Abstract
The present study aimed to elucidate ammonia (NH3) volatilization loss following surface incorporation (0–15 cm mixing depth) of nitrogen (N) fertilizer in an upland field of light-colored Andosol in central Japan. A dynamic chamber technique was used to measure the NH3 effluxes. Poultry manure, pelleted poultry manure, cattle manure, pelleted cattle manure and ammonium sulfate were used as N fertilizers for basal fertilization to a bare soil with surface incorporation. All three experiments in summer and autumn 2007 and in summer 2008 showed negligible NH3 volatilization losses following the application of all N fertilizers with the same application rate of 120 kg N ha−1 as total N; these negligible losses were primarily ascribed to chemical properties of the soil, that is, its high cation exchange capacity (283 mmolc kg−1 dry soil) and relatively low pH(H2O) (5.9). In addition, the surface incorporation, the very small ratio of ammoniacal N to total N for the manure, and the decrease in soil pH to ≤5.5 following applications of ammonium sulfate were also advantageous to the inhibition of NH3 volatilization loss from the field-applied N fertilizers.
Introduction
Anthropogenic emission of ammonia (NH3) is one of the main sources of airborne nitrogen (N). Agriculture is the largest source of atmospheric NH3 to which livestock waste and field-applied N fertilizer primarily contribute. For example, agriculture accounts for 90% of the total NH3 emissions in Europe (CitationErisman et al. 2008). Information regarding NH3 emission factors is necessary to assess the impacts of airborne NH3 emission, transportation and deposition. Application methods, weather and soil conditions, and fertilizer types strongly affect NH3 emission from field-applied N fertilizers (CitationHuijsmans et al. 2003). Therefore, the actual status of NH3 emission induced by fertilizer application should be elucidated for each region and country to derive representative emission factors of NH3.
Relevant studies of NH3 emission from field-applied N fertilizer have been increasing only recently in Japan. For example, CitationHayashi et al. (2006, Citation2008) reported NH3 volatilization losses following applications of urea to a paddy field, and CitationMatsunaka et al. (2008) showed NH3 volatilization losses following applications of cattle slurry to grassland. Using incubation experiments, CitationFueki et al. (2007) also reported the potential for NH3 emission from volcanic ash soils following applications of several N fertilizers. However, little information on the NH3 emission from Japanese upland fields is available. Volcanic ash soils cover approximately 50% of Japanese upland fields (CitationTakata et al. in press). Furthermore, it is known that physicochemical properties are largely different between volcanic ash soils and non-volcanic ash soils (CitationShoji et al. 1993), which leads to a possible difference in NH3 emissions. Thus, concrete data on NH3 emissions from upland fields of volcanic ash soils should be accumulated to establish NH3 emission factors.
Small experimental plots, such as lysimeters, are advantageous for simultaneous investigations of NH3 emissions under various treatments. However, a micrometeorological technique that gives relatively accurate fluxes, but requires a large area with homogeneous conditions (e.g. CitationFowler et al. 2001) is not applicable to such small plots. Instead, a dynamic chamber technique (e.g. CitationKissel et al. 1977) seems to be the best alternative. Therefore, the objectives of the present study were to use a dynamic chamber technique to measure NH3 effluxes from lysimeter plots and to elucidate the NH3 volatilization loss following basal fertilization in a Japanese upland field of volcanic ash soil. Specifically, NH3 volatilization loss from surface-incorporated composted manure and ammonium sulfate in an upland field of light-colored Andosol, a typical soil type in upland fields in central Japan, was investigated.
Materials and methods
Experimental field
The study site included lysimeter plots located at the National Institute for Agro-Environmental Sciences (NIAES), central Japan (36°01′N, 140°07′E); the site was originally established to study greenhouse gas emissions. The study site has been used to elucidate and evaluate carbon and nitrogen cycles in agroecosystems, including greenhouse gases and relevant substances. The present study forms part of this overall aim. Six lysimeter plots of upland fields were used in the present study. Each plot, 3 m × 3 m, was filled with light-colored volcanic ash soil, classified as Andosol (CitationFood and Agriculture Organization 1998). Soil from the plowed layer and the subsoil, both Andosols, was collected in March 1994 from depths of 0–15 cm at an upland field in Nishinarado and from depths of 50–100 cm at a construction field in Fukuoka, both in Tsukubamirai City, that is, the former Yawara Village. The subsoil was used to fill the lysimeter plots to a depth of 80 cm, and then 20 cm of plowed layer soil was filled onto the subsoil. Light-colored Andosols, the parent material of which is a mixture of sediment and volcanic ash or resediment of volcanic ash, are common soil types in central Japan. The main properties of the soil in the lysimeter plots are listed in ; the soil texture refers to the plowed layer and the other values refer to the topsoil (depth 0–5 cm). The soil pH values shown in are the mean values of six lysimeters just before summer cropping in 2007. The cation exchange capacity (CEC) and exchangeable cation values are the mean values of six lysimeters in November 2008. Soil samples to determine CEC and exchangeable cations were collected from depths of 0–5 cm in the topsoil at five points per lysimeter. A composite sample per lysimeter was then made and air-dried. The CEC and exchangeable cations were determined using the semi-micro Schollenberger method (CitationSchollenberger and Simon 1945).
Table 1 Main properties of the soil in the lysimeter plots
The lysimeter plots have been used in experiments since the summer of 1995. Cultivation experiments of carrot, barley, qing-geng-cai and spinach were conducted from summer cropping in 1995 to summer cropping in 2000. Magnesium lime was applied to correct the soil pH at the beginning of summer and autumn cropping in 1997 at a rate of 2,200 kg ha−1, and at the beginning of summer cropping in 1998 at a rate of 2,000 kg ha−1. The lysimeter plots were fallowed from July 2000 to May 2002. The cultivation history after May 2002 is shown in . The lysimeter plots were also fallowed from September 2002 to September 2005. Leaf vegetables were cultivated in recent years.
Cultivation and fertilizer application
The NH3 volatilization loss from field-applied N fertilizers was investigated during three experimental periods: summer cropping in 2007 (sum-07), autumn cropping in 2007 (aut-07) and summer cropping in 2008 (sum-08). Komatsuna (Brassica rapa L. var. perviridis) was cultivated during these experiments.
Three treatments of basal fertilizer were applied during each of the experimental periods, that is, applications of manure, pelleted manure and chemical fertilizer. Two lysimeter plots per treatment were used. Poultry manure (PM) and pelleted poultry manure (PP) without additional materials were used in sum-07, whereas cattle manure (CM) and pelleted cattle manure (CP) with sawdust and straw as bedding materials were used in aut-07 and sum-08. Poultry manure and PP were provided by Mie Prefecture Agricultural Research Institute, Japan, and CM and CP were provided by the National Agricultural Research Center for Kyushu Okinawa Region, Japan. The manure was well fermented (composted) and was not fresh farmyard manure. The manure and the pelleted manure had the same origin. The manure and the pelleted manure were special in terms of their low water content, which was a necessary condition in pelletizing. No problems were observed regarding changes in the composition of CM and CP between aut-07 and sum-08 because they were well dried. In contrast, the chemical fertilizer was a combination of ammonium sulfate (AS), potassium chloride or potassium bromide, and superphosphate.
Table 2 Cultivation history of the lysimeter plots
The dates of basal fertilization were 11 June 2007, 19 September 2007 and 27 May 2008 for sum-07, aut-07 and sum-08, respectively. These fertilizers were applied to the lysimeter plots by surface incorporation (0–15 cm mixing depth) to bare soil using a mini-tiller. The application rate was 120 kg N ha−1 as total N (T-N). The experiments were designed to apply the fertilizers at the same application rate as T-N. As a result, the application rate in the present study was low for the manures compared with the actual rate used in upland cropping in Japan. The main properties of the manure and the pelleted manure are shown in . The manure pH was determined from an extract solution obtained by adding 200 mL of deionized water to 50 g of fresh manure. The PM and PP were relatively low in carbon content, but rich in N content. Ammoniacal N (NH4-N) accounted for 6–11% of T-N. In contrast, CM and CP were rich in carbon content, obtained to an extent from plant materials, but were relatively low in N content. NH4-N accounted for 4–5% of T-N.
Table 3 Main properties of the manure and pelleted manure used in the present study
Row sowing of Komatsuna (12 rows per lysimeter) with a sufficient number of seeds for thinning was conducted by hand just after the basal fertilization. Immediate sowing after fertilization resulted in no failure to germinate because the manure was well fermented. Seedlings were thinned out two to three times until harvest. The mean yields of Komatsuna for the six plots were 1.7–1.8, 0.8–1.0 and 0.3–0.9 Mg dry matter ha−1 for sum-07, aut-07 and sum-08, respectively; the CM and CP plots in sum-08 had particularly low yields of 0.3–0.4 Mg dry matter ha−1. The low application rate of manure in the present study compared with the amount generally used in upland cropping seemed to trigger the low yields observed in sum-08. However, the growth stages of Komatsuna during the measurements of the NH3 efflux were seeds to small seedlings. Furthermore, the chambers were set at the lysimeter plots to avoid the rows of Komatsuna seeds. It is, therefore, hypothesized that the cultivation conditions of Komatsuna had little impact on the observed NH3 volatilization losses.
Field measurements
A dynamic chamber method, that is, an enclosure technique with forced ventilation, was adopted in the present study as the most appropriate method applicable to lysimeter plots with a limited area. A schematic view of the dynamic chamber system is shown in . The system was composed of two sampling lines, one to collect NH3 in the ambient air and the other to collect NH3 in the exhaust air of a dynamic chamber that contained both ambient and volatilized NH3. The two sampling lines were separated in sum-07 (); however, they had the same inlet to homogenize the ambient NH3 between the two sampling lines in aut-07 and sum-08 (). The rectangular, stainless steel chamber had two vents on each of the narrow sides, a base area of 400 cm2 and a volume of 4 L (excluding anterior and posterior spaces). The chamber used in aut-07 and sum-08 had an open–close top. The reason for the change in the designs of the dynamic chamber system, that is, the same inlet for the two sampling lines and the open–close top of the chamber, in and after aut-07 was to further reduce errors in measurement. A two-stage filter holder (NL-I or NL-O; NILU, Kjeller, Norway) was used as the dry NH3 collector. The first (upstream) stage removed dust and particles, including particulate ammonium, from the air using a polytetrafluoroethylene (PTFE) filter with a pore size of 0.8 μm (T080A047A; Advantec, Tokyo, Japan). The second (downstream) stage collected NH3 in the air using a cellulose filter (51A; Advantec) impregnated with phosphoric acid. The preparation method and the advantages of the dry NH3 collector were stated in CitationHayashi et al. (2006). Six chamber system sets were prepared and one set per lysimeter was used.
Figure 1 Schematic view of the dynamic chamber system used in the present study. (a) The chamber used in the experiment in summer 2007 (sum-07) and (b) the chamber used in the experiments in autumn 2007 (aut-07) and summer 2008 (sum-08). PTFE, polytetrafluoroethylene.
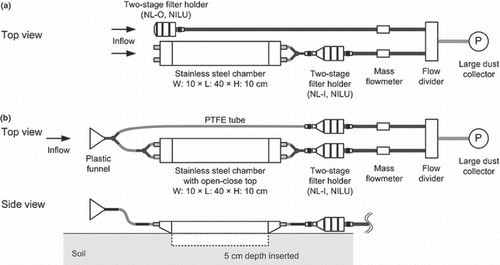
The NH3 emission was measured for 7 or 8 days following each fertilizer application. The exact time periods were 11–17 June 2007, 19–26 September 2007 and 27 May–2 June 2008 for sum-07, aut-07 and sum-08, respectively. The measurement frequency in each experiment was as follows: sum-07, three or four times per day with sampling times of 8.00–11.00 hours, 12.00–15.00 hours and 16.00–19.00 hours (and 20.00–23.00 hours when measurements were four times per day); aut-07, three times per day with sampling times of 8.00–11.00 hours, 12.00–15.00 hours and 16.00–19.00 hours; and sum-08, three times per day with sampling times of 8.30–10.30 hours, 12.00–14.00 hours and 15.30–17.30 hours.
The chamber was inserted into the soil to a depth of half its height (approximately 2 L internal volume) just after fertilization, avoiding the rows of Komatsuna seeds. In sum-07, the chamber was removed during the intervening periods and reinserted just before the next measurement. In contrast, in aut-07 and sum-08, an improved chamber with an open–close top was left in the soil after the installation. The top was closed during the measurements and left open during the intervening periods. The airflow rate in each sampling line was approximately 17 L min−1, which corresponded to an air exchange rate of approximately 8.5 exchange volume min−1 for the chamber, with a wind velocity within the chamber of approximately 0.06 m s−1. Except for the measuring times, rainwater was allowed to reach the soil surface where the chambers were located because the chambers were removed from the plots (sum-07) or the chamber tops were opened (aut-07 and sum-08). Although the chamber tops intercepted the rainwater during the measurements, the measuring times were slightly staggered to avoid heavy rain when forecasted.
After the samples were collected, the NH3 trapped on the phosphoric acid impregnated filter was extracted by adding deionized water. The concentration in the extracted solution was determined using a flow injection analyzer (AQLA-1000; Aqualab, Tokyo, Japan). The NH3 emission was expressed by.
The air temperature and relative humidity at a height of 0.1 m above the soil surface of lysimeter plot No. 3, corresponding to AS1 plot, were measured every 10 min using a sensor (HMP45A; Vaisala, Helsinki, Finland) and recorded on a datalogger (CR10X; Campbell Scientific, North Logan, UT, USA). Hourly rainfall data were obtained from the weather observatory of NIAES. Water-filled pore spaces (WFPS) of the soil at depths of 5 and 10 cm were measured at each lysimeter plot using time domain reflectometry (TDR) sensors (CS615; Campbell Scientific).
Soil sampling for chemical analysis was conducted every 2–3 days in principle. Five replicate surface soil (0–5 cm depth) samples were collected from each lysimeter and then merged into a composite sample. Fifteen grams of fresh soil was extracted by adding 100 mL of a 10% (w/v) potassium chloride solution under 1 h of shaking. The extracted solution was used to determine the NH4-N and nitrate N (NO3-N) contents of the soil using an auto analyzer (TRAACS2000; Bran + Luebbe, Norderstedt, Germany). Another portion of fresh soil was extracted by adding deionized water with a dry soil : water ratio of 1:5 (w/v) under 1 h of shaking. The pH of both solutions, the former as pH(KCl) and the latter as pH(H2O), was also measured.
Results
NH3 emissions
Overall, the measured NH3 fluxes showed very weak volatilization, indicating a rather negative value, that is, dry deposition, in some cases (). In sum-07, NH3 volatilization was clear only on the day of fertilization at one of the PM plots and at one of the PP plots and at 4–5 days after fertilization at the PP plots (). The maximum efflux was 0.46 μg N m−2 s−1 on the day of fertilization at one of the PM plots. The mean air temperature during sum-07 was 22.5°C. The soil received 15 mm of rainfall on the day before fertilization. The soil was moist during sum-07 and the WFPS was approximately 50%, excluding the direct effect of rain (). The rainfall 3–4 days after fertilization perhaps induced the delayed peak of NH3 volatilization at the PP plots. More specifically, chapping of the pellets as a result of swelling by the absorption of rainwater might have resulted in the volatilization of some of the NH4-N in the inner pellets.
In aut-07, NH3 volatilization was entirely negligible at the CM and CP plots. In contrast, very weak volatilization was found at the AS plots for several days following fertilization (). The mean air temperature during aut-07 was 24.0°C. The soil was dry during aut-07 and the WFPS was stable at approximately 30% (); there was no rain during aut-07 and for 1 week prior to the fertilization.
In sum-08, NH3 volatilization was also weak, although the emissions were slightly larger than those under the same treatments in aut-07 (). Weak volatilization was found at the CP and AS plots; however, no volatilization was observed at any of the plots on rainy days, that is, 2 and 4 days after fertilization (, ). In addition, the rain in the intervening time between measurements could fall onto and moisten the soil inside the chambers. The mean air temperature during aut-07 was 16.4°C. The temperature was particularly low 2–4 days after fertilization (). The soil was moist during sum-08 and the WFPS was approximately 40%, excluding the direct effect of rain ().
Figure 2 Ammonia emission following surface incorporation of poultry manure (PM), pelleted poultry manure (PP), cattle manure (CM), pelleted cattle manure (CP) and ammonium sulfate (AS). Arrows denote the fertilizer applications at a rate of 120 kg N ha−1 as total nitrogen. The numbers 1 and 2 refer to the respective lysimeter plots.
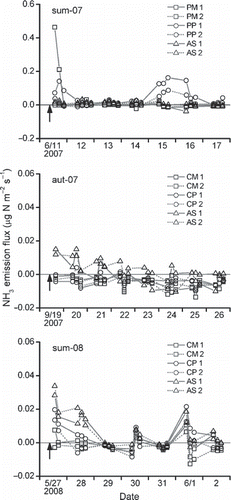
Cumulative NH3 volatilization loss
Reflecting the weak NH3 volatilization, the NH3 volatilization losses were very small for all of the treatments and experiments (). Losses to the applied NH4-N were only 0.7, 1.5, 0.0–0.1 and 0.0–0.3% for the PM, PP, CM and CP plots, respectively. In contrast, the AS plots showed no volatilization loss to the applied NH4-N. Therefore, the losses to the applied T-N were 0% for all treatments excluding the PP plots (0.2% of the applied T-N) ().
Table 4 Ammonia volatilization loss following surface incorporation (0–15 cm mixing depth) of the manure, pelleted manure and ammonium sulfate in the lysimeter plots of light-colored Andosol
Figure 4 Soil pH(H2O) and contents of ammoniacal nitrogen and nitrate nitrogen of the surface soil (depth 0–5 cm). Arrows denote the fertilizer applications at a rate of 120 kg N ha−1 as total nitrogen and the crossbars indicate the period of ammonia flux measurement. PM, poultry manure; PP, pelleted poultry manure; CM, cattle manure; CP, pelleted cattle manure; AS, ammonium sulfate.
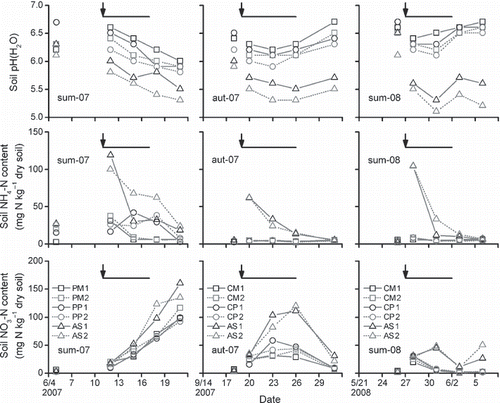
Changes in soil chemistry
Changes in the pH(H2O) and the contents of NH4-N and NO3-N of the surface soil (0–5 cm depth) around each of the experimental periods are shown in . In sum-07, the soil pH tended to decrease following fertilization at all plots; this tendency was particularly marked at the AS plots, with a decrease to ≤5.5 (). The increases in the NH4-N contents were less marked at the PM and PP plots, where the application rates of NH4-N were less than one-tenth of those at the AS plots (). However, the NH4-N contents at the PP plots slightly increased 5 days after fertilization, perhaps because of the release of NH4-N from the swollen pellets. In contrast, large increases in the NH4-N contents were found at the AS plots (), which could be ascribed to the rapid dissolution of AS owing to the moist soil and the relatively high temperature (). The decreases in pH were perhaps a result of nitrification in conjunction with a tendency toward large increases in the NO3-N contents at all plots ().
In aut-07, the decreases in pH were weak at the CM and CP plots, dropping to near 6 and then increasing. In contrast, the decreases in pH were marked at the AS plots, similar to those in sum-07. The NH4-N contents at the CM and CP plots remained flat because of the small application rates of NH4-N (). The relatively small increases in the NH4-N contents at the AS plots compared with those in sum-07 and sum-08 () perhaps resulted from the inhibition of the dissolution of AS owing to the dry conditions (). The NO3-N contents increased for 1 week following fertilization and then began to decrease ().
In sum-08, when the same treatments as those used in aut-07 were adopted, changes in the soil pH and soil inorganic N content showed similar tendencies to those observed in aut-07. However, increases in the NH4-N contents at the AS plots in sum-08 were larger than those in aut-07, which reflected the facilitation of the dissolution of AS as a result of the moist soil conditions in sum-08. The rainfall during sum-08 likely resulted in the small increases in the NO3-N content owing to NO3-N leaching from the surface soil by rainwater.
Discussion
Causes of the negligible volatilization loss
Adsorption of released NH4-N from N fertilizers onto soil particles inhibits NH3 volatilization. The present study supported this particularly clearly at the AS plots where no marked NH3 volatilization took place (), despite marked increases in NH4-N content following the application of AS (). A large CEC results in further adsorption of ammonium ions (NH4 +) onto soil particles. Incubation experiments using 22 types of soil in China with CEC values ranging from 63.6 to 254 mmolc kg−1 dry soil (CitationDuan and Xiao 2000) showed a negative correlation between CEC and NH3 volatilization (R = −0.568, P < 0.01). Furthermore, this relationship was well explained by an exponential function (R 2 = 0.564). According to the results of CitationDuan and Xiao (2000), a CEC above 130 mmolc kg−1 dry soil strongly inhibited NH3 volatilization. The CEC of light-colored Andosol in the present study, 283 mmolc kg−1 dry soil (), was larger than the maximum value of CEC, 254 mmolc kg−1 dry soil, in the study of CitationDuan and Xiao (2000). It is, therefore, concluded that the high CEC of the light-colored Andosol was the primary cause of the negligible NH3 volatilization losses observed in the present study. The low soil pH levels, which dropped to 5.0–5.5 following fertilization at the AS plots (), were also effective in inhibiting NH3 volatilization because a low pH moves the dissociation equilibrium of NH4 + in the liquid phase to a decrease in the fraction of aqueous NH3 (e.g. CitationHayashi et al. 2008). In addition, the marked decreases in pH at the AS plots could be ascribed to nitrification in conjunction with increases in soil NO3-N content (). Furthermore, dry conditions inhibit NH3 volatilization through a reduction in NH4-N release from N fertilizers (e.g. CitationKresge and Satchell 1960), which might, in part, have caused the result obtained in aut-07. In contrast, excessively moist conditions caused by rainfall or irrigation also inhibit NH3 volatilization through the effects of dilution and leaching (e.g. CitationWhitehead and Raistrick 1991), which might, in part, have caused the result obtained in sum-08. According to CitationKresge and Satchell (1960), an initial water content of 20%, that is, the approximate field water capacities of the test soils, resulted in the most enhanced NH3 volatilization loss following surface applications of urea.
NH3 volatilization originates directly from the NH4-N in the soil. Fermented manure and its pellets lose most of their NH4-N as a result of volatilization loss during fermentation. Therefore, fermented manure has a smaller potential for NH3 volatilization than fresh manure. For example, CitationMcGinn and Sommer (2007) reported that the NH3 volatilization losses following surface applications of fresh manure and compost of beef cattle were 5.7% and 0.2% of the applied T-N, respectively. According to CitationMcGinn and Sommer (2007), the very small loss of 0.2% of applied T-N resulted from the application of compost with a rate of 27.7 Mg fresh weight ha−1. Thus, it is inferred that changes in NH3 volatilization loss would be small if the low application rates of manure in the present study, corresponding to 2.4–5.2 Mg fresh weight ha−1, were increased to those of actual upland cropping (e.g. 10–20 Mg fresh weight ha−1).
Regarding the potential for NH3 emission from AS, the CitationEuropean Environment Agency (2007) suggested a range of emission factors, depending on climate, of 1.5–2.5% of applied T-N. However, these emission factors include emissions from both surface-applied fertilizer and crop foliage. The CitationEuropean Environment Agency (2007) further noted that a multiplier of 10 should be applied to the emission factors in soils with high pH levels, such as calcareous soils. In the present study, the soil pH was relatively low at 5.9 (). Surface incorporation was conducted instead of surface application, and foliage emission was not considered. It is, therefore, hypothesized that the potential for NH3 volatilization loss from the AS plots would be less than that stated by the CitationEuropean Environment Agency (2007).
The surface incorporation method of application has a strong effect on the inhibition of NH3 volatilization, although no direct comparison of NH3 volatilization loss between surface incorporation and surface application was conducted in the present study. For example, CitationHuijsmans et al. (2003) reported that the weighted means of NH3 volatilization loss from field-applied pig slurry by surface application (top-dressing), surface incorporation (mixing with the plowed layer) and deep placement (15–20 cm depth) were 68, 17 and 2% of the applied NH4-N, respectively. Thus, in the case of slurry, surface incorporation can reduce the NH3 volatilization loss to a quarter of that experienced with surface application. In contrast, manure has a lower potential for NH3 volatilization loss compared with slurry because a certain amount of NH3 has already been lost during the storage and/or fermentation of manure. Furthermore, deep placement is unpractical for solid manure. It is, however, inferred that surface incorporation is more effective to reduce NH3 volatilization loss than surface application (CitationHuijsmans et al. 2003), although its effect is weaker than the effect recorded for slurry.
A negative NH3 flux was occasionally found, particularly at the CM and CP plots (). Negative fluxes resulted from higher NH3 concentrations in the ambient air than in the soil air, which was ruled by the partition equilibrium of NH3 between the gas–liquid phases in the soil. As a result, the transfer of NH3 from the atmosphere to the soil, that is, dry deposition (absorption), took place instead of the inverse transfer, that is, volatilization. Dry deposition is strongly affected by micrometeorological factors (e.g. CitationAndersen and Hovmand 1999). The present study aimed to elucidate NH3 volatilization loss. Moreover, the dynamic chamber technique used in the present study was not suitable for measuring dry deposition, including micrometeorological effects, because the chamber covered the soil surface. Hence, negative fluxes were excluded from discussion in the present study.
The pH levels of upland soils, particularly those from volcanic ash, occasionally dip from the range suitable for cropping. In such cases, alkaline materials, such as lime and dolomite, are applied to adjust the pH level. However, an excessive application of these materials results in an unnecessarily high pH level, which could enhance NH3 volatilization loss. Furthermore, the applied N fertilizer, in the intervening time between application and incorporation, corresponds to the case of surface-applied fertilizer even if the surface incorporation method is conducted. The longer the time between application and incorporation, the more likely that NH3 volatilization will occur. However, the potential for NH3 volatilization loss with the use of manure is smaller than that with the use of slurries. Thereby, the difference in NH3 volatilization loss would be small for manure using the surface application and surface incorporation methods. In addition, the effects of successive applications of manure on NH3 volatilization loss were out of the scope of the present study because, in the experimental design, the application rates of manure as fresh weight were smaller than those used in actual upland cropping.
The atmosphere contains NH3. For example, the mean NH3 air concentration in the warm season on turf grassland near the study site was 2.7 μg N m−3 (0°C and 1013 hPa) (CitationHayashi et al. 2007). The NH3 concentrations in the ambient air in the present study ranged from 0.40 to 10.3 μg N m−3. The concentrations were high in the daytime and just after fertilization and low in the nighttime and on rainy days (data not shown). The partition equilibrium of NH3 between the gas–liquid phases rules the NH3 volatilization. Hence, the effect of ambient NH3 on NH3 efflux should be considered when evaluating NH3 volatilization loss in the environment. In contrast, CitationFueki et al. (2007) reported a small NH3 volatilization loss from surface-applied AS in incubation experiments using Andosols in Hokkaido, northern Japan. This relatively large volatilization loss, in contrast to the negligible loss obtained in the present study, could be ascribed to the surface application method. In addition, the NH3-free air that they used to ventilate the vessels appeared to increase volatilization loss to some extent because the NH3-free gas phase enhances NH3 efflux from the liquid phase. It is interpreted that incubation experiments using NH3-free air, such as CitationFueki et al. (2007), are used to elucidate potential NH3 volatilization loss.
Uncertainty of the dynamic chamber method
It is known that the ventilation rate within the chamber affects the NH3 emission. CitationKissel et al. (1977) reported that a ventilation rate of less than 15 exchange volume min−1 resulted in an underestimation of NH3 emission. According to CitationKissel et al. (1977), the ventilation rate of 8.5 exchange volume min−1 in the present study corresponded to a 14% underestimation of the fluxes. However, CitationHuijsmans et al. (2003) pointed out that the wind velocity in the actual environment affected the temporal patterns of NH3 emission, but rarely affected the cumulative NH3 flux, that is, the loss to the applied N. The present study also focused on the volatilization loss rather than on the respective fluxes. In addition, the clear reductions in the soil NH4-N content during the experimental periods () indicated that marked NH3 volatilization was unlikely to occur beyond the experimental periods. Hence, the derived negligible losses in the present study can be considered valid.
Conclusions
The NH3 volatilization loss from surface-incorporated manure and ammonium sulfate was investigated at an upland field of light-colored Andosol in central Japan. The three experimental periods, summer and autumn 2007 and summer 2008, showed negligible NH3 volatilization losses following applications of poultry manure, pelleted poultry manure, cattle manure, pelleted cattle manure and ammonium sulfate at the same application rate of 120 kg N ha−1 as T-N. Although the pelleted manure showed a delayed release of NH4-N to some extent, this delay in release did not affect the NH3 volatilization loss.
The high CEC of the light-colored Andosol used in the present study, 283 mmolc kg−1 dry soil, was primarily effective in inhibiting NH3 volatilization loss. The relatively low soil pH, 5.9 as pH(H2O), also reduced the NH3 volatilization loss. In particular, the marked decreases in soil pH to ≤5.5 following applications of ammonium sulfate effectively inhibited NH3 volatilization loss. The fermented manure (compost) used in the present study had a small fraction of NH4-N to T-N, which also contributed to the negligible NH3 volatilization loss. The NH3 efflux is positively and linearly proportional to the NH4-N concentration in soil solutions (CitationHayashi et al. 2008). Therefore, when the absolute amount of NH4-N applied to upland fields was small owing to the small fraction of NH4-N to T-N in manure, the NH3 volatilization loss was also small. It is also known that the application method used in the present study, that is, surface incorporation, is highly effective in inhibiting NH3 volatilization loss, although no direct comparison was conducted in the present study with the surface application method. Thus, it is necessary to steadily accumulate concrete data based on case studies, such as the present study, and then establish the NH3 emission factors of Japanese agriculture.
Acknowledgments
We would like to express our appreciation to Messrs Hiroshi Kamimura, Hironori Wakabayashi and Toshiyuki Okada, NIAES, for their practical help in managing the experimental field. We thank Dr Masaki Takeuchi, Mie Prefectural Agricultural Research Institute, for his provision of poultry manure and pelleted poultry manure, and Dr Yusuke Arakawa, National Agricultural Research Center for Kyushu Okinawa Region, for his provision of cattle manure and pelleted cattle manure. We also thank Dr Yasuhito Shirato, NIAES, for his assistance with soil analysis. Rainfall data were obtained from the Weather Data Acquisition System of NIAES. This study was supported in part by a Grant-in-Aid for Scientific Research (A), No.19201008, provided by the Ministry of Education, Culture, Sports, Science and Technology, Japan.
References
- Akiyama , H , Tsuruta , H and Watanabe , T . 2000 . N2O and NO emissions from soils after the application of different chemical fertilizers . Chemosphere Global Change Sci. , 2 : 313 – 320 .
- Andersen , HV and Hovmand , MF . 1999 . Review of dry deposition measurements of ammonia and nitric acid to forest . Forest Ecol. Manag. , 114 : 5 – 18 .
- Duan , Z and Xiao , H . 2000 . Effects of soil properties on ammonia volatilization . Soil Sci. Plant Nutr. , 46 : 845 – 852 .
- European Environment Agency . 2007 . EMEP/CORINAIR Emission Inventory Guidebook 2007 Available from URL: http://reports.eea.europa.eu/EMEPCORINAIR5/en/page002.html
- Erisman , JW , Bleeker , A , Hensen , A and Vermeulen , A . 2008 . Agricultural air quality in Europe and the future perspectives . Atmos. Environ. , 42 : 3209 – 3217 .
- Food and Agriculture Organization . 1998 . World Reference Base for Soil Resources. World Soil Resources Reports 84 , Rome : Food and Agriculture Organization .
- Fowler , D , Coyle , M , Flechard , C , Hargreaves , K , Nemitz , E , Streton-West , R , Sutton , MA and Erisman , JW . 2001 . Advances in micrometeorological methods for the measurement and interpretation of gas and particle nitrogen fluxes . Plant Soil , 228 : 117 – 129 .
- Fueki , N , Tani , M and Nakatsu , S . 2007 . Influence of soil pH, temperature and applied nitrogen forms on ammonia volatilization from Andosol . Jpn. J. Soil Sci. Plant Nutr. , 78 : 309 – 312 . (in Japanese)
- Hayashi , K , Nishimura , S and Yagi , K . 2006 . Ammonia volatilization from the surface of a Japanese paddy field during rice cultivation . Soil Sci. Plant Nutr. , 52 : 545 – 555 .
- Hayashi , K , Komada , M and Miyata , A . 2007 . Atmospheric deposition of reactive nitrogen on turf grassland in central Japan: Comparison of the contribution of wet and dry deposition . Water Air Soil Pollut. Focus , 7 : 119 – 129 .
- Hayashi , K , Nishimura , S and Yagi , K . 2008 . Ammonia volatilization from a paddy field following applications of urea: Rice plants are both an absorber and an emitter for atmospheric ammonia . Sci. Tot. Environ. , 390 : 486 – 495 .
- Huijsmans , JFM , Hol , JMG and Vermeulen , GD . 2003 . Effect of application method, manure characteristics, weather and field conditions on ammonia volatilization from manure applied to arable land . Atmos. Environ. , 37 : 3669 – 3680 .
- Kissel , DE , Brewer , HL and Arkin , GF . 1977 . Design and test of a field sampler for ammonia volatilization . Soil Sci. Soc. Am. J. , 41 : 1133 – 1138 .
- Kresge , CB and Satchell , DP . 1960 . Gaseous loss of ammonia from nitrogen fertilizers applied to soils . Agron. J. , 52 : 104 – 107 .
- Matsunaka , T , Sentoku , A , Mori , K and Satoh , S . 2008 . Ammonia volatilization factors following the surface application of dairy cattle slurry to grassland in Japan: Results from pot and field experiments . Soil Sci. Plant Nutr. , 54 : 627 – 637 .
- McGinn , SM and Sommer , SG . 2007 . Ammonia emissions from land-applied beef cattle manure . Can. J. Soil Sci. , 87 : 345 – 352 .
- Schollenberger , CJ and Simon , RH . 1945 . Determination of exchange capacity and exchangeable bases in soils . Soil Sci. , 59 : 13 – 24 .
- Shoji , S , Nanzyo , M and Dahlgren , RA . 1993 . Volcanic Ash Soils: Genesis, Properties, and Utilization , Amsterdam : Elsevier .
- Takata , Y , Nakai , M and Obara , H . Digital soil map of Japanese croplands in 1992 . Jpn. J. Soil Sci. Plant Nutr. , in press in Japanese
- Whitehead , DC and Raistrick , N . 1991 . Effects of some environmental factors on ammonia volatilization from simulated livestock urine applied to soil . Biol. Fertil. Soils , 11 : 279 – 284 .