Abstract
To assess the effects of scion and rootstock genotypes on plant growth and the anti-oxidant defense systems of cucumber, two cucumber cultivars (“Jinchun No. 2”, a relatively salt-sensitive cultivar, and “Jinyu No. 1”, a relatively salt-tolerant cultivar) were grafted onto two rootstocks (“Figleaf Gourd”, a relatively salt-sensitive cultivar, and “Chaojiquanwang”, a relatively salt-tolerant cultivar), respectively. The grafted plants were grown hydroponically and were exposed to 0 and 100 mmol L−1 NaCl stress under greenhouse conditions. The results showed that the salt injury index, leaf Na+ content and root hydrogen peroxide (H2O2) content in the plants grafted onto Figleaf Gourd were higher, whereas the activities of superoxide dismutase (SOD), peroxidase (POD) and catalase (CAT) in the leaves and roots were lower than those of the plants grafted onto Chaojiquanwang under NaCl stress, independent of the scion genotypes. This resulted in smaller reductions in root dry weight, shoot dry weight and leaf area in the plants grafted onto Chaojiquanwang. The salt injury index was positively correlated with the leaf Na+ content and H2O2 content in the leaves and roots, but was negatively correlated with the activities of SOD and POD in the roots. The effects of scion on the salt injury index, Na+ content in the leaves, H2O2 content and activities of POD and CAT in the roots were significant, whereas there were no significant effects of scion on the root and shoot dry weights, leaf area and leaf relative water content. Overall, these results suggest the following: (1) the salt-tolerant rootstock was more important in determining the salt tolerance of the grafted cucumber seedlings under NaCl stress compared with the scion genotypes, (2) the higher salt tolerance of the grafted cucumber seedlings could be partially attributed to the lower leaf Na+ content and root H2O2 content and higher activities of SOD, POD and CAT in the roots.
Introduction
Currently, approximately 20% of the world’s cultivated land and nearly half of all irrigated land are affected by salinity (CitationZhu 2001). Therefore, salinization has been a major factor limiting agricultural crop production (CitationParida and Das 2005). Salt stress causes multifarious adverse effects in plants. Thus, overcoming salt-stress problems will have a positive impact on agriculture production.
Ion regulation is an essential factor in the mechanism of salt tolerance in many crops. Na+ is the primary cause of ion-specific damage under salt stress (CitationTrajkova et al. 2006). Excessive accumulation of Na+ results in toxicity and growth inhibition (CitationSaqib et al. 2005). However, the effect of salinity can be mediated, at least partially, by the enhanced generation of reactive oxygen species (ROS), which cause oxidative stress (CitationAshraf 2009). These ROS are highly reactive and can seriously disrupt normal metabolism through oxidative damage to lipids, proteins and nucleic acids in the absence of any protective mechanism (CitationMittler 2002). In general, plants possess an anti-oxidant system that includes anti-oxidant enzymes, such as superoxide dismutase (SOD), catalase (CAT) and peroxidase (POD), that protect their cells against ROS. Superoxide dismutase, the first enzyme in the detoxifying process, converts O2 − to H2O2 and O2. Catalase and POD scavenge the accumulated H2O2 to non-toxic levels by conversion to H2O and O2 (CitationApel and Hirt 2004).
Numerous recent studies have used traditional breeding programs and molecular engineering to improve plant salt tolerance (CitationCuartero et al. 2006), but commercial success has been very limited because of the issue of salt tolerance (CitationFlowers 2004). Recently, there has been a great deal of effort in determining the response of grafted plants to saline conditions and in demonstrating that grafting is a valid strategy for increasing the salt tolerance of tomato (CitationHe et al. 2009; CitationMartinez-Rodriguez et al. 2008; CitationZhang et al. 2008), watermelon (CitationGoreta et al. 2008) and muskmelon (CitationXu et al. 2006). Our previous study on cucumber also suggests that grafting improves plant adaptation to salt stress (CitationHuang et al. 2009; CitationZhu et al. 2008b).
Grafting is an integrative reciprocal process and, therefore, both scion and rootstock influence salt tolerance (CitationEtehadnia et al. 2008). However, some studies have revealed that the growth and salt tolerance of grafted plants under saline conditions depend on the rootstock (CitationGarcia-Sanchez et al. 2006; CitationMickelbart and Arpaia 2002). CitationRomero et al. (1997) also observed that root characteristics are of primary importance in determining the salt tolerance of melon plants. In addition, the importance of the root system in the regulation of salt tolerance has also been documented in salt-sensitive and salt-tolerant potato genotypes (CitationShaterian et al. 2005a). In contrast, CitationChen et al. (2003) concluded that scion genotypes play an important role in the growth of grafted tomato plants, regardless of the salinity in the growing media, whereas rootstock has little influence. Studies on tomato plants suggest that the characteristics of the rootstock able to induce salt tolerance in the shoot depend on the salt tolerance of the shoot genotype (CitationSanta-Cruz et al. 2002). Our previous work on cucumber also suggested that the salt tolerance of grafted cucumber seedlings is related to the shoot genotype (CitationZhu et al. 2008b). However, an experiment with potato indicated that salt tolerance is controlled by both root and shoot factors (CitationEtehadnia et al. 2008). Therefore, more studies are necessary to investigate the primary factor that determines the salt tolerance of grafted plants.
The objective of the present experiment was to evaluate the effects of scion and rootstock genotypes on the growth and anti-oxidant defense systems of grafted cucumber seedlings under NaCl stress. Furthermore, we aimed to assess which is the primary factor that determines the salt tolerance of grafted cucumber seedlings.
Material and methods
Plant materials and treatments
Two cucumber (Cucumis sativus L.) cultivars, “Jinyu No. 1,” a relatively salt-tolerant cultivar, and “Jinchun No. 2,” a relatively salt-sensitive cultivar, were used as scions. The pumpkin cultivar “Chaojiquanwang” (Cucurbita moschata Duch.), a relatively salt-tolerant cultivar, and “Figleaf Gourd” (Cucurbita ficifolia B.), a relatively salt-sensitive cultivar (CitationZhu et al. 2006), were used as rootstocks. The experiment took place in a glasshouse situated at the experimental farm of Huazhong Agricultural University in central China (30°27′ N, 114°20′ E). The plants were cultivated under natural light conditions at 18–30°C and 60–70% relative humidity. Seeds of the rootstocks Chaojiquanwang and Figleaf Gourd were sown on 10 March 2008 into 50 seedling plug trays filled with a 2:1 (v/v) mixture of peat and perlite. Four days later, the seeds of the scions Jinyu No. 1 and Jinchun No. 2 were sown. When the seedlings of the rootstock had developed one true leaf, two scions were grafted onto the two rootstocks, respectively, using the “insert grafting” procedure described by CitationLee (1994). Four types of graft combinations were obtained: Jinyu No. 1/Chaojiquanwang (J1/C, scion/rootstock), Jinyu No. 1/Figleaf Gourd (J1/F), Jinchun No. 2/Chaojiquanwang (J2/C) and Jinchun No. 2/Figleaf Gourd (J2/F). After grafting for 10 days, the plants were transferred to 20 L plastic containers (12 plants per container) containing half-strength Hoagland solution (CitationHoagland and Arnon 1950). At the three-leaf stage, the plants were subjected to 0 (control) and 100 mmol L−1 NaCl for 10 days. The electrical conductivity (EC) of the nutrient solutions was 2.15 and 13.20 dS m−1, respectively. To avoid salt shock, the NaCl was added to the nutrient solutions in increments of 50 mmol L−1 per day until the desired concentrations were obtained. The nutrient solution was managed according to the method of CitationZhu et al. (2008c). Each treatment was replicated three times with 12 plants per replication.
Calculating the salt injury index
Salt tolerance was classified by visual appearance after 10 days of NaCl stress. Classification of the standard and calculation of the salt injury index followed the method of CitationShaterian et al. (2005b), with some modification. Leaf injury ratings were carried out on the second leaf above the base of the plant because the leaf is very sensitive to salt stress. The classified criteria of salt injury were: level 1 (0% area of yellow leaf), level 2 (1–25% area of yellow leaf), level 3 (26–50% area of yellow leaf), level 4 (51–75% area of yellow leaf) and level 5 (76–100% area of yellow leaf). The salt injury index was calculated using the following equation: salt injury index = Σ (level value × plant number)/total plant number.
Plant sampling
After 10 days of NaCl stress, plant materials were sampled from 10:00 to 11:00 AM. Six randomly selected plants per treatment were taken to measure plant dry weight and then the Na+ contents in the roots and leafs were determined using the dried material (two plants per replication). The first fully expanded fresh leaves of another six plants per treatment were used for measuring leaf relative water content (RWC). The first fully expanded fresh leaves of the remaining 24 plants per treatment (eight plants per replication) were immediately frozen in liquid nitrogen and stored at −76°C for later H2O2 content, as well as anti-oxidant enzyme activity analysis.
Determination of plant dry weight
Plants were collected, rinsed with deionized water and blotted carefully with tissue paper. Plant materials were separated into shoots (the part above the graft union) and roots (the part below the graft union). Leaf area was determined using a leaf area meter (LiCOR 3100; Li-cor, Lincoln, NB, USA). The dry weights were determined after oven-drying at 65°C for 2 days and then stored for further determination of the Na+ content.
Determination of sodium content, relative water content, and antioxidant parameters
The concentration of Na+ was determined using an atomic absorption spectrophotometer (Varian spectra AA 220; Varian, Palo Alto, CA, USA) according to the method of CitationDasgan et al. (2002). Leaf RWC was measured according to the method of CitationWeatherley (1950). The H2O2 levels were calculated according to the method described by CitationBrennan and Frenkel (1977). The SOD (EC: 1.15.1.1) and POD (EC: 1.11.1.7) activities were determined following CitationDhindsa et al. (1981), and CAT (EC: 1.11.1.6) activity was measured according to the method of CitationLiu (2000).
Statistical analysis
Data were subjected to a three-factor ANOVA with two scions, two rootstocks and two concentrations of NaCl using SAS 8.1 software (SAS Institute, Cary, NC, USA), and the means were separated using a Duncan’s multiple range test at P < 0.05. Linear correlation analysis was done between the salt injury index and other parameters of the grafted cucumber plants using SAS 8.1 software.
Results
Salt tolerance
Significant salt injury was observed in all graft combinations under 100 mmol L−1 NaCl stress (). The salt injury index of the plants grafted onto Figleaf Gourd was significantly higher than that of plants grafted onto Chaojiquanwang, regardless of the scion genotypes, indicating that grafting with the Chaojiquanwang rootstock could improve the salt tolerance of cucumber seedlings more effectively than grafting with Figleaf Gourd. Moreover, the salt injury index was higher in grafted Jinchun No. 2 plants than in grafted Jinyu No. 1 plants when Figleaf Gourd was used as the rootstock.
Figure 1 Salt injury index of the grafted cucumber seedlings under 0 mmol L−1 and 100 mmol L−1 NaCl stress. Leaf damage score: level 1 (0% area of yellow leaf), level 2 (1–25% area of yellow leaf), level 3 (26–50% area of yellow leaf), level 4 (51–75% area of yellow leaf) and level 5 (76–100% area of yellow leaf). Values are the mean ± standard error (n = 3). Bars with the same letters indicate no significant difference according to Duncan’s multiple range test (P < 0.05). J1/C, Jinyu No. 1 grafted onto Chaojiquanwang; J1/F, Jinyu No. 1 grafted onto Figleaf Gourd; J2/C, Jinchun No. 2 grafted onto Chaojiquanwang; J2/F, Jinchun No. 2 grafted onto Figleaf Gourd.
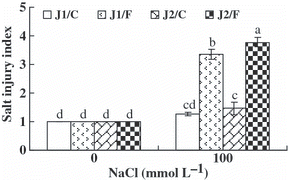
Plant growth
The 100 mmol L−1 NaCl treatment decreased the root and shoot dry weights, the leaf area and the leaf RWC in all graft combinations (). However, the reductions in the plants grafted onto Figleaf Gourd were more severe than those in plants grafted onto Chaojiquanwang under NaCl stress, regardless of the scion genotypes. For example, salinity decreased the root and shoot dry weights, leaf area and leaf RWC of J2/C by 16, 57, 62 and 13%, respectively, whereas the respective values of J2/F were 54, 72, 79 and 15%. These results indicated that grafting with Chaojiquanwang could compensate for the growth reduction of cucumber plants caused by NaCl stress more efficiently than grafting with Figleaf Gourd.
The dry weights of the roots and shoots, leaf area and leaf RWC were all significantly affected by salinity, and the leaf area and root dry weight were also significantly affected by rootstock. However, there were no significant effects of scion on the dry weights of the roots and shoots, leaf area and leaf RWC ().
Na+concentration
The Na+ content in the leaves and roots of all graft combinations increased under NaCl stress, except for the leaf Na+ content in J1/C and J2/C (). Under 100 mmol L−1 NaCl stress, the Na+ content was significantly higher in the roots, but was significantly lower in the leaves of plants grafted onto Chaojiquanwang than those grafted onto Figleaf Gourd, regardless of the scion genotypes. These results indicated that plants grafted onto Chaojiquanwang could limit the transport of Na+ to the leaves more effectively than plants grafted onto Figleaf Gourd under NaCl stress. Furthermore, the Na+ content in the leaves was higher in grafted Jinchun No. 2 plants than in grafted Jinyu No. 1 plants, when Figleaf Gourd was used as rootstock.
Table 1 Plant growth of grafted cucumber seedlings under 0 and 100 mmol L−1 NaCl stress
Figure 2 Na+ contents in the roots and leaves of grafted cucumber seedlings under 0 mmol L−1 and 100 mmol L−1 NaCl stress. Values are the mean ± standard error (n = 3). Bars with the same letters indicate no significant difference according to Duncan’s multiple range test (P < 0.05). J1/C, Jinyu No. 1 grafted onto Chaojiquanwang; J1/F, Jinyu No. 1 grafted onto Figleaf Gourd; J2/C, Jinchun No. 2 grafted onto Chaojiquanwang; J2/F, Jinchun No. 2 grafted onto Figleaf Gourd; DW, dry weight.
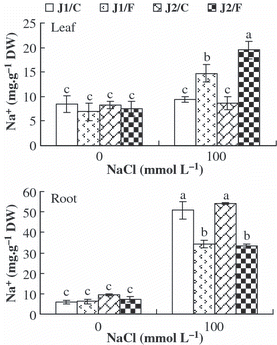
Figure 3 H2O2 content in the leaves and roots of the grafted cucumber seedlings under 0 mmol L−1 and 100 mmol L−1 NaCl stress. Values are the mean ± standard error (n = 3). Bars with the same letters indicate no significant difference according to Duncan’s multiple range test (P < 0.05). J1/C, Jinyu No. 1 grafted onto Chaojiquanwang; J1/F, Jinyu No. 1 grafted onto Figleaf Gourd; J2/C, Jinchun No. 2 grafted onto Chaojiquanwang; J2/F, Jinchun No. 2 grafted onto Figleaf Gourd; FW, fresh weight.
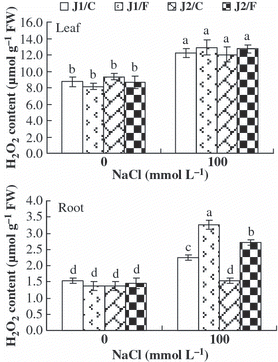
H2O2content
The H2O2 content in the leaves and roots of all graft combinations increased under NaCl stress, except for the root H2O2 content in J2/C (). However, under 100 mmol L−1 NaCl stress, the H2O2 content in the roots was significantly higher in plants grafted onto Figleaf Gourd than those grafted onto Chaojiquanwang, regardless of the scion genotypes; whereas the H2O2 content in the roots was higher in grafted Jinyu No. 1 plants than in grafted Jinchun No. 2 plants, regardless of the rootstock genotypes. These results indicated that the J2/C plants could limit excessive H2O2 accumulation in the roots more effectively than other graft combinations under NaCl stress.
Anti-oxidant enzyme activities
In general, under 100 mmol L−1 NaCl stress, the SOD and POD activities in the leaves and roots of plants grafted onto Chaojiquanwang were higher than those of plants grafted onto Figleaf Gourd, regardless of the genotype of the scion (,). The POD activity in the roots of grafted Jinyu No. 1 plants was higher than that of grafted Jinchun No. 2 plants under NaCl stress because Chaojiquanwang was used as rootstock. In addition, the SOD and POD activities in the leaves of plants grafted onto Chaojiquanwang were higher than those of plants grafted onto Figleaf Gourd under 0 mmol L−1 NaCl stress, regardless of the genotype of the scion.
The CAT activities in the leaves of all graft combinations and the CAT activities in the roots of plants grafted onto Chaojiquanwang increased under NaCl stress, whereas the CAT activities in the roots of plants grafted onto Figleaf Gourd decreased, regardless of the genotype of the scion (). In addition, the CAT activities in the roots of grafted Jinyu No. 1 plants were lower than those of grafted Jinchun No. 2 plants under NaCl stress because Figleaf Gourd was used as the rootstock.
Figure 4 Superoxide dismutase (SOD) activity in the leaves and roots of the grafted cucumber seedlings under 0 mmol L−1 and 100 mmol L−1 NaCl stress. Values are the mean ± standard error (n = 3). Bars with the same letters indicate no significant difference according to Duncan’s multiple range test (P < 0.05). J1/C, Jinyu No. 1 grafted onto Chaojiquanwang; J1/F, Jinyu No. 1 grafted onto Figleaf Gourd; J2/C, Jinchun No. 2 grafted onto Chaojiquanwang; J2/F, Jinchun No. 2 grafted onto Figleaf Gourd; FW, fresh weight.
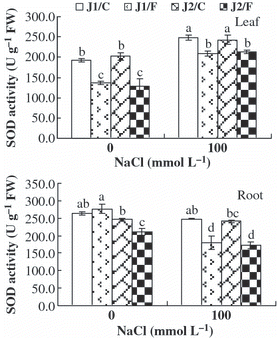
Figure 5 Peroxidase (POD) activity in the leaves and roots of grafted cucumber seedlings under 0 mmol L−1 and 100 mmol L−1 NaCl stress. Values are the mean ± standard error (n = 3). Bars with the same letters indicate no significant difference according to Duncan’s multiple range test (P < 0.05). J1/C, Jinyu No. 1 grafted onto Chaojiquanwang; J1/F, Jinyu No. 1 grafted onto Figleaf Gourd; J2/C, Jinchun No. 2 grafted onto Chaojiquanwang; J2/F, Jinchun No. 2 grafted onto Figleaf Gourd; FW, fresh weight.
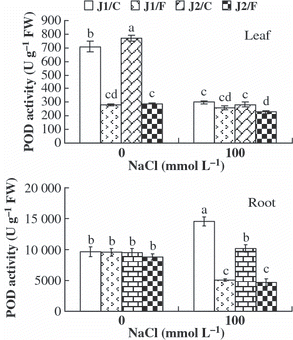
Correlation analysis
A correlation analysis between salt injury index and the other parameters of the grafted cucumber plants was conducted (). Salt injury index was found to be positively correlated with the leaf Na+ content and H2O2 content in the leaves and roots, but was negatively correlated with the activities of SOD and POD in the roots. Root H2O2 content was positively correlated with leaf Na+ content and leaf H2O2 content, but was negatively correlated with root SOD activity. Moreover, root SOD activity was positively correlated with root POD activity, and root POD activity was positively correlated with root CAT activity.
Discussion
Rootstock effects
Salt stress, like many other abiotic stresses, inhibits plant growth (CitationZhu 2001). When compared with the control (0 mmol L−1 NaCl), the salt injury index increased, but the shoot and root dry weights, leaf area and leaf RWC decreased in all grafted plants under NaCl stress (; ). However, the increase in salt injury index and the decreases in root and shoot dry weights and leaf area were higher in plants grafted onto Figleaf Gourd than those in plants grafted onto Chaojiquanwang under NaCl stress, regardless of the scion genotypes. This implies that grafting with the relatively salt-tolerant rootstock alleviated the growth inhibition and salt injury of cucumber seedlings caused by NaCl stress more effectively than grafting with the relatively salt-sensitive rootstock. This result supports similar findings in loquat (CitationLópez-Gómez et al. 2007). Thus, we conclude that the growth of cucumber plants in the presence of NaCl depends on the rootstock used.
Figure 6 Catalase (CAT) activity in the leaves and roots of grafted cucumber seedlings under 0 mmol L−1 and 100 mmol L−1 NaCl stress. Values are the mean ± standard error (n = 3). Bars with the same letters indicate no significant difference according to Duncan’s multiple range test (P < 0.05). J1/C, Jinyu No. 1 grafted onto Chaojiquanwang; J1/F, Jinyu No. 1 grafted onto Figleaf Gourd; J2/C, Jinchun No. 2 grafted onto Chaojiquanwang; J2/F, Jinchun No. 2 grafted onto Figleaf Gourd; FW, fresh weight.
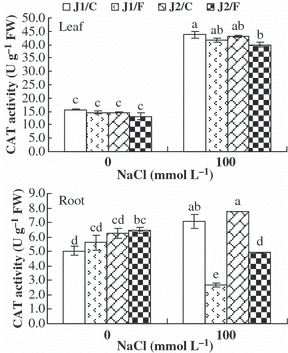
The higher salt tolerance of grafted cucumber seedlings is associated with lower Na+ concentrations in the leaves, and the rootstock roots of grafted cucumber seedlings play an important role in limiting the transport of Na+ to the leaves (CitationZhu et al. 2008b). In the present study, we also found that plants grafted with salt-tolerant rootstock had higher Na+ content in the roots, but lower Na+ content in the leaves, regardless of the scion genotypes (). Therefore, we conclude that salt-tolerant rootstock play an important role in alleviating Na+ toxicity in the shoots by limiting the transport of Na+ to the leaves. The correlation analysis indicated that the salt injury index was positively correlated with the Na+ content in the leaves (), implying that Na+ in the leaves is an important cause of salt injury. These results also support similar findings in tomato (CitationEstañet al. 2005).
Salt stress also leads to oxidative stress through an increase in ROS, such as superoxide (O2 −), H2O2 and hydroxyl radicals (OH−) (CitationMittler 2002), and it can injure the plant cell if it is not eliminated in time (CitationZhang et al. 2005). Some evidence suggests that resistance to oxidative stress may, at least in part, be involved in salt stress tolerance (CitationAshraf 2009; CitationBadawi et al. 2004; CitationMittova et al. 2002). CitationRout and Shaw (2001) reported that salt-tolerant plants should have a better anti-oxidant defense system for the effective removal of ROS, including anti-oxidant enzymes such as SOD, POD and CAT. In our study, under 100 mmol L−1 NaCl stress, the plants grafted onto Chaojiquanwang had lower H2O2 content and higher SOD, POD and CAT activities in the roots than the plants grafted onto Figleaf Gourd, regardless of the genotype of the scion (–). It appears that cucumber plants have a higher anti-oxidant capacity to eliminate H2O2 when they are grafted onto relatively salt-tolerant rootstock than onto relatively salt-sensitive rootstock under NaCl stress, regardless of the scion genotypes. This result is consistent with the results found in tomato (CitationHe et al. 2009) and loquat (CitationLópez-Gómez et al. 2007). The correlation analysis indicated that salt injury index was positively correlated with H2O2 content in the roots, but was negatively correlated with the activities of SOD and POD in the roots (). It appears that the salt tolerance of cucumber seedlings is associated with the anti-oxidant defense system for the effective removal of H2O2. This supports similar findings in tobacco (CitationRuiz et al. 2006) and eggplant (CitationWei et al. 2009). Therefore, we conclude that salt-tolerant rootstock play an important role in alleviating the oxidative damage caused by NaCl by enhancing the anti-oxidant capacity.
Table 2 Correlation coefficient between the salt injury index and the other parameters of the grafted cucumber seedlings under 0 and 100 mmol L−1 NaCl stress
However, the H2O2 content in the leaves increased under saline conditions, but there was not a rootstock or scion genotypic difference. And the differences in H2O2 content and in the activities of POD and CAT between the two rootstocks were more obvious in the root system than in the leaves under NaCl stress. Thus, we suggest that the anti-oxidant capacity in the roots of grafted plants could affect the extent of salt injury in the shoots indirectly. The root is the direct organ when plants are exposed to saline medium and the rootstock roots of grafted cucumber seedlings may play an important role in limiting the transport of Na+ to the leaves (CitationZhu et al. 2008b). Thus, the roots of the Chaojiquanwang grafted plants may have an improved capacity to limit the transport of Na+ to the shoots and, as a result, the extent of injury in the shoots is alleviated.
In addition, under control conditions, the SOD and POD activities in the leaves were different depending on the rootstock genotypes. This result implies that the SOD and POD activities in the leaves of cucumber seedlings could be affected by rootstock even when unstressed, which is consistent with our previous reports that the SOD and ascorbate peroxidase (APX) activities in the leaves of cucumber were different depending on the rootstock genotypes, irrespective of salinity (CitationZhu et al. 2008a).
Scion effects
Although a great deal of work has been done to improve plant salt tolerance by grafting onto salt-tolerant rootstock, only a few studies have examined the effect of grafting with different scion genotypes. In the present study, the effects of scion on salt injury index, Na+ content in the leaves, H2O2 content and the activities of POD and CAT in the roots were significant. Under 100 mmol L−1 NaCl stress, the salt injury index and the Na+ content in the leaves was higher in grafted Jinchun No. 2 plants than in grafted Jinyu No. 1 plants when Figleaf Gourd was used as the rootstock. Thus, we conclude that grafting with a relatively salt-tolerant scion can partially improve the salt tolerance of cucumber seedlings. This is partially consistent with the results found in tomato (CitationMartinez-Rodriguez et al. 2008; CitationSanta-Cruz et al. 2002). However, the ANOVA showed that there were no significant effects of the scion on the dry weights of the roots and shoots, leaf area or leaf RWC in the present study (). This is partially inconsistent with our previous study on cucumber (CitationZhu et al. 2008b). Different salt sensitivities of cucumber scions may explain this discrepancy.
Moreover, differences in salt injury index, leaf Na+ content and in the activities of POD and CAT in the roots between grafted Jinyu No. 1 plants and grafted Jinchun No. 2 plants were inconsistent under salt stress when Chaojiquanwang and Figleaf gourd were used as rootstock, respectively. This may be associated with the regulation of some hormone signals produced in the rootstock roots. It has been suggested that the root is a major site for producing abscisic acid (ABA), and at least part of the ABA in the leaves is translocated from the roots (CitationThompson et al. 2007). Abscisic acid is defined as a stress hormone because of its rapid accumulation in response to stresses and its mediation of many stress responses that help plants to survive stress (CitationZhang et al. 2006). The anti-oxidant enzyme activities and most genes responding to drought and salt-stress have also been shown to be induced by ABA (CitationChandler and Robertson 1994; CitationLu et al. 2003). Thus, we conjecture that the differences in these physiological characteristics between grafted Jinyu No. 1 plants and grafted Jinchun No. 2 plants may be regulated by ABA produced in rootstock roots directly or indirectly. In addition, the roots are also a major site of cytokinin production, and cytokinins play a role as a root-to-shoot acropetal signal (CitationHirose et al. 2008). Hormones regulate many aspects of plant growth and development and control plant adaptation to environmental challenges through a complex network of signaling pathways. Therefore, further study into hormone metabolism is necessary. Furthermore, to better clarify the scion–rootstock relationship, the putative changes in some other possible signal elements, including calcium ion, polyamines and ROS, in the roots and shoots needs further research.
In conclusion, our results demonstrate that salt-tolerant rootstock has a primary effect in determining the salt tolerance of grafted cucumber seedlings under NaCl stress compared with the scion genotypes. Higher salt tolerance in grafted cucumber seedlings is attributed to lower leaf Na+ content and root H2O2 content, as well as to higher SOD, POD and CAT activities in the roots. In other words, grafting with salt-tolerant rootstock was found to increase the anti-oxidant capacity of cucumber seedlings and to limit the transport of Na+ to the leaves. As a result, the growth and salt tolerance of cucumber are promoted.
Acknowledgments
This work was supported by the National Basic Research Program of China (2009CB119000), the National Natural Science Foundation of China (30871738), the Key Project of the Chinese Ministry of Education (109113) and the Natural Science Foundation in Hubei Province (2008CDB081).
References
- Apel , K and Hirt , H . 2004 . Reactive oxygen species: metabolism, oxidative stress, and signal transduction . Annu. Rev. Plant Biol. , 55 : 373 – 399 .
- Ashraf , M . 2009 . Biotechnological approach of improving plant salt tolerance using antioxidants as markers . Biotechnol. Adv. , 27 : 84 – 93 .
- Badawi , GH , Yamauchi , Y Shimada , E . 2004 . Enhanced tolerance to salt stress and water deficit by overexpressing superoxide dismutase in tobacco (Nicotiana tabacum) chloroplasts . Plant Sci. , 166 : 919 – 928 .
- Brennan , T and Frenkel , C . 1977 . Involvement of hydrogen peroxide in the regulation of senescence in pear . Plant Physiol. , 59 : 411 – 416 .
- Chandler , PM and Robertson , M . 1994 . Gene expression regulated by abscisic acid and its relation to stress tolerance . Ann. Rev. Plant Physiol, Plant Mol. Biol. , 45 : 113 – 141 .
- Chen , G , Fu , X , Lips , SH and Sagi , M . 2003 . Control of plant growth resides in the shoot, and not in the root, in reciprocal grafts of flacca and wild-type tomato (Lycopersicon esculentum), in the presence and absence of salinity stress . Plant Soil , 256 : 205 – 215 .
- Cuartero , J , Bolarin , MC , Asins , MJ and Moreno , V . 2006 . Increasing salt tolerance in tomato . J. Exp. Bot. , 57 : 1045 – 1058 .
- Dasgan , HY , Aktas , H , Abak , K and Cakmak , I . 2002 . Determination of screening techniques to salinity tolerance in tomatoes and investigation of genotype responses . Plant Sci. , 163 : 695 – 703 .
- Dhindsa , RS , Plumb-Dhindsa , P and Thorpe , TA . 1981 . Leaf senescence: correlated with increased levels of membrane permeability and lipid peroxidation, and decreased levels of superoxide dismutase and catalase . J. Exp. Bot. , 32 : 93 – 101 .
- Estañ , MT , Martinez-Rodriguez , MM , Perez-Alfocea , F , Flowers , TJ and Bolarína , MC . 2005 . Grafting raises the salt tolerance of tomato through limiting the transport of sodium and chloride to the shoot . J. Exp. Bot. , 56 : 703 – 712 .
- Etehadnia , M , Waterer , D , Jong , HD and Tanino , KK . 2008 . Scion and rootstock effects on ABA-mediated plant growth regulation and salt tolerance of acclimated and unacclimated potato genotypes . J. Plant Growth Regul. , 27 : 125 – 140 .
- Flowers , TJ . 2004 . Improving crop salt tolerance . J. Exp. Bot. , 55 : 307 – 319 .
- Garcia-Sanchez , F , Perez-Perez , JG , Botia , P and Martinez , V . 2006 . The response of young mandarin trees grown under saline conditions depends on the rootstock . Eur. J. Agronomy , 24 : 129 – 139 .
- Goreta , S , Bucevic-Popovic , V , Selak , GV , Pavela-Vrancic , M and Perica , S . 2008 . Vegetative growth, superoxide dismutase activity and ion concentration of salt-stressed watermelon as influenced by rootstock . J. Agri Sci. , 146 : 695 – 704 .
- He , Y , Zhu , ZJ , Yang , J , Ni , Xl and Zhu , B . 2009 . Grafting increases the salt tolerance of tomato by improvement of photosynthesis and enhancement of antioxidant enzymes activity . Environ. Exp. Bot. , 66 : 270 – 278 .
- Hirose , N , Takei , K , Kuroha , T , Kamada-Nobusada , T , Hayashi , H and Sakakibara , H . 2008 . Regulation of cytokinin biosynthesis, compartmentalization and translocation . J. Exp. Bot. , 59 : 75 – 83 .
- Hoagland , DR and Arnon , DS . 1950 . The water culture method for growing plants without soil . Calif. Agric. Exp. Stat. Circ. , 347 : 1 – 32 .
- Huang , Y , Tang , R , Cao , QL and Bie , ZL . 2009 . Improving the fruit yield and quality of cucumber by grafting onto the salt tolerant rootstock under NaCl stress . Sci. Hort , 122 : 26 – 31 .
- Lee , JM . 1994 . Cultivation of grafted vegetables I. Current status, grafting methods, and benefits . Hort Science , 29 : 235 – 239 .
- Liu , YJ . 2000 . Experiment Technology of Plant Biochemistry and Physiology , 146 – 147 . Beijing : Agriculture of China Press .
- López-Gómez , E , San Juan , MA , Diaz-Vivancos , P , Mataix Beneyto , J , García-Legaz , MF and Hernández , JA . 2007 . Effect of rootstocks grafting and boron on the antioxidant systems and salinity tolerance of loquat plants (Eriobotrya japonicaLindl.) . Environ. Exp. Bot. , 60 : 151 – 158 .
- Lu , SY , Guo , ZF and Peng , XX . 2003 . Effects of ABA and S-3307 on drought resistance and antioxidative enzyme activity of turfgrass . J.Hort. Sci. Biotechnol. , 78 : 663 – 666 .
- Martinez-Rodriguez , MM , Estañ , MT Moyano , E . 2008 . The effectiveness of grafting to improve salt tolerance in tomato when an “excluder” genotype is used as scion . Environ. Exp. Bot. , 63 : 392 – 401 .
- Mickelbart , MV and Arpaia , ML . 2002 . Rootstock influence changes in ion concentrations, growth, and photosynthesis of “Hass” avocado trees in response to salinity . J. Amer. Soc. Hort. Sci. , 127 : 649 – 655 .
- Mittler , R . 2002 . Oxidative stress, antioxidants and stress tolerance . Trends Plant Sci. , 7 : 405 – 410 .
- Mittova , V , Tal , M , Volokita , M and Guy , M . 2002 . Salt stress induces up-regulation of an efficient chloroplast antioxidant system in the salttolerant wild tomato species Lycopersicon pennelliibut not in the cultivated species . Physiol. Plant. , 115 : 393 – 400 .
- Parida , AK and Das , AB . 2005 . Salt tolerance and salinity effects on plants: a review . Ecotoxicol. Environ. Safety , 60 : 324 – 349 .
- Romero , L , Belakbir , A , Ragala , L and Ruiz , JM . 1997 . Response of plant yield and leaf pigments to saline conditions: effectiveness of different rootstocks in melon plants (Cucumis meloL.) . Soil Sci. Plant Nutr. , 43 : 855 – 862 .
- Rout , NP and Shaw , BP . 2001 . Salt tolerance in aquatic macrophytes: possible involvement of the antioxidative enzymes . Plant Sci. , 160 : 415 – 423 .
- Ruiz , JM , Ríos , JJ , Rosales , MA , Rivero , RM and Romero , L . 2006 . Grafting between tobacco plants to enhance salinity tolerance . J. Exp. Bot. , 163 : 1229 – 1237 .
- Santa-Cruz , A , Martinez-Rodriguez , MM , Perez-Alfocea , F , Romero-Aranda , R and Bolarin , MC . 2002 . The rootstock effect on the tomato salinity response depends on the shoot genotype . Plant Sci. , 162 : 825 – 831 .
- Saqib , M , Akhtar , J and Qureshi , RH . 2005 . Na+exclusion and salt resistance of wheat (Triticum aestivum) in saline-waterlogged conditions are improved by the development of adventitious nodal roots and cortical root aerenchyma . Plant Sci. , 169 : 125 – 130 .
- Shaterian , J , Georges , F , Hussain , A , Waterer , D , De Jong , H and Tanino , KK . 2005a . Root to shoot communication and abscisic acid in calreticulin (CR) gene expression and salt-stress tolerance in grafted diploid potato clones . Environ. Exp. Bot. , 53 : 323 – 332 .
- Shaterian , J , Waterer , D , De Jong , H and Tanino , KK . 2005b . Differential stress responses to NaCl salt application in early-and late maturing diploid potato (Solanumsp.) clones . Environ. Exp. Bot. , 54 : 202 – 212 .
- Thompson , AJ , Mulholland , BJ Jackson , AC . 2007 . Regulation and manipulation of ABA biosynthesis in roots . Plant Cell Environ. , 30 : 67 – 78 .
- Trajkova , F , Papadantonakis , N and Savvas , D . 2006 . Comparative effects of NaCl and CaCl2salinity on cucumber grown in a closed hydroponic system . HortScience. , 41 : 437 – 441 .
- Weatherley , PE . 1950 . Studies in water relations of cotton plants. I. The field measurement of water deficits in leaves . New Phytol. , 49 : 81 – 97 .
- Wei , GP , Yang , LF , Zhu , YL and Chen , G . 2009 . Changes in oxidative damage, antioxidant enzyme activities and polyamine contents in leaves of grafted and non-grafted eggplant seedlings under stress by excess of calcium nitrate . Sci. Horti. , 120 : 443 – 451 .
- Xu , SL , Chen , QY , Chen , XQ and Li , H . 2006 . Relationship between grafted muskmelon growth and polyamine and polyamine oxidase activities under salt stress . J. Fruit. Sci. , 23 : 260 – 265 .
- Zhang , JH , Jia , WS , Yang , JC and Ismail , AM . 2006 . Role of ABA in integrating plant responses to drought and salt stresses . Field Crops Res. , 97 : 111 – 119 .
- Zhang , QF , Li , YY , Pang , CH , Lu , CM and Wang , BS . 2005 . NaCl enhances thylakoid-bound SOD activity in the leaves of C3halophyte Suaeda salsa L . Plant Sci. , 168 : 423 – 430 .
- Zhang , GW , Liu , ZL , Zhou , JG and Zhu , YL . 2008 . Effects of Ca(NO3)2stress on oxidative damage, antioxidant enzymes activities and polyamine contents in roots of grafted and non-grafted tomato plants . Plant Growth Regul. , 56 : 7 – 19 .
- Zhu , JK . 2001 . Plant salt tolerance . Trends Plant Sci. , 6 : 66 – 71 .
- Zhu , J , Bie , ZL and Huang , Y . 2008a . Effects of grafting with different rootstocks on the growth, osmotic adjustment and anti-oxidant enzyme activities of cucumber seedlings under salt stress . J. Shanghai Jiaotong Univ , 26 : 393 – 397 . in Chinese with English summary
- Zhu , J , Bie , ZL , Huang , Y and Han , XY . 2008b . Effect of grafting on the growth and ion concentrations of cucumber seedlings under NaCl stress . Soil Sci. Plant Nutr. , 54 : 895 – 902 .
- Zhu , J , Bie , ZL and Li , YN . 2006 . Evaluation of salt resistance of cucumber at seed germination and rootstock-seedling stages . Sci. Agri. Sin. , 39 : 772 – 778 .
- Zhu , J , Bie , ZL and Li , YN . 2008c . Physiological and growth responses of two different salt-sensitive cucumber cultivars to NaCl stress . Soil Sci. Plant Nutr. , 54 : 400 – 407 .