Abstract
We developed a new and improved method, the ‘high-emission-incorporation (HEI) method’, for estimating soil nitrous oxide (N2O) emission rates at a watershed level based on nitrogen (N) input (consisting of fertilizer, manure, slurry and excreta N) and N surplus (calculated by subtracting the amount of crop yield and consumed N from the N input) of different sites in a livestock farm located in a watershed. The main characteristic of this method is the inclusion of extremely high N2O emission rates, ‘outlier’, which are normally excluded from estimation. High N2O emission rates were estimated using the regression model obtained from the measured N2O values and the amounts of N surplus; normal N2O emission rates were estimated using the regression model obtained from the measured values and the amount of N input. The probability of occurrence of a high flux was used to incorporate calculated high and normal N2O emissions into one. The annual N2O emission rate from the livestock farm in the watershed (467 ha), estimated using the HEI method, was 1156 ± 147 kg N year−1 over a 5-year period. The annual N2O emission rates calculated using the site-specific emission factor (EF = 0.0789) and the emission factor of the Intergovernmental Panel on Climate Change (EF = 0.01) were 1838 ± 585 kg N year−1 and 673 (522–1103) kg N year−1, respectively. The estimated value using the measure-and-multiply method, in which each land-use area is multiplied by the representative emission rate for each land-use type, was 964 (509–1610) kg N year−1. The N2O emission rates estimated by our newly developed method were consistent with the values calculated by the measure-and-multiply method and offered improvement over this measure because the new measure can also predict future N2O emission rates from the watershed.
Introduction
Nitrous oxide (N2O) is a major greenhouse gas and soil is one of its main sources. The global N2O emission rate from soils has been estimated to be 10.2 Tg N year−1. This is 58% of the total N2O emission rate of 17.7 Tg N year−1 (CitationIntergovernmental Panel on Climate Change 2001). Agricultural fields are considered to be one of the major sources of N2O. However, because of the large uncertainty in temporal and spatial variability in N2O fluxes, it is difficult to estimate an accurate N2O emission rate.
The use of emission factors (EFs) is one of the N2O estimation methods recommended by the United Nations Framework Convention on Climate Change (UNFCCC). The Intergovernmental Panel on Climate Change (IPCC) N2O estimation method that uses EFs is named the IPCC Tier 1 method (CitationIPCC 1997, Citation2006). When using the IPCC Tier 1 method, N2O emission rates are estimated using the sum of the background emission rate and the emission rates resulting from the N inputs to a field. This is done by multiplying the N input by the EF. The default EF value recommended by the CitationIPCC++ (1997) is 0.0125, which was calculated by CitationBouwman (1996). This value is based on the results of only 20 experiments that did not include leguminous crop data and was assumed to include a large uncertainty (CitationBouwman 1996). The default EF was changed from 0.0125 (with an uncertainty range of 0.0025–0.06) in CitationIPCC (1997) to 0.01 (with an uncertainty range of 0.003–0.03) in CitationIPCC (2006) based on further reports (e.g. CitationBouwman et al. 2002a,Citationb; CitationNovoa and Tejeda 2006; CitationStehfest and Bouwman 2006). In addition to those reports, a number of other studies have also reported different EF values (e.g. 0.0048 by CitationKaiser and Ruser 2000; 0.0118 and 0.002 by CitationHelgason et al. 2005; 0.011–0.064 by CitationKusa et al. 2002; 0.013–0.055 by CitationToma et al. 2007), indicating that there is large variability in EFs and that EFs still have to be measured to estimate more exact N2O emission rates.
Another major N2O estimation method is the measure-and-multiply method using field monitoring data (CitationCorre et al. 1999; CitationReiners et al. 1998; CitationSchimel and Potter 1995). In this method, the N2O emission rate is calculated by multiplying the representative values obtained from field measurements by the surface area of the vegetation, soil, ecosystem, or biome. The measure-and-multiply method is simple and is commonly used because the method only requires a few measured values for each surface type and it is easy to extrapolate those values to a wide area with the assumption that the N2O fluxes of the investigated site show the typical N2O emission of that surface type throughout the study region (CitationCorre et al. 1999). The advantage of this method is that the uncertainty caused by the difference among surface types can be decreased by collecting representative values for each surface type, although many samples would be required if EFs were calculated for each surface type using this method. Furthermore, this method can represent inter-annual variation if the representative values of N2O emission rates were measured at each surface type every year; estimated values calculated using the IPCC Tier 1 method cannot represent inter-annual variation. Inter-annual variation in N2O emission is very high and measurements would be required if one attempted to represent and evaluate the variation (e.g. CitationToma et al. 2007).
The measure-and-multiply method is a more advanced method than the method using EFs, but the problem of excluding extremely high N2O emission rates or outliers from the analysis needs to be addressed in both methods. CitationKaiser and Ruser (2000) defined outliers using a cluster analysis by dividing N2O emission rates into three groups: manure-fertilized plots with high N-related N2O emissions, legume plots with very low N input–output balances, and forage production plots with high N-fertilizer inputs. The outliers were excluded from the regression analysis between N2O emission rates and N input or N input–output balances. CitationHelgason et al. (2005) excluded an extremely high N2O emission value (44 kg N ha−1), which was derived from a barley field that received 60 kg of N fertilizer ha−1 where one site consistently showed emission rates 5–30-fold higher than the rates recorded at other sites in apparently uniform field plot experiments.
In our previous study (CitationKatayanagi et al. 2008), the results of 5-years of measurements of N2O emission rates in cornfield, grassland, pasture and forest in a livestock farm located in an agricultural watershed were evaluated. We began by obtaining an all-inclusive regression model, by incorporating all monitoring data on annual N2O emission rates at a livestock farm in a watershed and the N input to the soil for each measured site is as follows:
However, because our measured annual N2O emission data varied considerably and N2O emission rates from the livestock farm estimated using Eq. Equation1 included large uncertainty (CitationKatayanagi et al. 2008), we divided all N2O emission values (E all) into two groups: high values (E high, representing values higher than the overall average of 6.6 kg N ha−1, n = 9) and normal values (E norm, representing values lower than the overall average, n = 37). We then developed regression models for E high and E norm. We defined the equation for E norm as the ‘normal’ regression model:
We defined Eqs Equation3 and Equation4 as the ‘high’ regression models. Furthermore, we also defined the probability of occurrence of high N2O emissions (p f, %) to integrate the estimates calculated using these two models. The p f was calculated by the following equation:
The calculated p f values for cornfield, grassland, pasture and forest showed a weak positive correlation with precipitation for the months from May to June, from June to July, from May to August, and for August, respectively (CitationKatayanagi et al. 2008). These models and the probability of occurrence are useful because they can represent inter-annual variation in N2O emission rates and can reduce uncertainty in N2O estimations by including extremely high N2O emission rates.
In the present study, we propose a new method, the high-emission-incorporation (HEI) method, for estimating soil N2O emission rates at a watershed level using the models and the probability of occurrence of high emission rates developed in our previous paper (CitationKatayanagi et al. 2008). The main characteristic of this method is the inclusion of extremely high N2O emission rates by using not only the N input, but also the N surplus of a site and meteorological data. To verify the new method, we compared the results calculated by the new method with the results calculated using three other methods: the all-inclusive regression method, the measure-and-multiply method and the IPCC Tier 1 method.
Materials and methods
Study area and estimation of the N budget
The present study was carried out at the Shizunai Experimental Livestock Farm (467 ha), Field Science Center for Northern Biosphere, Hokkaido University, Japan (42°25′9″N, 142°29′1″E). Detailed field information was described in our previous paper (CitationKatayanagi et al. 2008).
Annual N budgets for each site were estimated for a 5-year period from 2000 to 2004 following the method outlined by CitationHayakawa et al. (2004). The N input, output and surplus were calculated mainly based on the livestock farm register. The quantity, the variety and timing of application of chemical fertilizer, the compost manure, slurry and feed supply, grazing and harvests of grass were taken into consideration when estimating the N budgets. Ammonia volatilization, denitrification, N fixation and N deposition were not taken into account in the estimation. Detailed methods and the calculated results were described in CitationHayakawa et al. (2004) and CitationKatayanagi and Hatano (2005). The calculated results for the annual N budget of the livestock farm from 2000 to 2004 are summarized in . During the study period, the total N input ranged from 20,754 to 22,561 kg N year−1, the total N output ranged from 13,453 to 19,089 kg N year−1 and the total N surplus ranged from 1,665 to 9,108 kg N year−1.
Table 1 Nitrogen input, N output and surplus N (kg N year−1) at the livestock farm (467 ha) in a watershed in 2000–2004
Estimation of annual N2O emission rates using the four methods
To verify the total annual N2O emission rates from the entire area of the livestock farm located in the watershed using the HEI method, the emission rates were also estimated using three other methods commonly in use, the all-inclusive method, the measure-and-multiply method (CitationCorre et al. 1999; CitationReiners et al. 1998; CitationSchimel and Potter 1995) and the IPCC Tier 1 method (CitationBouwman 1996; CitationIPCC 1997, Citation2006). All four methods are explained in the following sections and are summarized in .
All-inclusive regression method
The annual N2O emission rate from the watershed based on the all-inclusive method, E ai (kg N year−1), was calculated using Eq. Equation1 and the following equation:
High-emission-incorporation method
The annual N2O emission rate from the watershed based on the HEI method, E hei (kg N year−1), was calculated using the following equation:
Figure 1 Schematic diagram of different approaches for scaling up the estimation of annual N2O emission rates from a site to the watershed. WFPS, water-filled pore space.
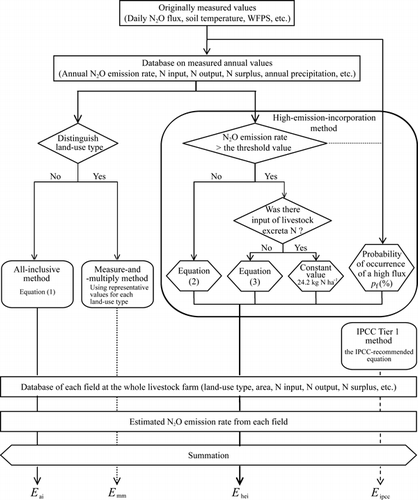
Figure 2 Diagram of the regression models for Ef1, Ef2 and Ef3. The solid bold lines represent the regression lines obtained for calculating Ef1, Ef2 and Ef3. The solid thin lines represent the regression lines at a 95% confidence interval and the dotted bold line represents Ef4. The dotted line represents the standard error of Ef4.
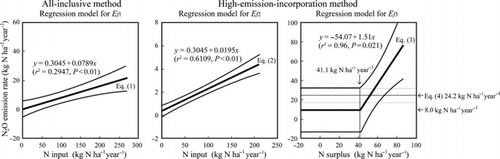
Measure-and-multiply method
The annual N2O emission rate from the watershed based on the measure-and-multiply method, E mm (kg N year−1), was calculated using the following equation:
Intergovernmental Panel on Climate Change Tier 1 method
The annual N2O emission rate from the watershed based on the IPCC Tier 1 method, E ipcc (kg N year−1), was calculated by the following equation:
Statistical analyses
Significant differences between the N2O emission rates calculated using the different methods were evaluated by Tukey’s tests (P < 0.05).
Results
The annual N2O emission rates from each site, calculated using the normal and high regression models (E f2 and E f3) for E hei, ranged from 0.3 to 40.4 kg N ha−1 year−1 and from 8.0 to 562 kg N ha−1 year−1, respectively (). The p f for cornfield, grassland, pasture and forest was 11–41%, 6.4–26%, 0–10% and 0–2.0%, respectively (). The p f and its inter-annual variation in cornfield and grassland were very large. The p f was 0% for pasture in 2002–2004 and for forest in 2000, 2001 and 2004.
The mean annual N2O emission rates from the watershed for a 5-year period, calculated using the all-inclusive regression method (Eai), the HEI method (E hei), the measure-and-multiply method (E mm) and the IPCC Tier 1 method (E ipcc), were 1838, 1156, 964 and 673 kg N year−1, respectively (). E ai and E ipcc always showed the largest and smallest values, respectively among the values estimated by the four methods. E hei and E mm had similar mean, minimum and maximum values for the 5-year period. However, the pattern of inter-annual variation in E hei and E mm was not consistent; that is, E hei had the highest value in 2001, whereas E mm had the highest value in 2002.
A comparison of the confidence interval between E ai and E hei showed that the interval for E ai was wider than that for E hei in every year (). However inter-annual variation in the confidence interval for E ai was smaller than that for E hei. Furthermore, the confidence interval for E mm and E ipcc could not be calculated because there were not enough replicates for each land-use type and each year to calculate a confidence interval for E mm. A confidence interval for the IPCC Tier 1 method was not given in CitationIPCC (2006). The inter-annual variations in minimum and maximum values of E ipcc were very small, whereas those of E mm were very large ().
Discussion
The annual N2O emission rates from the watershed calculated using the all-inclusive regression method, E ai, were significantly higher than those calculated using the other methods (), and this result was caused by the higher site-specific EF (0.0789, see Eq. Equation1) based on the N input values rather than the IPCC-recommended EF. There was a higher site-specific EF for the all-inclusive regression method because the N2O emission rates were much higher than the normal values, that is, there were ‘outliers’ that were included in the calculations of EF. Large variations in N2O emission rates in space and time have been reported by many researchers (e.g. CitationKatayanagi and Hatano 2005; CitationVelthof and Oenema 1995) and the existence of ‘outliers’ has been reported in previous papers (e.g. CitationHelgason et al. 2005; CitationKaiser and Ruser 2000). Therefore, to reduce uncertainty in N2O estimation, it would be better to include outliers using a different method.
Table 2 Ef2 and Ef3 of each land-use type in the livestock farm in 2000–2004
Table 3 Estimated annual N2O emission rates from the farm calculated using the all-inclusive (Eai), the high-emission-incorporation(Ehei), the measure-and-multiply(Emm), and the Intergovernmental Panel on Climate Change Tier 1 (Eipcc) methods for each land-use type in 2000–2004
Although the inter-annual variation and the confidence interval in E ai were smaller than in E hei, E ai was significantly higher than E hei (). The reason for the small inter-annual variation in E ai was because the estimates calculated using a site-specific EF for a 5-year period could only represent inter-annual variation in N input rates. The IPCC Tier 1 method also had similar limitations to the all-inclusive regression method. The E ipcc provided the smallest estimates () because the EF of the IPCC Tier 1 method was smaller than that of the all-inclusive regression method. In fact, inter-annual variation in N input to each site was smaller than the inter-annual variation in the N2O emission rate (coefficient of variation for N input was 3%; ), whereas that for N2O emission rates calculated using the measure-and-multiply method was 36% over a 5-year period (). To solve the problems concerning methods using an EF to estimate N2O emission that cannot represent inter-annual variation in N2O emission rates and to reduce the uncertainty in N2O estimation, a site-specific EF for each research area for each year should be calculated. However, it would be much more costly to calculate a site-specific EF for each research area for every year and it would require a method that enables estimating N2O emission rates without continuous monitoring. In view of this, we have developed the HEI method.
The HEI method is considered to be more advanced than methods using an EF and just as advanced as the measure-and-multiply method. This is because the E mm values obtained using the measure-and-multiply method are considered to be more reliable and accurate than the values obtained using a single emission factor (E ai and E ipcc). In fact, the E mm values were substantially different from the E ai and E ipcc values (). Furthermore, E hei based on our new method was similar to E mm and showed similar inter-annual variation to E mm ().
The large inter-annual variation in E hei was similar to that of E mm; however, the pattern of inter-annual variation in E mm and E hei was different. The reason for this difference could be that the application methods of incorporating the outlier values differ between the HEI method and the measure-and-multiply method. In the measure-and-multiply method, the representative values were calculated using a few measured N2O emission rates. This means, there is a possibility that outliers may not have been taken into consideration, particularly in a year when annual N2O emission rates were calculated from only a few samples. A low p f indicates the probability of their neglect, because the p f is the probability of the occurrence of high N2O emission rates and a low p f means that the probability of the occurrence of high N2O emission rates in a few replicates is low.
In contrast, the HEI method can include outliers by estimating the probability of the occurrence of high emission rates, which are calculated from a number of N2O flux values. At this point, it can be said that the HEI method is a more useful technique compared with the measure-and-multiply method in terms of reducing the possibility of overlooking extreme emission values and uncertainty in N2O estimation.
In addition, the HEI method is useful in predicting inter-annual variation in N2O emission rates at a larger scale because the occurrence probability of high N2O emissions showed a positive correlation with cumulative precipitation from May to June in the cornfield, from June to July in the grassland, from May to August in the pasture, and in August in the forest (CitationKatayanagi et al. 2008). N2O emission rates are also reported to increase after fertilization and precipitation (CitationKatayanagi et al. 2008). A number of reports have indicated the relationship between water-filled pore spaces (WFPS), which is influenced by precipitation, and N2O emission rates (CitationKoga et al. 2004; CitationKusa et al. 2006). When the timing of the N application was different among the land-use types, the WFPS values were also different in the case of the highest N2O emission rates (CitationKatayanagi et al. 2008). Therefore, it could also be possible that the p f for each land-use type showed a positive correlation with the different accumulation period of precipitation.
The HEI method is a newly developed method that will be useful for N2O estimation at a watershed scale, but the method can still be improved on in some fields, for example, with regard to the adequacy of the threshold value to divide the overall flux values into higher and normal fluxes or emission rates. This also applies to unclear mechanisms for estimating the probability of occurrence of high emission rates and a consideration of other soil types and climatic conditions.
The HEI method is new and improved in terms of estimating much more reliable N2O emission rates at a watershed level, including outliers (extremely high N2O emission rates compared with normal values). This method accounted for the existence of outliers by using N inputs, N surplus and the probability of occurrence of these high rates and precipitation. It is expected that this new method could become more useful for estimating N2O emission rates at a watershed scale by incorporating some of these improvements.
Conclusion
The HEI method, in which normal N2O emission rates and higher N2O emission rates than the normal values are calculated and incorporated into one by using the probability of occurrence of a high flux, improved our estimation of N2O emission rates. The N2O emission rates from the watershed calculated using the HEI method were consistent with estimates based on the measure-and-multiply method. In addition, the HEI method could predict future N2O emission rates from the watershed by using input N, surplus N and precipitation.
Acknowledgments
We thank Dr H. Hata and the staff of the Shizunai Experimental Livestock Farm, Field Science Center, Hokkaido University, for their assistance; Dr O. Nakahara of Hokkaido University, Dr K. P. Woli of the University of Illinois and Dr S. D. Kimura of the Tokyo University of Agriculture and Technology for useful discussions; and Miss M. Takemoto of Oyo Corporation, Dr. M. Shimizu of the Soil Science Laboratory, Mr S. Okada of Asahi Breweries and Miss Y. Usui of New Energy and Industrial Technology Development Organization for their assistance with the sampling. This paper was presented at the International Workshop on Monsoon Asia Agricultural Greenhouse Gas Emissions (MAGE-WS), 7–9 March 2006, Tsukuba, Japan. This study was partly supported by a Japanese Grant-in-Aid for Science Research from the Ministry of Education, Culture, Sports, Science and Technology (No.11460028).
References
- Bouwman , AF . 1996 . Direct emission of nitrous oxide from agricultural soils . Nutr. Cycl. Agroecosyst. , 46 : 53 – 70 .
- Bouwman , AF , Boumans , LJM and Batjes , NH . 2002a . Emissions of N2O and NO from fertilized fields. Summary of available measurement data . Glob. Biogeochem. Cycles , 16 art. no. 1058
- Bouwman , AF , Boumans , LJM and Batjes , NH . 2002b . Modeling global annual N2O and NO emissions from fertilized fields . Glob. Biogeochem. Cycles , 16 art. no. 1080
- Corre , MD , Pennock , DJ , Van Kessel , C and Elliott , DK . 1999 . Estimation of annual nitrous oxide emissions from a transitional grassland-forest region in Saskatchewan, Canada . Biogeochemistry , 44 : 29 – 49 .
- Hayakawa , A , Hatano , R and Hata , H . Nitrogen budget approach for environmental evaluation of nitrogen management in beef cattle farming system, southern Hokkaido, Japan . Proc. of the Third International Nitrogen Conference . Edited by: Zhu , Z , Minami , K and Xing , G . pp. 65 – 70 . Monmouth Junction : Science Press USA .
- Helgason , BL , Janzen , HH Chantigny , MH . 2005 . Toward improved coefficients for predicting direct N2O emissions from soil in Canadian agroecosystems . Nutr. Cycl. Agroecosyst. , 72 : 87 – 99 .
- Intergovernmental Panel on Climate Change . 1997 . Revised 1996 IPCC Guidelines for National Greenhouse Gas Inventories , Kamiyamaguchi : IPCC Task Force on National Greenhouse Gas Inventories, TSU, Institute for Global Environmental Strategies, the Institute for Global Environmental Strategies (IGES) for the IPCC .
- Intergovernmental Panel on Climate Change . 2001 . IPCC Third Assessment Report: Climate Change 2001, the Scientific Basis , Cambridge : Cambridge University Press .
- Intergovernmental Panel on Climate Change . 2006 . 2006 IPCC Guidelines for National Greenhouse Gas Inventories , Vol. 4 , 11.6 – 11.10 . Agriculture, Forestry and Other Land Use, the Institute for Global Environmental Strategies (IGES) for the IPCC . http://www.ipcc-nggip.iges.or.jp/public/2006gl/vol4.htm
- Kaiser , E-A and Ruser , R . 2000 . Nitrous oxide emissions from arable soils in Germany: an evaluation of six long-term field experiments . J. Plant Nutr. Soil Sci. , 163 : 249 – 260 .
- Katayanagi , N and Hatano , R . 2005 . Spatial variability of greenhouse gas fluxes from soils of various land uses on a livestock farm in southern Hokkaido, Japan . Phyton (Austria) Special Issue: “APGC 2004” , 45 : 309 – 318 .
- Katayanagi , N , Sawamoto , T , Hayakawa , A and Hatano , R . 2008 . Nitrous oxide and nitric oxide fluxes from cornfield, grassland, pasture and forest in a watershed in Southern Hokkaido, Japan . Soil Sci. Plant Nutr. , 54 : 662 – 680 .
- Koga , N , Tsuruta , H , Sawamoto , T , Nishimura , S and Yagi , K . 2004 . N2O emission and CH4uptake in arable fields managed under conventional and reduced tillage cropping systems in northern Japan . Global Biogeochem. Cycles , 18 : GB4025
- Kusa , K , Hu , RG , Sawamoto , T and Hatano , R . 2006 . Three years of nitrous oxide and nitric oxide emissions from silandic andosols cultivated with maize in Hokkaido, Japan . Soil Sci. Plant Nutr. , 52 : 103 – 113 .
- Kusa , K , Sawamoto , T and Hatano , R . 2002 . Nitrous oxide emissions for 6 years from a gray lowland soil cultivated with onions in Hokkaido, Japan . Nutr. Cycl. Agroecosyst. , 63 : 239 – 247 .
- Novoa , R and Tejeda , HR . 2006 . Evaluation of the N2O emissions from N in plant esidues as affected by environmental and management factors . Nutr. Cycl. Agroecosyst. , 75 : 29 – 46 .
- Reiners , WA , Keller , M and Gerow , KG . 1998 . Estimating rainy season nitrous oxide and methane fluxes across forest and pasture landscapes in Costa Rica . Water, Air, Soil Pollut. , 105 : 117 – 130 .
- Schimel , DS and Potter , CS . 1995 . “ Process modeling and spatial extrapolation: biogenic trace gases ” . In Measuring Emissions from Soil and Water , Edited by: Matson , PA and Harris , RC . 358 – 383 . Cambridge, MA : Blackwell .
- Stehfest , E and Bouwman , L . 2006 . N2O and NO emission from agricultural fields and soils under natural vegetation: summarizing available measurement data and modeling of global annual emissions . Nutr. Cycl. Agroecosyst. , 74 : 207 – 228 .
- Toma , Y , Kimura , SD , Hirose , Y , Kusa , K and Hatano , R . 2007 . Variation in the emission factor of N2O derived from chemical nitrogen fertilizer and organic matter: A case study of onion fields in Mikasa, Hokkaido, Japan . Soil Sci. Plant Nutr. , 53 : 692 – 703 .
- Velthof , GL and Oenema , O . 1995 . Nitrous oxide fluxes from grassland in the Netherlands. I. Statistical analysis of flux-chamber measurements . Eur. J. Soil Sci. , 46 : 533 – 540 .