Abstract
Volcanic acidification has created unique ecosystems that have had to adapt to the acidic environments in volcanic regions. To characterize the primary microbial properties of strongly acidified soils in such environments, we investigated microbial biomass, nitrogen transformations and other relevant chemical properties in the surface soils of solfatara and forests from Osorezan, a typical volcanic region in Japan, and compared the results to common Japanese forest soils. Soil microbial biomass C (MBC) and N (MBN) were determined using the chloroform fumigation–extraction method. Potential net N mineralization and net nitrification were measured in aerobic laboratory incubations. Long-term acidification in the Osorezan soils by volcanic hydrogen sulfide deposition caused low soil pH (3.0–3.8), base cation deficiency and increased concentrations of toxic ions such as Al3+. The proportions of MBC to total carbon (MBC/TC ratio) and MBN to total nitrogen (MBN/TN ratio) were lower than those in common Japanese forest soils. The extreme acidic conditions may have inhibited microbial survival in the Osorezan acid soils. Net N mineralization occurred at rates comparable to those in common Cryptomeria japonica forest soils, probably because of the presence of acid-tolerant soil microorganisms. Net nitrification was completely inhibited and autotrophic ammonia oxidizers were not detected by the MPN method. The inhibition of nitrification prevents nitrogen leaching from the soils, thus maintaining a nitrogen cycle in the volcanic acid region in which NH+ 4 (and NH3) is recycled among microorganisms and plants.
Introduction
Japan, located in the Pacific Ring of Fire, has over 100 active volcanoes (CitationJapan Meteorological Agency 2005). Some volcanoes, such as Sakurajima and Miyakejima, erupt frequently and there are many regions where acidic gases, including hydrogen sulfide (H2S), sulfur dioxide and hydrogen chloride, are emitted from fumaroles. Long-term exposure to these gases has acidified the soils and freshwaters in volcanic regions. This acidification increases the concentrations of Al3+ and heavy metals in the soil solution. These increased concentrations may be toxic to plants and soil microorganisms (CitationTakamatsu et al. 1992; CitationYoshitake et al. 2007). Because only a few organisms can survive in these extreme volcanic environments, special ecosystems exist that have adapted to acidic conditions (CitationSatake et al. 1995; CitationYoshitake et al. 2007). For example, solfatara plants, such as Carex angustisquama and Deschampsia flexuosa, are distributed around fumaroles (CitationTsujimura 1977; CitationYoshida and Wakamatsu 1991). CitationYoshitake et al. (2007) recorded reduced soil microbial biomass and different microbial communities in a solfatara soil compared with an adjacent forest soil.
Chemical and microbiological investigations of strongly acidified soils in volcanic regions are important for understanding soil elemental cycles in soil ecosystems adapted to acidic environments. Osorezan (in Aomori, Japan) is a typical volcanic region acidified by H2S gas emitted from fumaroles (CitationSatake et al. 1995; CitationTakamatsu et al. 1992). Many researchers have studied the plant and fish ecology of acidic ecosystems in Osorezan (CitationHirata et al. 2003; CitationSatake et al. 1995; CitationTsujimura 1977). CitationTakamatsu et al. (1992) studied exchangeable cations and sulfur in Osorezan acid soils with and without vegetation. However, soil microbiology in this area remains unstudied.
The aim of the present study was to characterize the primary microbial properties of acid soils from Osorezan. For this purpose, we analyzed microbial biomass and the rate of nitrogen transformations (mineralization and nitrification), together with relevant chemical properties. We compared the microbial biomass and N mineralization rate of Osorezan acid soils to those in common Japanese forest soils to characterize the fertility status and nitrogen cycle in these acid soils.
Materials and methods
Study area and soil sampling
The study area is the Osorezan volcanic region (41°19′N, 141°6′E) located in the city of Mutsu, Aomori, Japan, 200–300 m a.s.l. (). The mean annual temperature and precipitation in this area are approximately 10°C and 1,400 mm, respectively (CitationJapan Meteorological Agency 2009). The area has a central crater lake (Lake Usorisan) and is dotted with many fumaroles that emit high H2S concentrations and several hot springs (CitationTakamatsu et al. 1992). The Osorezan volcano erupted intermittently between 240,000 and 300,000 years ago, with a few explosive eruptions after that, but there have been no eruptions in the last 10,000 years (CitationKuwabara and Yamazaki 2001).
Soil samples were collected from six sites at different distances from the fumaroles. The soil type and vegetation at each site are summarized in . Three sites in Oniishi (Sites 1–3) are located in the solfatara field near the shore of the crater lake, near which are distributed many fumaroles (). The soil surface at Site 1, which is closest to the fumaroles, is exposed to H2S at a level of 2.3 mg S cm−2 month−1 (CitationTakamatsu et al. 1992). This site is almost bare land, although some solfatara plants (e.g. Carex angustisquama and Deschampsia flexuosa) are present in the vicinity (CitationTsujimura 1977). Although Sites 2 and 3 are adjacent to Site 1, a dense vegetation cover of dwarf bamboo (Sasa kurilensis) is present at these sites. Sites 4 and 5 are located in artificial coniferous forests of Cryptomeria japonica and Thujopsis dolabrata, respectively. The stands in these sites appeared healthy, although their location is relatively near the fumaroles. Site 6, located outside the Osorezan crater, was selected as a reference site. This site is the least affected by volcanic gases because of interception by the somma and has the same vegetation (Cryptomeria japonica) as Site 4 and the same soil type (Dystric Cambisol) as Sites 4 and 5.
Figure 1 Map showing the sampling sites. Sites 1–5 are acidified sites located within the crater of Osorezan and Site 6 is a reference site. Asterisks (*) indicate the fumaroles.
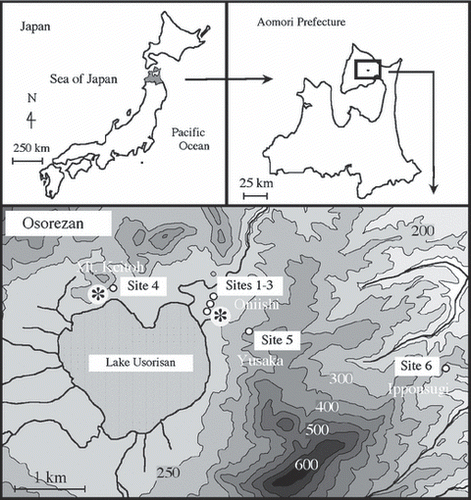
Table 1 Site characteristics in the present study
After removing the fresh litter and the rhizomes of dwarf bamboo at Sites 2 and 3, surface soil samples (primarily F/H/A horizons) were collected because these were expected to be most affected by volcanic acid deposition. The depth of the soil collected at each site is shown in . Because the soil had no well-differentiated horizons, a yellowish-brown color was considered to be the B-horizon and the upper part of the soil above the B-horizon was collected. Soil samples at Sites 2–5 were collected predominantly from the F/H horizons because of the thickness of these horizons. Sampling was conducted in summer from 1998 to 2000 and the collected samples were transported to the laboratory in a cooling box.
Soil chemical analysis
Aliquots of the soil samples were used to measure the pH and the concentrations of water-soluble elements. Soil pH was measured in both water and 1 mol L–1 KCl suspensions (1:2.5 w/v) with a glass electrode. Soils (10 g) were extracted with 50 mL deionized water by shaking for 30 min on a reciprocal shaker. The resulting extracts were filtered (pore size: 0.45-μm, 13AI; GL Sciences, Tokyo, Japan). The concentrations of SO2- 4, Cl−, NO- 3, PO3 4 and NH+ 4 in the extracts were measured by ion chromatography (DX-100; Dionex, Osaka, Japan), and the concentrations of Al, K, Na, Ca and Mg were measured by inductively coupled plasma-atomic emission spectrometry (ICP-AES) (ICAP-750; Nippon Jarrell-Ash, Tokyo, Japan) after adjusting the pH of extracts to <2 with HNO3. The remaining soil samples were sieved (<5 mm), mixed and then stored at 4°C until use (generally within 1 month).
Aliquots of the stored soil samples were air-dried, ground and homogenized for analysis of total carbon (TC), nitrogen (TN) and sulfur (TS) contents. Total C and TN were measured after combustion with a CN corder (MT-500, Yanagimoto, Kyoto, Japan). For TS analysis, the soil samples (10 mg) were digested with mixtures of 60% HNO3 (2 mL) and 60% HClO4 (1 mL) in stainless-steel high-pressure digestion bombs (140°C, 6 h) and subsequently with 50% HF (1 mL) on a hot plate (at ∼190°C) (CitationHou et al. 2006; CitationTakamatsu et al. 1992). The digests obtained were diluted with deionized water, filtered (pore size: 0.45-μm) and analyzed for sulfur by ICP-AES (61E-Trace; Thermo Jarrell-Ash, MA).
Soil microbial analysis
Determination of soil microbial biomass C, N and S
The microbial biomass carbon (MBC), nitrogen (MBN) and sulfur (MBS) in the soils were determined using the chloroform fumigation–extraction method (CitationBrookes et al. 1985;CitationSaggar et al. 1981; CitationVance et al. 1987). Before analysis, the stored soil samples were adjusted to 60% of their respective water-holding capacities and pre-incubated at 25°C for 10 days in the dark to stabilize microbial activities (CitationVance et al. 1987; CitationWu et al. 1994). Pre-incubated soil samples (10 g fresh weight) were fumigated with alcohol-free chloroform for 24 h at 25°C in a sealed desiccator. After removal of the chloroform, the fumigated soil samples were extracted with 50 mL of 0.5 mol L–1 K2SO4 by shaking for 30 min and the extract was filtered. For the analysis of MBS, 0.1 mol L–1 CaCl2 was used as the extractant. Unfumigated soil samples were also subjected to extraction using the same method. Organic carbon in the K2SO4 extracts was measured by an automatic combustion TOC analyzer (TOC-5000; Shimadzu, Kyoto, Japan). The MBC was calculated as E C/k EC, where E C = (organic C extracted from fumigated soils) − (organic C extracted from unfumigated soils) and k EC (conversion factor) = 0.45 (CitationJoergensen 1996; CitationWu et al. 1990). Total nitrogen in the K2SO4 extracts was determined using the hydrazine reduction method (CitationHayashi et al. 1997; CitationKamphake et al. 1967) following alkaline persulfate oxidation (CitationCabrera and Beare 1993). The MBN was calculated as E N/k EN, where E N = (total N extracted from fumigated soils) − (total N extracted from unfumigated soils) and k EN = 0.54 (CitationBrookes et al. 1985; CitationJoergensen and Mueller 1996). Total S in the CaCl2 extracts was measured by ICP-AES following HNO3 digestion, which was conducted to convert organic S to SO2- 4 and/or to prevent the formation of precipitates. The MBS was calculated as E S/k ES, where E S = (total S extracted from fumigated soils) − (total S extracted from unfumigated soils) and k ES = 0.35 (CitationBanerjee and Chapman 1996; CitationWu et al. 1994).
Compilation of data on MBC, MBN and MBS in common Japanese forest soils
To compare the soil parameters obtained in the present study with those from common Japanese forest soils, previously published data on TC, TN, TS, MBC, MBN and MBS were compiled. All compiled data for microbial biomass were determined using the chloroform fumigation–extraction method. However, the conversion factors for MBC and MBN (k EC and k EN) in the literature differed occasionally from those used in the present study. In such cases, the data were corrected using our values (i.e. k EC = 0.45 and k EN = 0.54). When compiling the data, values influenced by fertilization or weeding were excluded. In addition, the data used were taken only from texts and tables and not from figures.
Data on MBC and MBN were abundant for mineral soil horizons (e.g. CitationOhse et al. 2003b; CitationTokuchi et al. 2000), but limited for organic soil horizons (CitationChihara et al. 2000; CitationOyanagi et al. 2002a). Therefore, data for both MBC and MBN were used without distinction in our discussion, although some Osorezan acid soils had thick organic soil horizons. Soil microbial biomass varies significantly with several soil factors, such as vegetation, soil type, temperature and water conditions (CitationBauhus et al. 1998; CitationDevi and Yadava 2006; CitationOyanagi et al. 2002a; CitationSato et al. 2000); thus, only tentative comparisons of soil microbial biomass and related parameters may be possible between Osorezan acid soils and common Japanese forest soils.
Determination of N mineralization and nitrification rates
Potential net N mineralization and net nitrification rates were determined by aerobic incubation of the soils. Because the soil samples had been stored at 4°C for 1–6 months, the soils were pre-incubated as previously described to allow the microbial activity to restore and stabilize. Pre-incubated soil samples (5 g dry weight) were then placed into 100 mL bottles and sealed loosely. The bottles were incubated at 30°C for 4 weeks in the dark, with the moisture maintained at 60% of water-holding capacity by periodic addition of deionized water (control plots). Soil samples spiked with ammonium (200 mg N kg−1 dry soil) as (NH4)2SO4 solution prior to incubation were also incubated in a similar manner (+NH+ 4 plots). The +PO3 4 plots were used to study the effect of NH+ 4 accumulation on rates of N mineralization and nitrification. Fifteen bottles were prepared for both the control plots and the +NH+ 4 plots. Three replicate bottles were retrieved from each plot weekly and NH+ 4 and NO- 3 were extracted with 50 mL of 1 mol L–1 KCl by shaking for 30 min. The extracts were filtered and analyzed colorimetrically for NH+ 4-N and NO- 3-N using the Berthelot reaction (salicylate–nitroprusside–hypochlorite method) (CitationAnderson and Ingram 1993; CitationSearle 1984) and the hydrazine reduction method (CitationHayashi et al. 1997; CitationKamphake et al. 1967), respectively. Rates of net N mineralization and net nitrification during the 4-week incubation were calculated from the differences in total inorganic N (sum of NH+ 4-N and NO- 3-N) and NO- 3-N concentrations, respectively, before and after incubation in the control plots. NO- 2-N was not detected in the extracts throughout the incubation.
Enumeration of autotrophic ammonia oxidizers
Autotrophic ammonia oxidizers in pre-incubated soil were enumerated using a microtechnique for the most-probable-number (MPN) method (CitationRowe et al. 1977). Until recently, ammonia oxidation, the first step in nitrification, was considered to be carried out almost exclusively by chemolithoautotrophic ammonia-oxidizing bacteria in soils. However, recent molecular ecological studies have suggested that ammonia-oxidizing archaea may also play an important role in nitrification (CitationHayatsu et al. 2008; CitationLeininger et al. 2006; CitationNakaya et al. 2009; CitationProsser and Nicol 2008). The MPN method is expected to detect both groups of microorganisms as ammonia oxidizers because it is based on the chemical detection of potential ammonia oxidation. Five grams of pre-incubated soil samples and 45 mL of sterile water were placed into sterile bottles and shaken vigorously for 10 min on a reciprocal shaker. Serial 10-fold dilutions were made by adding 1 mL of the suspension to 9 mL of sterile water. The neutral inorganic medium for ammonia oxidizers contained (in g L–1): (NH4)2SO4, 0.5; K2HPO4, 1.0; MgSO4·7H2O, 0.3; NaCl, 0.3; FeSO4·7H2O, 0.03; CaCO3, 7.5. The medium (0.1 mL) was dispensed into each well of a 96-well microtiter plate and the serial dilution (0.1 mL) was inoculated into eight replicate wells for each dilution. The pH values in the medium inoculated with 10-fold dilutions ranged from 6.3 to 6.9. The inoculated media were incubated at 30°C for 4 weeks in the dark. Growth of ammonia oxidizers was determined by the production of nitrite and/or nitrate in the medium. Nitrite was detected using the Griess–Ilosvay reaction following the reduction of nitrate to nitrite by the addition of zinc powder. The numbers of wells that were positive or negative to the growth test were noted, and the MPN values were calculated from a MPN table (CitationRowe et al. 1977).
Statistical analysis
All statistical calculations were carried out using StatView 4.5j (Abacus Concepts, CA).
Results and Discussion
Soil chemical properties
The chemical properties of the Osorezan acid soils are shown in . The soil pH (H2O) at Sites 1–5 within the crater ranged from 3.0 to 3.8, and the soil pH at Site 6 was 5.4 (). The soil pH at Sites 1–5 was much lower than that recorded in Japanese forest soils, where the soil pH (H2O) of mineral soils ranged from 3.5 to 8.1, with a median of 5.1 (CitationTakahashi et al. 2001). The pH at Site 6 (the reference site) located outside the crater was close to the median of the Japanese forest soils. The surface soil at Site 1 adjacent to the fumaroles was the most strongly acidified. Direct exposure of soil and vegetation surfaces to volcanically generated H2S and its subsequent oxidation to H2SO4 has been shown to cause soil acidification at Osorezan (CitationTakamatsu et al. 1992). Low soil pH around fumaroles has also been reported in other Japanese volcanic regions, for example, pH 2.4–4.2 at Mt Garandake in Oita prefecture (CitationYoshitake et al. 2007) and pH 2.5–3.9 at Hachimantai in Iwate prefecture (CitationYoshida and Wakamatsu 1991).
Total C and TN were lowest at Site 1, which has little or no vegetation. At the other sites with vegetation, Sites 2–5 had higher TC and TN than Site 6 (the reference site) (). The thick F/H horizons at Sites 2–5 result from the accumulation of organic matter on the soil surface, probably resulting from the suppressed decomposition of litter and plant residues under strongly acidic conditions (CitationAnderson and Joergensen 1997). The TS at Sites 1–5 (1.0–2.2 g kg−1) was comparable to that in organic and mineral soils in Japanese forests (∼0.1–2.2 g kg−1) (CitationSakuma et al. 1994; CitationTakamatsu et al. 1992; CitationTanikawa et al. 2003), despite the continuous exposure of the soils to H2S.
CitationTakamatsu et al. (1992) showed that over 70% of the sulfur is present as organic S and that inorganic S is present exclusively as SO2- 4 in acid soils from Oniishi (corresponding to Sites 1–3). In fact, SO2- 4 was the major anion in the soil extracts from Sites 1–5, and the proportion of NO- 3 to total anions increased from Site 5 to Site 1, with increasing closeness to fumaroles (). In contrast, at Site 6 (the reference site), SO2- 4 was the major anion and was also the dominant form of inorganic N in the soil extracts (). However, at Sites 2–5 NO- 3 was not detected except at Site 4, and NH+ 4 was the dominant form of inorganic N. Base cations (Ca2+, Mg2+, K+ and Na+) were dominant at Site 6, whereas acidic cations (H+ and Al3+) increased at Sites 1–5 with decreasing soil pH (). The high concentrations of K+ at Sites 2 and 3 and Ca2+ at Sites 4 and 6 might have been influenced by the vegetation because Sasa kurilensis and Cryptomeria japonica accumulate K (CitationTakamatsu et al. 1997) and Ca (CitationBaba et al. 2004), respectively.
Cation exchange and aluminum hydrolysis are the main pH-buffering systems in acid soils (CitationUlrich 1991). However, the soils from Oniishi (corresponding to Sites 1–3) were depleted not only in exchangeable base cations, but also in aluminum (exchangeable Al3+ and Al-oxides) as a result of long-term exposure to acidic volcanic gases (CitationTakamatsu et al. 1992). Thus, the pH-buffering system has progressed to the next stage, in which iron hydrolysis and H+-exchange at strongly acidic sites of soluble and insoluble organic matter and clay minerals are initiated (CitationTakamatsu et al. 1992). Similarly, the acid soils from Osorezan demonstrate a unique inorganic environment.
Table 2 Chemical and microbial properties of the Osorezan acid soils
Soil microbial properties
Microbial biomass C, N and S
The MBC, MBN and MBS are shown in . These values tended to be higher at Sites 2–5 where the soils were rich in organic matter, although a significant correlation was found only between TC and MBC (r = 0.884, P < 0.01, n = 6). Soil pH showed no significant correlation with MBC, MBN or MBS (r = −0.129, 0.208 and 0.065, respectively, P > 0.1, n = 6). Soil organic matter contents and vegetation types are significant parameters controlling soil microbial biomass (CitationBanerjee and Chapman 1996; CitationBauhus et al. 1998; CitationChowdhury et al. 1999; CitationOhya et al. 2000; CitationOyanagi et al. 2002a). The ratios of MBC to MBN (biomass C/N) were correlated with those of TC to TN (soil C/N) (r = 0.962, P < 0.01, n = 6). Thus, biomass C/N ratios in the Osorezan acid soils might be affected by soil C/N ratios. The MBC, MBN, and MBS were well correlated with each other (r = 0.810–0.940, P ≤ 0.05, n = 6).
To characterize the soil microbial biomass at Osorezan, the relationships between MBC and TC and between MBN and TN were compared with literature data from common Japanese forest soils (). In the common forest soils, MBC was positively correlated with TC (r = 0.746, P < 0.01, n = 49) (). The MBN was also correlated with TN (r = 0.488, P < 0.01, n = 41) (). The proportion of MBC to TC (the MBC/TC ratio) ranged from 0.5 to 4.2%, with an arithmetic mean and standard deviation of 1.7 ± 0.9%, and the MBN/TN ratio was 3.9 ± 2.8% (range: 0.7–16.4%). In contrast, the MBC/TC ratio in the Osorezan acid soils was low (<0.5%) even at Sites 2–5 where the TC was high (). The MBN/TN ratio in the Osorezan acid soils was relatively low even at Sites 2–5 with high TN, and ranged from 0.3 to 1.7% at Sites 1–5 (). These results indicate that the microbial biomass is less in the Osorezan acid soils than in common Japanese forest soils.
Figure 3 Relationships between (a) total C (TC) and microbial biomass C (MBC), (b) total N (TN) and microbial biomass N (MBN), (c) MBN and MBC, and (d) TN and TC in Osorezan acid soils () and common Japanese forest soils (○). Values are expressed on an oven-dry soil basis (DS). The site number is labeled near each plot. Values in common Japanese forest soils were cited from: Billore et al. 1995; Chihara et al. 2000; Chowdhury et al. 1999; Guan et al. 1997; Furusawa et al. 2005; Mabuhay et al. 2006; Ohse et al. 2003a,b; Ohya et al. 2000; Oyanagi et al. 2002a,b; Sato et al. 2000; Sato and Seto 1995; Tamai et al. 2003; Tokuchi et al. 2000; Tripathi et al. 2005, 2006.
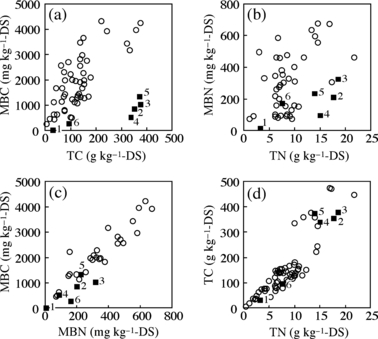
Soil microbial biomass generally decreases with acidification. CitationAnderson and Joergensen (1997) showed a decreasing MBC/TC ratio with decreasing soil pH in a German beech forest. CitationThirukkumaran and Morrison (1996) and CitationPennanen et al. (1998) found that the ratio of MBC/TC in forest soils decreased with the application of simulated acid rain. Only a few microbial communities can adapt to extremely acidic soil environments that are deficient in nutrients such as base cations and rich in potentially toxic ions such as Al3+ (CitationPennanen et al. 1998; CitationYoshitake et al. 2007). Thus, microbial biomass and survival may have been restricted in the Osorezan acid soils, resulting in low MBC/TC and MBN/TN ratios.
Relationships between MBC and MBN and between TC and TN were also compared between the Osorezan acid soils and the common forest soils. In the latter, MBC was highly correlated with MBN (r = 0.934, P < 0.01, n = 27) as shown in and the biomass C/N ratio was 6.6 ± 1.8 (range: 4.8–14.0). These values were similar to those (7.0 ± 0.3) found in forest soils around the globe (CitationCleveland and Liptzin 2007). Relative to these values, biomass C/N ratios in the Osorezan acid soils were slightly low, although this difference was not significant (). Total C was correlated with TN (r = 0.865, P < 0.01, n = 63) in common forest soils (), and the soil C/N ratio was 17.2 ± 5.2 (range: 8.2–33.4). Soil C/N ratios in the Osorezan acid soils were almost equivalent to this (). Thus, biomass C/N and soil C/N ratios were similar in the Osorezan acid soils to those in common Japanese forest soils.
Although soil C/N ratios in the Osorezan acid soils were equivalent to those in common Japanese forest soils, the concentrations of elements other than C and N in dwarf bamboo and litter have been found to be significantly different in the Osorezan area from those in other non-acidic areas (CitationTakamatsu et al. 1997). Therefore, the change in quality (availability and decomposability) of soil organic matter may have influenced the microbial biomass (CitationBauhus et al. 1998; CitationOhse et al. 2003b; CitationRaubuch and Beese 2005). At Sites 2 and 3, it is also possible that competitive nutrient uptake by the dense dwarf bamboo inhibited any increase in microbial biomass (CitationTakamatsu et al. 1997; CitationTripathi et al. 2006).
Although information on MBS is limited, CitationChowdhury et al. (1999) showed that MBS and the MBS/TS ratio in two different Japanese forest soils were 6.4 and 8.0 mg kg−1 and 4.0 and 3.4%, respectively. When including grassland and arable land, the values were 0.8–13.4 mg kg−1 and 1.1–4.0%, respectively. CitationBanerjee and Chapman (1996) found that MBS in global forest soils ranged from 2.9 to 140 mg kg−1. The MBS values in the Osorezan acid soils (3.8–73.2 mg kg−1) were higher than those found by CitationChowdhury et al. (1999), but were within the range found in global forest soils. However, the MBS/TS ratios (0.4–4.3%) were similar to those found by CitationChowdhury et al. (1999).
Nitrogen mineralization and nitrification
To clarify the nitrogen cycle in the Osorezan acid soils, the potential rates of N mineralization and nitrification were measured in aerobic laboratory incubations. shows the changes in NH+ 4-N and NO- 3-N concentrations during the course of the incubation. At Sites 1–5, NH+ 4-N increased almost linearly throughout the incubation period, whereas NO- 3-N did not increase. A similar increase in NH+ 4-N was observed in the +NH+ 4 plots. In contrast, at Site 6 (the reference site), although NH+ 4-N was always present in trace amounts, NO- 3-N increased during the incubation and at day 28 the proportion of NO- 3-N to total inorganic nitrogen was 97%. In the +NH+ 4 plots, NH+ 4-N decreased and NO- 3-N increased concomitantly. These results indicated that net N mineralization occurred at approximately constant rates at all sites, whereas net nitrification occurred only at Site 6 and was inhibited completely at Sites 1–5.
Figure 4 Changes in -N and -N concentrations in Osorezan acid soils during aerobic incubation. Values are expressed on an oven-dry soil basis (DS). In the + plots, the soil was spiked with ammonium (200 mg N kg−1) before the incubation. Concentrations of -N and -N in the control plots at the start of the incubation were set to zero.
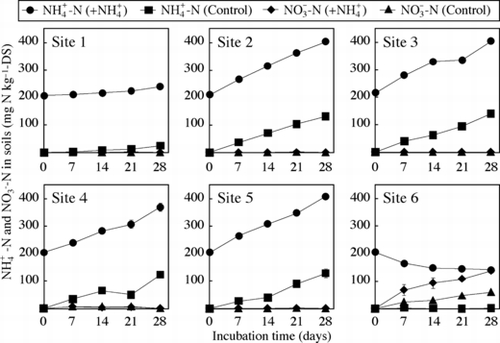
At Sites 1–5, autotrophic ammonia oxidizers were not detected in the soil by the MPN method (). In addition, NO- 3-N was not detected in the soil extracts except at Site 4 (). These results suggest that ammonia oxidizers are absent or that activity is seriously inhibited in the Osorezan acid soils. Ammonia oxidation is conducted mainly by a relatively narrow range of bacteria and maybe by archaea, although it remains unclear as to what extent archaea actually contribute to nitrification activity in soils (CitationHayatsu et al. 2008; CitationLeininger et al. 2006; CitationNakaya et al. 2009; CitationProsser and Nicol 2008). Ammonia-oxidizing bacteria (e.g. Nitrosospira and Nitrosomonas) prefer NH3 to NH+ 4 as a substrate, but the availability of NH3 decreases exponentially with decreasing pH (NH3+ H+↔NH+ 4; pKa = 9.25), and there are few acid-tolerant strains that can use NH+ 4 at low pH (CitationDe Boer and Kowalchuk 2001; CitationFrijlink et al. 1992). Although pH-neutral medium were used for MPN enumeration, ammonia oxidizers were not detected in the Osorezan acid soils. In addition to nitrogen availability, other factors such as the presence of natural inhibitors and water stress suppress the activity of autotrophic nitrifiers (CitationDe Boer and Kowalchuk 2001; CitationHirobe et al. 1998; CitationPaavolainen et al. 1998; CitationTokuchi et al. 2000). In contrast, heterotrophic or fungal nitrification also contributes to nitrification in some acid forest soils (CitationBrierley et al. 2001; CitationDe Boer and Kowalchuk 2001). At Site 4, the concentration of NO- 3-N did not increase, but certain amounts (∼38 mg-N kg−1) of NO- 3 were detected throughout the incubation period, although NO- 3 was not detected in the other acid soils. This NO- 3 might have been produced by heterotrophic or fungal nitrification in view of the absence of autotrophic ammonia oxidizers ().
The rates of net N mineralization in the control plots were in the order: Site 3 (139 mg N kg−1 4 weeks−1) ≥Site 2 (132) ≥ Site 5 (128) ≥ Site 4 (122) > Site 6 (62) > Site 1 (25). The corresponding values in the +NH+ 4 plots ranged from 32 to 203 mg N kg−1 4 weeks−1, and were 1.2–1.6-fold higher than those in the control plots. This result suggests that NH+ 4 accumulation probably accelerates N mineralization in the soils. Because NH+ 4 was accumulated during the storage and pre-incubation periods in the control plots (21–273 mg N kg−1), there is a possibility that the N mineralization rates were overestimated. The N mineralization rate was significantly correlated with both TC (r = 0.989, P < 0.001, n = 6) and TN (r = 0.979, P < 0.001, n = 6), without the influence of soil pH. A similar dependence of N mineralization rate on the amount of soil organic matter has been reported by CitationHirobe et al. (1998) and CitationHirai et al. (2006). The N mineralization rates in the Osorezan acid soils were compared with the rate in common Cryptomeria japonica forest soils. The latter ranged from 12.5 to 245.3 mg N kg−1 4 weeks−1 and the arithmetic mean ± standard deviation were 93.7 ± 58.0 mg N kg−1 4 weeks−1 (n = 85) (CitationHirai et al. 2006). The N mineralization rates observed in the Osorezan acid soils (25–139 mg N kg−1 4 weeks−1) were within the range of those found in common forest soils. In contrast to nitrification, N mineralization is conducted by various microorganisms, some of which may have been able to adapt to the acidic conditions in Osorezan and maintain mineralization rates.
Inorganic N is essential for plants and soil microorganisms, but NO- 3-N is readily leached from soils. A lack of nitrification prevents nitrogen leaching from the acid soils, and thus an optimum nitrogen cycle, in which NH+ 4 (and NH3) is recycled among soil microorganisms and plants, may have been established in Osorezan.
Acknowledgments
The authors thank Dr M. Nishikawa, Mrs R. Kumata and Mrs M. Okawa of the National Institute for Environmental Studies for assistance with the ICP-AES analysis
References
- Anderson , JM and Ingram , JSI . 1993 . “ Colorimetric determination of ammonium ” . In Tropical soil biology and fertility: a handbook of methods , Edited by: Anderson , JM and Ingram , JSI . 42 – 43 . Wallingford : CAB International .
- Anderson , TH and Joergensen , RG . 1997 . Relationship between SIR and FE estimates of microbial biomass C in deciduous forest soils at different pH . Soil Biol. Biochem. , 29 : 1033 – 1042 .
- Baba , M , Kato , M , Sugiura , T and Kobayashi , H . 2004 . Calcium accumulation alleviates soil acidification in Japanese cedar (Cryptomeria japonica) stands . Soil Sci. Plant Nutr. , 50 : 403 – 411 .
- Banerjee , MR and Chapman , SJ . 1996 . The significance of microbial biomass sulphur in soil . Biol. Fertil. Soils , 22 : 116 – 125 .
- Bauhus , J , Pare , D and Cote , L . 1998 . Effects of tree species, stand age and soil type on soil microbial biomass and its activity in a southern boreal forest . Soil Biol. Biochem. , 30 : 1077 – 1089 .
- Billore , SK , Ohsawa , M , Numata , M and Okano , S . 1995 . Microbial biomass nitrogen pool in soils from a warm temperate grassland, and from deciduous and evergreen forests in Chiba, central Japan . Biol. Fertil. Soils , 19 : 124 – 128 .
- Brierley , EDR , Wood , M and Shaw , PJA . 2001 . Influence of tree species and ground vegetation on nitrification in an acid forest soil . Plant Soil , 229 : 97 – 104 .
- Brookes , PC , Landman , A , Pruden , G and Jenkinson , DS . 1985 . Chloroform fumigation and the release of soil nitrogen: a rapid direct extraction method to measure microbial biomass nitrogen in soil . Soil Biol. Biochem. , 17 : 837 – 842 .
- Cabrera , ML and Beare , MH . 1993 . Alkaline persulfate oxidation for determining total nitrogen in microbial biomass extracts . Soil Sci. Soc. Am. J. , 57 : 1007 – 1012 .
- Chihara , M , Oyanagi , N , Toda , H and Haibara , K . 2000 . Effects of soil microbial flora on nitrogen mineralization in forest soil . For. Resour. Environ. , 38 : 97 – 106 . (in Japanese with English summary)
- Chowdhury , MAH , Kouno , K and Ando , T . 1999 . Correlation among microbial biomass S, soil properties, and other biomass nutrients . Soil Sci. Plant Nutr. , 45 : 175 – 186 .
- Cleveland , CC and Liptzin , D . 2007 . C:N:P stoichiometry in soil: Is there a “Redfield ratio” for the microbial biomass? . Biogeochemistry , 85 : 235 – 252 .
- De Boer , W and Kowalchuk , GA . 2001 . Nitrification in acid soils: micro-organisms and mechanisms . Soil Biol. Biochem. , 33 : 853 – 866 .
- Devi , NB and Yadava , PS . 2006 . Seasonal dynamics in soil microbial biomass C, N and P in a mixed-oak forest ecosystem of Manipur, North-east India . Appl. Soil Ecol. , 32 : 220 – 227 .
- Frijlink , MJ , Abee , T , Laanbroek , HJ , De Boer , W and Konings , WN . 1992 . The bioenergetics of ammonia and hydroxylamine oxidation in Nitrosomonas europaeaat acid and alkaline pH . Arch. Microbiol. , 157 : 194 – 199 .
- Furusawa , H , Hino , T , Kaneko , S and Araki , M . 2005 . Effect of dwarf bamboo (Sasa nipponica) and deer (Cervus nippon centralis) on the chemical properties of soil and microbial biomass in a forest at Ohdaigahara, central Japan . Bulletin of FFPRI , 4 : 157 – 165 .
- Guan , G , Marumoto , T , Shindo , H and Nishiuama , M . 1997 . Relationship between the amount of microbial biomass and the physicochemical properties of soil – comparison between volcanic and non-volcanic ash soils . Jpn J. Soil Sci. Plant Nutr. , 68 : 614 – 621 . (in Japanese with English summary)
- Hayashi , A , Sakamoto , K and Yoshida , T . 1997 . A rapid method for determination of nitrate in soil by hydrazine reduction procedure . Jpn J. Soil Sci. Plant Nutr. , 68 : 322 – 326 . (in Japanese)
- Hayatsu , M , Tago , K and Saito , M . 2008 . Various players in the nitrogen cycle: diversity and functions of the microorganisms involved in nitrification and denitrification . Soil Sci. Plant Nutr. , 54 : 33 – 45 .
- Hirai , K , Sakata , T , Morishita , T and Takahashi , M . 2006 . Characteristics of nitrogen mineralization in the soil of Japanese cedar (Cryptomeria japonica) and their responses to environmental changes and forest management . J. Jpn. For. Soc. , 88 : 302 – 311 . (in Japanese with English summary)
- Hirata , T , Kaneko , T Ono , T . 2003 . Mechanism of acid adaptation of a fish living in a pH 3.5 lake . Am. J. Physiol. Regul. Integr. Comp. Physiol. , 284 : R1199 – R1212 .
- Hirobe , M , Tokuchi , N and Iwatsubo , G . 1998 . Spatial variability of soil nitrogen transformation patterns along a forest slope in a Cryptomeria japonicaD. Don plantation . Eur. J. Soil Biol. , 34 : 123 – 131 .
- Hou , H , Takamatsu , T , Koshikawa , MK and Hosomi , M . 2006 . Concentrations of Ag, In, Sn, Sb and Bi, and their chemical fractionation in typical soils in Japan . Eur. J. Soil Sci. , 57 : 214 – 227 .
- Japan Meteorological Agency . 2005 . “ Definition of the active volcano ” . In National catalogue of the active volcanoes in Japan , 3rd edn , Edited by: Japan Meteorological Agency . 7 Tokyo : Japan Meteorological Business Support Center . in Japanese
- Japan Meteorological Agency2009Climate Statisticshttp://www.data.jma.go.jp/obd/stats/data/en/index.html(November 2009)
- Joergensen , RG . 1996 . The fumigation–extraction method to estimate soil microbial biomass: calibration of the kECvalue . Soil Biol. Biochem. , 28 : 25 – 31 .
- Joergensen , RG and Mueller , T . 1996 . The fumigation–extraction method to estimate soil microbial biomass: calibration of the kENvalue . Soil Biol. Biochem. , 28 : 33 – 37 .
- Kamphake , LJ , Hannah , SH and Cohen , JM . 1967 . Automated analysis for nitrate by hydrazine reduction . Water Res. , 1 : 205 – 216 .
- Kuwabara , T and Yamazaki , H . 2001 . Tephrostratigraphy and eruptive history during the last 450,000 years at Osore-zan volcano, Shimokita peninsula, northeast Japan . Kazan , 46 : 37 – 52 . (in Japanese with English summary)
- Leininger , S , Urich , T Schloter , M . 2006 . Archaea predominate among ammonia-oxidizing prokaryotes in soils . Nature , 7104 : 806 – 809 .
- Mabuhay , JA , Nakagoshi , N and Isagi , Y . 2006 . Microbial responses to organic and inorganic amendments in eroded soil . Land Degrad. Develop. , 17 : 321 – 332 .
- Nakaya , A , Onodera , Y Nakagawa , T . 2009 . Analysis of ammonia monooxygenase and archaeal 16S rRNA gene fragments in nitrifying acid-sulfate soil microcosms . Microbes Environ. , 24 : 168 – 174 .
- Ohse , K , Ohkawa , Y , Tamura , K and Higashi , T . 2003a . Influences of forest decline on various properties of soils on Mt. Hirugatake, Tanzawa mountains, Kanto district, Japan: II. changes in some physical and chemical properties of surface soils . Soil Sci. Plant Nutr. , 49 : 171 – 177 .
- Ohse , K , Tamura , K and Higashi , T . 2003b . Influences of forest decline on various properties of soils on Mt. Hirugatake, Tanzawa mountains, Kanto district, Japan: III. changes in microbial biomass and enzyme activities of surface soils . Soil Sci. Plant Nutr. , 49 : 179 – 184 .
- Ohya , S , Tamura , K and Azuma , J . 2000 . Microbial biomass and enzyme activity of soils in an urban area . Tsuchi To Biseibutsu , 54 : 159 – 164 .
- Oyanagi , N , Chihara , M , Haibara , K and Toda , H . 2002a . Nitrogen and carbon mineralization characteristics of different decomposition stages in forest floor of some tree species . Jpn J. Soil Sci. Plant Nutr. , 73 : 363 – 372 . (in Japanese with English summary)
- Oyanagi , N , Chihara , M , Toda , H and Haibara , K . 2002b . Characteristics of carbon and nitrogen mineralization of forest soils in different slopes and vegetation . J. Jpn. For. Soc. , 84 : 111 – 119 . (in Japanese with English summary)
- Paavolainen , L , Kitunen , V and Smolander , A . 1998 . Inhibition of nitrification in forest soil by monoterpenes . Plant Soil , 205 : 147 – 154 .
- Pennanen , T , Fritze , H , Vanhala , P , Kiikkila , O , Neuvonen , S and Baath , E . 1998 . Structure of a microbial community in soil after prolonged addition of low levels of simulated acid rain . Appl. Environ. Microbiol. , 64 : 2173 – 2180 .
- Prosser , JI and Nicol , GW . 2008 . Relative contributions of archaea and bacteria to aerobic ammonia oxidation in the environment . Environ. Microbiol. , 10 : 2931 – 2941 .
- Raubuch , M and Beese , F . 2005 . Influence of soil acidity on depth gradients of microbial biomass in beech forest soils . Eur. J. For. Res. , 124 : 87 – 93 .
- Rowe , R , Todd , R and Waide , J . 1977 . Microtechnique for most-probable-number analysis . Appl. Environ. Microbiol. , 33 : 675 – 680 .
- Saggar , S , Bettany , JR and Stewart , JWB . 1981 . Measurement of microbial sulfur in soil . Soil Biol. Biochem. , 13 : 493 – 498 .
- Sakuma , T , Tomita , A , Shibata , H and Tanaka , Y . 1994 . Distribution and cycling of sulfur in deciduous and coniferous forest ecosystems as influenced by acid deposition I : distribution of biomass and sulfur . Jpn J. Soil Sci. Plant Nutr. , 65 : 677 – 683 . (in Japanese with English summary)
- Satake , K , Oyagi , A and Iwao , Y . 1995 . Natural acidification of lakes and rivers in Japan: the ecosystem of Lake Usoriko (pH 3.4–3.8) . Water Air Soil Pollut. , 85 : 511 – 516 .
- Sato , A and Seto , M . 1995 . Relationship between the size of microbial biomass carbon and the amounts of mineral ions in arable and forest soils . Tsuchi To Biseibutsu , 46 : 51 – 59 . (in Japanese with English summary)
- Sato , A , Tsuyuzaki , T and Seto , M . 2000 . Effect of soil agitation, temperature or moisture on microbial biomass carbon of a forest and an arable soil . Microbes Environ. , 15 : 23 – 30 . (in Japanese with English summary)
- Searle , PL . 1984 . The Berthelot or indophenol reaction and its use in the analytical-chemistry of nitrogen: a review . Analyst. , 109 : 549 – 568 .
- Takahashi , M , Sakata , T and Ishizuka , K . 2001 . Chemical characteristics and acid buffering capacity of surface soils in Japanese forests . Water Air Soil Pollut. , 130 : 727 – 732 .
- Takamatsu , T , Boratynski , J and Satake , K . 1992 . Effects of volcanic acid deposition on soil chemistry: I. status of exchangeable cations and sulfur . Soil Sci. , 154 : 435 – 449 .
- Takamatsu , T , Kohno , T , Ishida , K , Sase , H , Yoshida , T and Morishita , T . 1997 . Role of the dwarf bamboo (Sasa) community in retaining basic cations in soil and preventing soil acidification in mountainous areas of Japan . Plant Soil , 192 : 167 – 179 .
- Tamai , N , Takenaka , C , Ishizuka , S and Tezuka , T . 2003 . Methane flux and regulatory variables in soils of three equal-aged Japanese cypress (Chamaecyparis obtusa) forests in central Japan . Soil Biol. Biochem. , 35 : 633 – 641 .
- Tanikawa , T , Takahashi , M , Imaya , A , Inagaki , Y and Ishizuka , K . 2003 . Vertical distributions and accumulations of sulfate in Andisols and Inceptisols – adsorbed and soluble sulfate fractions . Jpn J. Soil Sci. Plant Nutr. , 74 : 149 – 155 . (in Japanese with English summary)
- Thirukkumaran , CM and Morrison , IK . 1996 . Impact of simulated acid rain on microbial respiration, biomass, and metabolic quotient in a mature sugar maple (Acer saccharum) forest floor . Can. J. For. Res. , 26 : 1446 – 1453 .
- Tokuchi , N , Hirobe , M and Koba , K . 2000 . Topographical differences in soil n transformation using 15N dilution method along a slope in a conifer plantation forest in Japan . J. For. Res. , 5 : 13 – 19 .
- Tripathi , SK , Sumida , A Ono , K . 2006 . The effects of understorey dwarf bamboo (Sasa kurilensis) removal on soil fertility in a Betula ermaniiforest of northern Japan . Ecol. Res. , 21 : 315 – 320 .
- Tripathi , SK , Sumida , A , Shibata , H , Uemura , S , Ono , K and Hara , T . 2005 . Growth and substrate quality of fine root and soil nitrogen availability in a young Betula ermaniiforest of northern Japan: effects of the removal of understory dwarf bamboo (Sasa kurilensis) . For. Ecol. Manage. , 212 : 278 – 290 .
- Tsujimura , A . 1977 . Difference of the vegetation of solfataras according to their position on the vegetation zone . Jpn. J. Ecol. , 27 : 319 – 322 . (in Japanese)
- Ulrich , B . 1991 . “ An ecosystem approach to soil acidification ” . In Soil acidity , Edited by: Ulrich , B and Sumner , ME . 28 – 79 . Berlin : Springer-Verlag .
- Vance , ED , Brookes , PC and Jenkinson , DS . 1987 . An extraction method for measuring soil microbial biomass-C . Soil Biol. Biochem. , 19 : 703 – 707 .
- Wu , J , Joergensen , RG , Pommerening , B , Chaussod , R and Brookes , PC . 1990 . Measurement of soil microbial biomass C by fumigation extraction - an automated procedure . Soil Biol. Biochem. , 22 : 1167 – 1169 .
- Wu , J , O’Donnell , AG , He , ZL and Syers , JK . 1994 . Fumigation–extraction method for the measurement of soil microbial biomass-S . Soil Biol. Biochem. , 26 : 117 – 125 .
- Yoshida , M and Wakamatsu , Y . 1991 . Soil pH and vegetation of solfatara near the Tohsichi hot spring, Iwate prefecture . Jpn J. Soil Sci. Plant Nutr. , 62 : 434 (in Japanese)
- Yoshitake , S , Sasaki , A , Uchida , M , Funatsu , Y and Nakatsubo , T . 2007 . Carbon and nitrogen limitation to microbial respiration and biomass in an acidic solfatara field . Eur. J. Soil Biol. , 43 : 1 – 13 .