil from the surface of an agricultural field was used in a pot cultivation experiment. Brassica rapa nothovar. was cultivated in a pot in which rhizosphere soil (R) was separated by a nylon net screen from non-rhizosphere soil (NR). Soil solutions were collected using a high-speed centrifugation method 3 weeks after sowing and analyzed using SEC–ICP-MS. Some peaks of DOM with a high molecular size were detected in the ultraviolet-absorbing chromatograph (280 nm) of the soil solution samples. Their concentrations were much higher in the R solution than in the NR solution. Metals including Al, Fe, Cu, Zn, Pb, Y, La and U were detected at the ultraviolet peak positions of the DOM. The ultrafiltration experiment showed that the size distributions of the organic materials to which the metals were combined differed between the R and NR soil solutions. These results suggest that plant growth enhanced the dissolution of metals adsorbed with organic matter from the solid phase in rhizosphere soil.
INTRODUCTION
Dissolved organic matter (DOM) is an important constituent of soil solution and plays an important role in many chemical and biological processes in soil. In particular, DOM regulates the bioavailability, toxicity and mobility of metals in soil solution because of its ability to complex with metals. To better understand the behavior of metals in soil–plant systems, the fate and role of DOM–metal complexes in soil needs to be elucidated. The rhizosphere is an extremely important microenvironment for the transport of metals from the soil to plants, and soil characteristics in the rhizosphere differ greatly from bulk soil because of plant–soil–microbial interactions (CitationHinsinger 2001; CitationLombi et al. 2001). A number of studies have shown that changes to the dissolved organic carbon (DOC) concentration are closely related to the bioavailability or speciation of metals in rhizosphere soil (CitationLegrand et al. 2005; CitationTao et al. 2005). However, there is little information about DOM–metal complexes in the rhizosphere and their behavior and status needs to be elucidated.
Size exclusion chromatography (SEC) has been widely used to estimate the molecular size of humic substances (CitationNobili and Chen 1999; CitationPiccolo et al. 2001). In recent metal speciation studies, inductively coupled plasma-mass spectrometry (ICP-MS) has been used in direct combination with SEC (CitationPiatina and Hering 2000), particularly for the determination of DOM–metal complexes in natural waters (CitationHaraguchi et al. 1995, Citation1998; CitationItoh et al. 2000; CitationVogl and Heumann 1997; CitationWu et al. 2004). The SEC–ICP-MS system has also been used for analyses of various materials, for example, for water-soluble boron compounds in radish roots (CitationMatsunaga et al. 1996), for iodine associated with humic and fulvic acid in soil (CitationYamada et al. 2002), for trace elements in citrate extracts from soil (CitationKimura 2004), in soil water (CitationCasartelli and Miekeley 2003) and in extracts from solid waste (CitationSadi et al. 2002), and for uranium (U) in a water extract from sediment (CitationJackson et al. 2005).
In a previous report, we showed that concentrations of DOC and U in a soil solution in the rhizosphere of Brassica rapa nothovar. increased with time during cultivation, implying that plant growth enhances U solubility in relation to DOM (CitationTakeda et al. 2008). In the present study, DOM–metal complexes in soil solution under plant growth conditions were observed using a SEC–ICP-MS system and an ultrafiltration technique to elucidate the influence of plant root activity on metal speciation in a rhizosphere soil solution.
MATERIALS AND METHODS
Soil and soil solution
The soil used in the present study was collected from the plow layer of an Andosol in Aomori Prefecture, Japan (40°51′N, 141°06′E). The collected soil was dried at 50°C and passed through a 2-mm sieve. The particle size distribution of the soil samples was determined using sieving and sedimentation methods. The water-holding capacity was determined using a Hilgard soil cup (Daiki Rika Kogyo, Kounosu, Japan). The pH (H2O) and pH (KCl) values were measured at a soil : water ratio of 1:2.5. The cation exchange capacity (CEC) of the soil samples was determined using the semi-micro Schollenberger method. Exchangeable cations (Ca, Mg, K and Na) were extracted by 1 mol L−1 ammonium acetate (pH 7) and determined using ICP atomic emission spectrometry (ICP-AES). Acid-oxalate-extractable Si (Sio), Al (Alo) and Fe (Feo) were determined using the methods described by CitationBlakemore et al. (1981). The soil samples were finely ground with an agate mortar and pestle. The total carbon and nitrogen contents in the soil were measured using a dry combustion method. The concentrations of metals in the soils were determined by ICP-AES or ICP-MS after decomposition with a mixed acid solution (HNO3, HF and HClO4) using a microwave digestion technique.
The physical and chemical properties are shown in . The soil was rich in poorly crystalline clay minerals and organic matter. An X-ray diffraction analysis showed the occurrence of 2:1–2:1:1 intergrade mineral, illite and kaolinite in the soil clay fraction. The soil had normal levels of metal concentrations without evident pollution in comparison with the average concentrations in Japanese soils (CitationTakeda et al. 2004).
Table 1 Soil properties and concentrations of the elements in the soil
Soil (400 g) was placed into a plastic pot (11-cm diameter and 7-cm high) after mixing with NH4NO3 and KH2PO4 (60 mg N pot−1; 17 mg P pot−1). The rhizosphere (R) soil in the pot was separated from the non-rhizosphere (NR) soil by a nylon mesh screen (with a mesh size of 20 µm) through which water, but not plant roots, could pass. The R compartment (upper part of the pot) and the NR compartment (bottom part of the pot) contained 80 g and 320 g of soil, respectively. The soil water content was adjusted to 70% of the water-holding capacity by adding deionized water 2 days before sowing and everyday after sowing. Brassica rapa nothovar. (komatsuna, Japanese mustard spinach) was used for the experiment. After five germinated seeds were sown into each pot, the soil surface in each pot was covered with polypropylene balls (5-mm diameter) to reduce water evaporation. The plants were cultivated in a controlled environmental growth chamber (20°C, 70% relative humidity, 12 h day length and 20,000 lx light intensity). Two seedlings from each pot were culled 6 days after sowing, leaving three plants in each pot. The pot experiments were conducted in duplicate.
At 0 days and 21 days after sowing, the R and NR soils were collected from two pots 4 h after adjusting the water content in the soil. Most plant roots were removed from the R soil. The soil solution was collected immediately after soil sampling using a high-speed centrifuge (H-1400pF; Kokusan Corporation, Tokyo, Japan) up to –1.5 M Pa (10,000 g, 90 min, 20°C) and filtered through a membrane filter (pore size 0.45 µm). The R and NR soil solutions obtained from two soils were mixed to obtain composite samples.
An ultrafiltration technique was used to acquire a rough size distribution of elements in the soil solution. The R and NR soils were collected from three different pots 26 days after sowing, and the soil solutions were collected from the soils immediately by centrifugation (as outlined above). The soil solutions were filtered through a 0.45-µm pore size membrane filter and a portion of the solution was filtered using a centrifugal filter device (Vivaspin 20; Sartorius AG, Goettingen, Germany) with a cutoff molecular weight of 10,000 Da. The concentrations of metals in the soil solution with and without the ultrafiltration process were determined.
Analytical method
The soil solution samples collected from the R and NR soil 21 days after sowing were analyzed using SEC–ICP-MS. The SEC–ICP-MS analysis used in the present study was based on a method developed for lake water (CitationTakaku et al. 2003). The LC/ICP-MS system consisted of a high-performance liquid chromatograph system (LC1100 LC system; Agilent Technologies, Santa Clara, CA, USA) combined with an ICP-MS instrument (VG-PQ-Excel; Thermo Elemental, Winsford, UK). The organic materials were measured at an absorbance of 280 nm ultraviolet (UV) using an UV/Vis absorption detector. The UV absorbance at 280 nm is commonly used to estimate the apparent molecular size and aromaticity of DOM (CitationChin et al. 1994; CitationKalbits et al. 2003). A gel permeation column measuring 10.7 mm internal diameter and 300 mm length (GL-W540; Hitachi Chemical, Tokyo, Japan) was used as an SEC column. A buffer solution of 0.01 mol L−1 Tris–HNO3 (pH 7.3) was selected as the mobile phase. The sample volume injected into the column was 100 µL, and the flow rate was 1.0 mL min−1. The molecular weights were calibrated using a Gel Filtration Standard (Bio-Rad Laboratories, Hercules, CA, USA), which was a lyophilized mixture of molecular weight markers, including thyroglobulin (670,000 Da), γ-globulin (158,000 Da), ovalbumin (44,000 Da), myoglobin (17,000 Da) and vitamin B-12 (1,350 Da). Metals that might be environmental pollutants (Cu, Zn, Cd, Pb and U) and metals with a high ability to complex with organic ligands (Y and La) were selected for determination, along with metals that were abundant in the soil (Al, Fe and Mn). Signals of 27Al, 55Mn, 65Cu, 66Zn, 89Y, 114Cd, 139La, 208Pb and 238U in the eluate from the UV detector were monitored using ICP-MS. Collision cell technology with helium gas was used to reduce the spectral interference resulting from 40Ar16O for 56Fe measurements in the ICP-MS. The time lag between the UV and ICP-MS detection was corrected for by using the signal of 59Co in the vitamin B-12.
The concentrations of the elements in the soil solution before and after the ultrafiltration treatment were measured using ICP-MS and ICP-AES. The DOC in the soil solution was measured using a total organic carbon analyzer (TOC-5000; Shimadzu, Kyoto, Japan).
RESULTS AND DISCUSSION
The soil solution pH at the beginning of the experiment was 5.8, resembling that in the NR soil solution after 21 days. In contrast, the soil solution pH in the R soil was significantly higher than that in the NR soil (). The pH change in the rhizosphere compensates for the imbalance of anions and cations taken up by the plant roots (CitationHinsinger et al. 2003; CitationNye 1981). The electrical conductivity in the soil solution collected from the R soil was much lower than that in the NR soil, probably because of nutrient uptake (). The DOC concentration in the soil solution at the beginning of the experiment was high, and decreased with time, particularly in the NR soil solution because the DOC was readily available to microorganisms (CitationCook and Allin 1992; CitationMarschner and Bredow 2002). The concentration of DOC in the R soil solution was approximately threefold higher than that in the NR soil solution, indicating that root activity elevates the DOC in the rhizosphere. Plant roots release various organic substances, including both high and low molecular weight compounds, which can increase the DOC in the rhizosphere soil solution. In the experimental pot, the root compartment is mixed with the soil. Therefore, both root exudate and soil compounds might have contributed to the increase in DOC (CitationWenzel et al. 2001). In addition, the root hairs cannot be completely removed from the R soil before the collection of the soil solution, and this can cause disruption to the roots during the centrifugation process and root sap might have contaminated the solution samples (CitationLorenz et al. 1994). In this experiment, the R soil was placed in the upper part of the pot. Therefore, differences in the results between the R and the NR soils might be caused by a position effect as well as a plant effect. However, the water content in the soils was less than 70% of the maximum water-holding capacity during the experimental period. The plastic balls on the soil surface prevented the influence of water evaporation and exposure to light. Therefore, the results obtained in the experiment strongly reflect a plant effect rather than a position effect.
Table 2 pH, electrical conductivity and concentrations of the elements in the soil solution (< 0.45 µm)
The concentrations of the metals in the soil solution are presented in . The concentrations in the R soil solution were higher than those in the NR soil solution for some metals, such as Fe, U, Al, Y and La. In particular, the Fe concentration in the R soil solution was more than 20-fold higher than that in the NR soil solution.
The results of the UV absorbance in the soil solutions are shown in . In both the R and the NR soil solutions, three broad UV-absorption peaks were observed over a retention time of 16–20 min, which corresponded to molecular weights of approximately 5–30 kDa. The peaks for the R soil solution were higher than those for the NR soil solution. A small peak was observed only in the R soil solution at a retention time of approximately 11 min, which would be larger than the exclusion limit of the column. The results suggest that plant roots enhance the dissolution of high molecular weight organic substances into the rhizosphere soil solution. Plant roots release low molecular weight organic acids into the rhizosphere soil. Some Brassica plants exude considerable amounts of organic acids, such as citric acid (CitationChiang et al. 2005; CitationGerke and Meyer 1995; CitationHoffland et al. 1989; CitationJones 1998; CitationZhang et al. 1997). The UV-absorbing organic substances in the soil solutions are considered to be humic substances (CitationAoyama 1996; CitationBerdén and Berggren 1990), mainly fulvic acid (CitationAoyama 2002). Humic substances are modified by the organic acids of the root exudates (CitationAlbuzio and Ferrari 1989). Therefore, the low molecular weight organic acids released from the root might solubilize the humic substances in the R soil.
The signal intensities detected by ICP-MS are shown in . In both the R and NR soil solutions, Al and Fe were detected at 16–20 min, which corresponds to UV-absorbing organic substances. The peaks of Al and Fe in the R soil solution were much higher than those in the NR soil solution. These results suggest that Al and Fe were dissolved from the soil solid phase as forms of DOM complexes. Humic substances are known to be solubilized by citrate, and humic–Al and humic–Fe complexes are important species in the presence of humic substances and citrate (CitationGerke 1993, Citation1997).
Figure 1 Size exclusion chromatography–inductively coupled plasma-mass spectrometry (SEC–ICP-MS) chromatograms of the soil solution samples. (A) Ultraviolet absorbance and (B) ICP-MS detection of the metals. The arrows indicate the retention time for the molecular weight markers. R, rhizosphere soil solution; NR, non-rhizosphere soil solution.
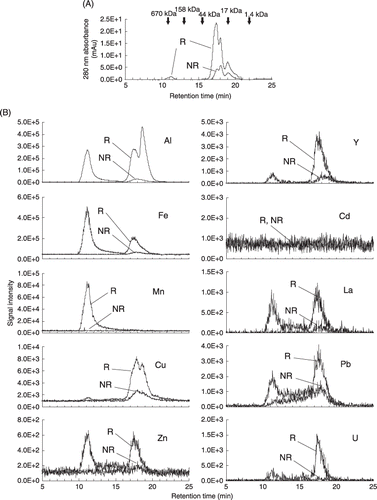
Figure 2 Percentages of metal concentrations in the low molecular weight fraction (< 10 kDa) compared with the soil solution (< 0.45 µm). Lowercase letters indicate significant differences between R and NR in each metal using a two-sample t-test (P < 0.05). Error bars indicate standard deviation (n = 3). R, rhizosphere soil solution; NR, non-rhizosphere soil solution; n.d, not detected.
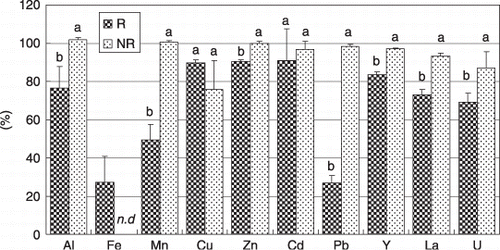
The exclusion limit for the SEC column was 700 kDa, and molecules or colloids larger than this size should elute in the void volume at 11 min. For both Al and Fe, a large peak was found only in the R soil solution at 11 min, and this might correspond to metals mainly in an inorganic colloidal form. The lower ionic strength in the R soil solution might enhance the dispersion of colloidal substances. Free metal cations or complexes with low molecular weight organic acids less than the effective sizable range of the SEC column (approximately 1 kDa) did not elute and were presumably bound to anionic surface groups of the gel phase (CitationJackson et al. 2005).
Results for the other trace metals are presented in . The chromatograms observed for Cu, Zn, Y, La, Pb and U resemble those for Al and Fe. These metals would also be mobilized as DOM complexes and/or colloidal forms in the rhizosphere soil. Using SEC–ICP-MS, similar results have been found for metals associated with DOM in natural water samples (CitationCasartelli and Miekeley 2003; CitationHaraguchi et al. 1995, Citation1998; CitationItoh et al. 2000; CitationJackson et al. 2005; CitationTakaku et al. 2003; CitationWu et al. 2004). In contrast, Mn was only found in the fraction at the exclusion limit in the R soil solution, indicating that Mn did not exist as a DOM-binding form in the soil solution, but was present as a colloidal form in the rhizosphere solution. No obvious peaks were observed for Cd in either the R or NR soil solution.
The concentrations of metals in the filtrate of the ultrafiltration with a cutoff molecular weight of 10 kDa were compared to those in the original soil solution (< 0.45 µm). The metal concentrations in the low molecular weight fraction (< 10 kDa) are shown in as percentages of those in the whole soil solution (< 0.45 µm). In the NR soil solution, the metal concentrations before and after ultrafiltration did not differ greatly. Therefore, the low molecular weight fraction occupied more than 90%, except for U (87%) and Cu (76%). These results indicated that the metals in the soil solution were mainly in smaller labile forms, including free ions or organic and inorganic complexes. In contrast, the percentages of metals in the low molecular weight fraction in the R soil solution were significantly lower than those in the NR soil solution for Al, Mn, Zn, Pb, Y, La and U. In other words, the percentages of the larger size fraction (0.45 µm–10 kDa) would be higher in the R solution than in the NR solution. The percentages of the low molecular weight fraction of metals in the R solution were in the following order: Cd, Zn, Cu and Y (> 80%); Al, La and U (80–60%); Mn (49%); Fe and Pb (27%). The results obtained in the filtration experiments were consistent with the observations obtained using SEC–ICP-MS (), and suggested that the high molecular weight DOM complexes or colloidal forms of the metals would be more abundant in the rhizosphere soil solution.
For Cd, free ion and labile complexes smaller than 10 kDa would be dominant in both the R and NR soil solution, according to the SEC–ICP-MS and ultrafiltration analysis (, ). Although a higher concentration of a DOM–Cu complex in the R solution was found in the SEC–ICP-MS analysis (), significant differences were not found for the concentration and size distribution of the DOM–Cu complex between the R and the NR solutions (; ). It is important to note that the SEC and ultrafiltration techniques sometimes yield different results for molecular weight estimations, possibly because of filtration effect artifacts during the ultrafiltration process (CitationZsolnay 2003), non-size exclusion effects (CitationPerminova 1999) and dissociation of metal complexes during chromatographic separation (CitationPiatina and Hering 2000).
The results obtained in the present study suggest that root activities can influence not only the concentrations of metals, but also the size distribution of their complexes with DOM in the rhizosphere soil solution. Many studies have examined the relationship between root exudation and metal mobilization in the rhizosphere, particularly in relation to the chelation of metals by organic acids (CitationLombi et al. 2001). In contrast, low molecular weight organic acids, such as citrate, can break bridges between Fe or Al and humic molecules by complexing the metals (CitationGerke 1992). Therefore, Al, Fe and other trace metals that are strongly associated with humic substances might be released to the soil solution as forms of DOM–metal complexes in the rhizosphere. The partitioning of metals between humic substances and organic acids should depend on the concentrations and species of the organic acids. CitationKimura (2004) reported that most transitional metals in 0.01 mol L−1 citric acid extracts formed complexes with citric acid, and it is implied that these metals were desorbed from humic complexes by citric acid.
Although the separation of the R soil was operationally defined, the results show that the R and NR soils clearly differ with respect to the concentration and speciation of metals in the soil solution, and our results suggest that rhizosphere effects can modify the molecular size distribution of DOM–metal complexes in soil solution.
ACKNOWLEDGMENTS
The authors are grateful to Ms J. Kogawa and K. Taneichi (ZAX) for their assistance with our work. We also thank the analytical chemistry staff at the Environmental Research Center for their assistance with the elemental analysis and JGC Plantech Aomori for their assistance with plant cultivation in the environmentally controlled chamber. This work was carried out under contract with the Aomori Prefectural Government, Japan.
REFERENCES
- Albuzio , A and Ferrari , G . 1989 . Modulation of the molecular size of humic substances by organic acids of the root exudates . Plant Soil , 113 : 237 – 241 .
- Aoyama , M . 2002 . Characterization of water-soluble organic matter in soils by size-exclusion chromatography and fractionation with polyvinylpyrrolidone . Soil Sci. Plant Nutr , 48 : 475 – 481 .
- Aoyama , M . 1996 . Use of high performance size exclusion chromatography to monitor the dynamic of water-soluble organic substances during the decomposition of plant residues in soil . Soil Sci. Plant Nutr , 42 : 21 – 30 .
- Berdén , M and Berggren , D . 1990 . Gel filtration chromatography of humic substances in soil solutions using HPLC-determination of the molecular weight distribution . J. Soil Sci , 41 : 61 – 72 .
- Blakemore , LC , Searle , PL and Daly , BK . 1981 . Methods for chemical analysis of soils , New Zealand Soil Bureau Scientific Report 10A Wellington : Department of Scientific and Industrial Research .
- Casartelli , EA and Miekeley , N . 2003 . Determination of thorium and light rare-earth elements in soil water and its high molecular mass organic fractions by inductively coupled plasma mass spectrometry and on-line-coupled size-exclusion chromatography . Anal. Bioanal. Chem , 377 : 58 – 64 .
- Chiang , PN , Wang , MK , Wang , JJ and Chiu , C-Y . 2005 . Low-molecular-weight organic acid exudation of rape (Brassica campestris) roots in cesium-contaminated soils . Soil Sci , 170 : 726 – 733 .
- Chin , YP , Alken , G and O’Loughlin , E . 1994 . Molecular weight, polydispersity, and spectroscopic properties of aquatic humic substances . Environ. Sci. Technol , 28 : 1853 – 1858 .
- Cook , BD and Allen , DL . 1992 . Dissolved organic carbon in old field soils: total amounts as a measure for available resources for soil mineralization . Soil Biol. Biochem , 24 : 585 – 594 .
- Gerke , J . 1992 . Phosphate, aluminium and iron in the soil solution of three different soils in relation to varying concentrations of citric acid . Z. Pflanzenernähr. Bodenk , 155 : 339 – 343 .
- Gerke , J . 1993 . Solubilization of Fe (III) from humic–Fe complexes, humic/Fe-oxide mixtures and from poorly ordered Fe-oxide by organic acids – consequences for P adsorption . Z. Pflanzenernähr. Bodenk , 156 : 253 – 257 .
- Gerke , J . 1997 . Aluminum and iron (III) species in the soil solution including organic complexes with citrate and humic substances . Z. Pflanzenernähr. Bodenk , 160 : 427 – 432 .
- Gerke , J and Meyer , U . 1995 . Phosphate acquisition by red clover and black mustard on humic podzol . J. Plant Nutr , 18 : 2409 – 2429 .
- Haraguchi , H , Itoh , A and Kimata , C . 1995 . Chemical speciation of trace metals in natural water by ultrafiltration/size exclusion chromatography/UV absorption/ICP-MS . Anal. Sci. Technol , 8 : 405 – 410 .
- Haraguchi , H , Itoh , A , Kimata , C and Miwa , H . 1998 . Speciation of yttrium and lanthanides in natural water by inductively coupled plasma mass spectrometry after preconcentration by ultrafiltration and with chelating resin . Analyst , 123 : 773 – 778 .
- Hinsinger , P . 2001 . “ Bioavailability of trace elements as related to root-induced chemical changes in the rhizosphere ” . In Trace Elements in the Rhizosphere , Edited by: Gobran , GR , Wenzel , WW and Lombi , E . 25 – 41 . Boca Raton : CRC Press .
- Hinsinger , P , Plassard , D , Tand , C and Jaillard , B . 2003 . Origins of root-meditated pH changes in the rhizosphere and their responses to environmental constraints: A review . Plant Soil , 248 : 43 – 59 .
- Hoffland , E , Findenegg , GR and Nelemans , JA . 1989 . Solubilization of rock phosphate by rape, II: Local root exudation of organic acids as a response to P-starvation . Plant Soil , 113 : 161 – 165 .
- Itoh , A , Iwata , K , Chiba , K and Haraguchi , H . 2000 . Chemical stability of large organic molecule–metal complexes dissolved in natural water as studied by size exclusion chromatography/inductively coupled plasma mass spectrometry . Bull. Chem. Soc. Jpn , 73 : 121 – 127 .
- Jackson , BP , Ranville , JF , Bertsch , PM and Sowder , AG . 2005 . Characterization of colloidal and humic-bound Ni and U in the “dissolved” fraction of contaminated sediment extracts . Environ. Sci. Technol , 39 : 2478 – 2485 .
- Jones , DL . 1998 . Organic acids in the rhizosphere – a critical review . Plant Soil , 205 : 25 – 44 .
- Kalbitz , K , Schmerwitz , J , Schwesig , D and Matzner , E . 2003 . Biodegradation of soil-derived dissolved organic matter as related to its properties . Geoderma , 113 : 273 – 291 .
- Kimura , K . Speciation of metals bound to humic substances and citric acid in soil extracts by size exclusion chromatography/inductively coupled plasma mass spectrometry . Proc. of the 6th International Symposium on Plant–Soil Interactions at low pH, 1–5 August 2004 . Sendai, Japan. pp. 166 – 167 .
- Legrand , P , Turmel , MC , Sauvé , S and Courchesne , F . 2005 . “ Speciation and bioavailability of trace metals (Cd, Cu, Ni, Pb, Zn) in the rhizosphere of contaminated soils ” . In Biogeochemistry of Trace Elements in the Rhizosphere , Edited by: Huang , PM and Gobran , GR . 261 – 299 . Amsterdam : Elsevier B.V .
- Lombi , E , Wenzl , W , Gobran , GR and Adriano , DC . 2001 . “ Dependency of phytoavailability of metals on indigenous and induced rhizosphere processes: a review ” . In Trace Elements in the Rhizosphere , Edited by: Gobran , GR , Wenzel , WW and Lombi , E . 3 – 24 . Boca Raton : CRC Press .
- Lorenz , SE , Hamon , R and McGrath , SP . 1994 . Differences between soil solutions obtained from rhizosphere and non-rhizosphere soils by water displacement and soil centrifugation . Eur. J. Soil Sci , 45 : 431 – 438 .
- Marschner , B and Bredow , A . 2002 . Temperature effects on the release and ecologically relevant properties of dissolved organic carbon in sterilized and biologically active soils . Soil Biol. Biochem , 34 : 459 – 466 .
- Matsunaga , T , Ishii , T and Watanabe , H . 1996 . Speciation of water-soluble boron compounds in radish roots by size exclusion HPLC/ICP-MS . Anal. Sci , 12 : 673 – 675 .
- Nobili , MD and Chen , Y . 1999 . Size exclusion chromatography of humic substances: limits, perspectives and prospectives . Soil Sci , 164 : 825 – 833 .
- Nye , PH . 1981 . Changes of pH across the rhizosphere induced by roots . Plant Soil , 61 : 7 – 26 .
- Perminova , IV . 1999 . Size exclusion chromatography of humic substances: complexities of data interpretation attributable to non-size exclusion effects . Soil Sci , 164 : 834 – 840 .
- Piatina , TB and Hering , JG . 2000 . Direct quantification of metal-organic interactions by size-exclusion chromatography (SEC) and inductively coupled plasma mass spectrometry . J. Environ. Qual , 29 : 1839 – 1845 .
- Piccolo , A , Conte , P , Cozzolino , A and Spaccini , R . 2001 . “ Molecular sizes and association forces of humic substances in solution ” . In Humic Substances and Chemical Contaminants , Edited by: Clapp , CE , Hayes , MHB , Senesi , N , Bloom , PR and Jardine , PM . 89 – 118 . Madison : Soil Science Society of America .
- Sadi , BBM , Wrobel , K , Wrobel , K , Kannamkumarath , SS , Castillo , JR and Caruso , JA . 2002 . SEC-ICP-MS studies for elements binding to different molecular weight fractions of humic substances in compost extract obtained from urban solid waste . J. Environ. Monit , 4 : 1010 – 1016 .
- Takaku , Y , Kakiuchi , H , Ohtsuka , Y , Hisamatsu , S and Inaba , J . Development of speciation analytical methods for lanthanides and actinides in lake water . Proc. of the International Symposium on Radioecology and Environmental Dosimetry . October 22–24 2003 , Rokkasho, Aomori, Japan. Edited by: Inaba , J , Tsukada , H and Takeda , A . pp. 336 – 342 .
- Takeda , A , Kimura , K and Yamasaki , S . 2004 . Analysis of 57 elements in Japanese soils, with special reference to soil group and agricultural use . Geoderma , 119 : 291 – 307 .
- Takeda , A , Tsukada , H , Takaku , Y , Akata , N and Hisamatsu , S . 2008 . Plant induced changes in concentrations of caesium, strontium and uranium in soil solution with reference to major ions and dissolved organic matter . J. Environ. Radioactiv , 99 : 900 – 911 .
- Tao , S , Liu , WX , Chen , YJ , Cao , J , Li , BG and Xu , FL . 2005 . “ Fractionation and bioavailability of copper, cadmium and lead in rhizosphere soil ” . In Biogeochemistry of Trace Elements in the Rhizosphere , Edited by: Huang , PM and Gobran , GR . 313 – 336 . Amsterdam : Elsevier B.V .
- Vogl , J and Heumann , KG . 1997 . Determination of heavy metal complexes with humic substances by HPLC/ICP-MS coupling using an on-line isotope dilution technique . Fresenius J. Anal. Chem , 359 : 438 – 441 .
- Wenzel , WW , Wieshammer , G , Fitz , WJ and Puschenreiter , M . 2001 . Novel rhizobox design to assess rhizosphere characteristics at high spatial resolution . Plant Soil , 237 : 37 – 45 .
- Wu , F , Evans , D , Dillon , P and Schiff , S . 2004 . Molecular size distribution characteristics of the metal–DOM complexes in stream waters by high-performance size-exclusion chromatography (HPSEC) and high-resolution inductively coupled plasma mass spectrometry (ICP-MS) . J. Anal. At. Spectrom , 19 : 979 – 983 .
- Yamada , H , Hisamori , I and Yonebayashi , K . 2002 . Identification of organically bound iodine in soil humic substances by size exclusion chromatography/inductively coupled plasma mass spectrometry (SEC/ICP-MS) . Soil Sci. Plant Nutr , 48 : 379 – 385 .
- Zhang , FS , Ma , J and Cao , JP . 1997 . Phosphorus deficiency induces root exudation of low-molecular weight organic acids and utilization of sparingly soluble inorganic phosphates by radish (Raghaus sativus L.) and rape (Brassica napus L.) plants . Plant Soil , 196 : 261 – 264 .
- Zsolnay , Á . 2003 . Dissolved organic matter: artifacts, definitions, and functions . Geoderma , 113 : 187 – 209 .