Abstract
The present study describes succession in the abundances of ammonia-oxidizing archaea (AOA) and ammonia-oxidizing bacteria (AOB) in the upland and flooded bulk soils of a Japanese rice paddy field over 2 years using a quantitative polymerase chain reaction of both crenarchaeotal and betaproteobacterial ammonia monooxygenase alpha subunit (amoA) genes. A marked increase in the abundance of AOA amoA gene was observed in upland bulk soil after plowing, drainage and rice harvesting. A marked increase was also observed in the abundance of AOB amoA gene after plowing. The abundances of both AOA and AOB amoA genes in flooded bulk soil decreased immediately after flooding. During the middle period of flooding, the abundance of AOA amoA gene increased slightly in the flooded bulk soil. As the flooding subsided, the abundance of AOA amoA gene decreased, whereas that of AOB amoA gene increased. The AOA amoA gene sequences were affiliated with two phylogenetic clusters previously found in marine and soil environments. Both Nitrosospira-like and Nitrosomonas-like clones were detected. Our results revealed that there was a difference in the succession of abundances between AOA and AOB amoA genes in Japanese bulk paddy soil.
Introduction
Ammonia-oxidizing microorganisms (AOM) play an important role in the nitrification of rice paddy soil (CitationKeeney and Sahrawat 1986; CitationRao et al. 1984). The AOM catalyze the conversion of ammonia (NH3) to nitrite (NO2 −), which is then converted to nitrate (NO3 −) by nitrite-oxidizing bacteria. Ammonia-oxidizing bacteria (AOB) are affiliated with the Betaproteobacteria and Gammaproteobacteria. In terrestrial soil ecosystems, oxidation of ammonia is believed to be mainly carried out by AOB within the Betaproteobacteria (CitationStephen et al. 1996). Populations of AOB have been investigated in rice paddy soil (CitationBowatte et al. 2006; CitationNicolaisen et al. 2004) and in the root environment of rice (CitationBriones et al. 2002; CitationIkenaga et al. 2003; CitationRotthauwe et al. 1997) via molecular ecological approaches based on the 16S rDNA and ammonia monooxygenase alpha-subunit (amoA) gene responsible for catalyzing the first step of ammonia oxidation. Recent studies have demonstrated the existence of ammonia-oxidizing archaea (AOA) within the Crenarchaeota in soil and marine environments using metagenomic and cultivation approaches (CitationHallam et al. 2006; CitationKönneke et al. 2005; CitationSchleper et al. 2005; CitationTreusch et al. 2005; CitationVenter et al. 2004). In addition, several studies based on the crenarchaeotal amoA gene have revealed the numerical dominance of AOA over AOB in both terrestrial and marine environments, suggesting that oxidation of ammonia may be carried out by both AOA and AOB in natural ecosystems, such as terrestrial soil, freshwater sediments and marine water columns and sediments (CitationAdair and Schwartz 2008; CitationChen et al. 2008; CitationHe et al. 2007; CitationHerrmann et al. 2009; CitationLe Roux et al. 2008; CitationLeininger et al. 2006; CitationNakagawa et al. 2007; CitationSchauss et al. 2009; CitationShen et al. 2008; CitationWuchter et al. 2006). However, to date no study has investigated changes in the relative abundance of AOA and AOB in paddy field soil.
Nitrogen fertilizer is of great importance in raising the rice grain yield in paddy fields (CitationBouldin 1986; CitationCassman et al. 1993). Ammonium (NH4 +) in the soil is assimilated from rice roots as a major source of nitrogen nutrients (CitationKeeney and Sahrawat 1986; CitationWang et al. 1993). In contrast, it has been suggested that rice roots may also assimilate significant amounts of nitrate formed through nitrification in the rhizosphere (CitationKronzucker et al. 2000). Compared to ammonium, which is usually linked to the soil matrix, NO3 −- N is easily lost from paddy fields through leaching that results both from the mobility of nitrate within the soil and from denitrification (CitationGeorge et al. 1993; CitationKeeney and Sahrawat 1986; CitationTripathi et al. 1997). As the soil during rice growth is underwater, oxygen is rapidly depleted a few millimeters beneath the soil–water interface (CitationKimura 2000; CitationNicolaisen et al. 2004; CitationRevsbech et al. 1999). Accordingly, the nitrification rate and cell number of AOB in the thin surface soil were higher than those in the rice rhizosphere and bulk soil in a high-yield irrigated Philippine rice field (CitationNicolaisen et al. 2004). Furthermore, it has been suggested that AOB in the rhizosphere of rice roots and on the root surface use oxygen leaked from the rice roots within the anoxic bulk soil (CitationBriones et al. 2002). Although nitrification is involved in the nitrogen dynamics in paddy field soil, the mechanism of ammonia oxidation by AOA in paddy field soil is still unclear.
In the present study, we examine succession in the abundances of crenarchaeotal and betaproteobacterial amoA genes and the community compositions of AOA and AOB over 2 years in upland and flooded soils of a Japanese rice paddy field. Moreover, we compare changes in the abundance of both amoA genes and in the concentrations of NH4 + and NO3 − in the soil.
Materials and methods
Sample collection
Soil samples were taken from a rice (Oryza sativa L.) paddy at the Center for Natural Environment Science, College of Bioresource Sciences, Nihon University, Fujisawa, Kanagawa, Japan. Field management in 2007 and 2008 consisted of plowing on 3 and 30 April, basal fertilization (100 kg N ha−1 of organic fertilizer [earthworm casts] and 100 kg N ha−1 [73 kg NH4 +-N ha−1], 100 kg P ha−1 and 200 kg K2O ha−1of compound fertilizer) and flooding on 13, 15 and 16 May, puddling on 20 and 22 May, transplanting on 22 and 26 May, drainage on 8 and 11 September, and rice harvesting (except for 5-cm stubble bases) on 18 September and 2 October (). The soil type was a dystric-silic andisol. Soil temperature was measured with a portable thermometer. The pH of in situ flooded soil was measured using a portable pH meter (D-55; HORIBA, Kyoto, Japan). Bulk soil was defined as soil containing fine roots at a depth of between 0 and approximately 5 cm, and was roughly collected at three different points in the same field with a sterile spatula between the rice plants during rice growth. Three replicate bulk soil samples were placed in sterile plastic tubes (50 mL). After centrifugation (5,800 g, 20 min) of the flooded bulk soil during rice growth, the supernatants were removed. Soil samples for the chemical analyses and DNA extraction were stored at −20°C.
Chemical analysis
Slurries (1:2.5 mass ratio of soil and deionized water) were ultrasonicated for 30 s. After filtration of the water containing soil, we measured the concentrations of nitrite and nitrate with an ion chromatography system-1,000 attached to an UltiMate 3,000 Variable Wavelength Detector (Dionex, Sunnyvale, CA, USA). The quantitative limits of NO2 − and NO3 − concentrations were 0.1 and 0.2 μg (wet soil g)−1, respectively. After shaking 0.15 g of soil mixed with 1.5 mL of ammonium extraction solution (1 mol L–1 KCl and 0.01 mol L–1 HCl) for 30 min, the concentration of ammonium in the soil was determined colorimetrically by a modified indophenol reaction (CitationKandeler and Gerber 1988). The quantitative limit of NH4 + concentration was 3.6 μg (wet soil g)−1.
Amplification of the amoA gene and cloning
Nucleic acids were extracted from 0.5 g of wet soils with an ISOIL for Beads Beating Kit (Nippon Gene, Toyama, Japan) as previously described (CitationNakaya et al. 2009). Nucleic acids extracted in 20 μL of Tris–ethylenediaminetetraacetic acid buffer were stored at −20°C until the polymerase chain reaction (PCR). The total DNA concentration was measured with a Qubit Fluorometer and Quant-iT dsDNA HS Assay kit (Invitrogen, Carlsbad, CA, USA) to exclude the possibility that quantification was biased by differences in the effect of DNA extraction among the soil samples. For phylogenetic analysis of AOA amoA genes, partial amoA gene fragments were amplified with TaKaRa EX Taq DNA polymerase (Takara Bio, Otsu, Japan) using template DNA extracted from soils collected on 7 April, 6 June, 28 August, 28 November 2007 and 27 February, 23 April and 9 May 2008. For phylogenetic analysis of AOB amoA genes, template DNA extracted from soils collected on 28 August, 28 November 2007 and 27 February, 23 April and 9 May 2008 was used. The total volume of the PCR reaction was 50 μL, containing 5 μL of 10× EX Taq buffer, 250 μmol L–1 each of deoxynucleoside triphosphate, 25 pmol of each primer and 1.25 U of EX Taq DNA polymerase. The nucleotide primers were amoA19F (5′-ATGGTCTGGCTWAGACG-3′) (CitationLeininger et al. 2006) and amo643R (5′-TCCCACTTWGACCARGCGGCCATCCA-3′) (CitationTreusch et al. 2005) for the crenarchaeotal amoA gene, and amoA-1F (5′-GGGGTTTCTACTGGTGGT-3′) and amoA-2R (5′-CCCCTCKGSAAAGCCTTCTTC-3′) for the betaproteobacterial amoA gene (CitationRotthauwe et al. 1997). The following protocol was used for crenarchaeotal amoA: an initial denaturation step at 95°C for 5 min and then 25 cycles at 95°C for 1 min, 55°C for 1 min and 72°C for 40 s, with a final 10-min extension at 72°C. For betaproteobacterial amoA, there was an initial denaturation step at 94°C for 5 min and then 35 cycles at 94°C for 30 s, 57°C for 1 min and 72°C for 45 s, with a final extension at 72°C for 5 min. The PCR products were visualized on an agarose gel stained with ethidium bromide and extracted with a QIAGEN II Gel Extraction Kit (QIAGEN, Valencia, CA, USA). Amplified gene fragments were cloned with a TOPO TA PCR cloning kit (Invitrogen). White colonies were transferred to fresh Luria-Bertani (LB) broth agar plates with kanamycin, grown overnight at 37°C and amplified with the vector-specific primers M13F and M13R. After the PCR products were electrophoresed again in an agarose gel to check the length of the bands, amplicons were purified with a QIAquick PCR purification kit (QIAGEN) prior to sequencing.
Figure 1 Successions in (A) soil temperature, (B) abundance of crenarchaeotal and betaproteobacterial amoA genes (n = 3) in soil and (C) NO3− and NH4+ concentrations (n = 3) in soil. a, plowing; b, basal fertilization and flooding; c, puddling; d, transplanting; e, drainage; f, rice harvesting. Error bars are standard deviations (n = 3). Asterisks above the symbols from April 2007 indicate that the values were estimated as wet weight soil.
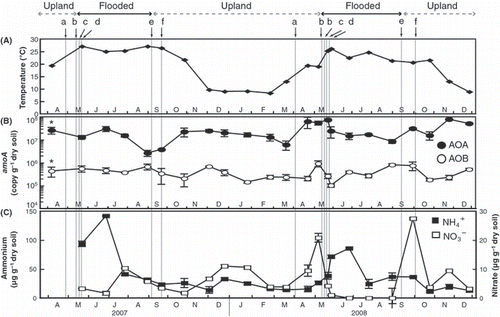
Fluorescent quantitative polymerase chain reaction
Crenarchaeotal and betaproteobacterial amoA genes in the soils were quantified by real-time PCR in a Light-Cycler system 350S (Roche Diagnostics, Mannheim, Germany) with SYBR Green I as a fluorescent dye using the primers amoA19F/amo643R and amoA-1F/amoA-2R. Each reaction was carried out in a 20-μL volume containing 2 μL of template DNA solution, 0.2 μg μL−1 of bovine serum albumin, 0.2 μmol L–1 of each primer and 10 μL of SYBR Premix Ex Taq (Perfect Real Time) (Takara Bio). The cycling conditions were as follows: for the crenarchaeotal amoA gene there was an initial denaturation step at 95°C for 30 s and then 30 cycles at 95°C for 15 s, 55°C for 15 s and 72°C for 30 s. The fluorescence intensity was measured at 80°C. For the betaproteobacterial amoA gene there was an initial denaturation step at 95°C for 30 s followed by 40 cycles of 95°C for 15 s, 57°C for 15 s and 72°C for 30 s. The fluorescence intensity was measured at 80°C. After each run, the amplicon was visualized on an agarose gel to confirm specific product bands of the expected size. The standard curves consisted of a dilution series (2.7 × 103–2.7 × 108 copies μL−1) of a plasmid (pCR2.1-TOPO; Invitrogen) containing the amoA of clone PFS_G34614 obtained in the present study and generated with primers amoA19F/amo643R, and with a dilution series (8.3 × 101–8.3 × 108 copies μL−1) of a plasmid containing the amoA of Nitrosospira multiformis ATCC25196 generated with primers amoA-1F/amoA-2R.
Sequencing, phylogenetic analysis and rarefaction analysis
The M13F/M13R PCR products were sequenced with a BigDye v.3.1 sequencing kit (Applied Biosystems, Foster City, CA, USA) and vector primers M13F and M13R on a Model 3130×l DNA sequencer (Applied Biosystems). Similarities in the amoA sequences were investigated in the databases of the National Center for Biotechnology Information and the DNA Data Bank of Japan using the BLAST program (CitationAltschul et al. 1997). Sequence similarity was determined with mega4 software (CitationTamura et al. 2007). Operational taxonomic units (OTUs) were defined as sequence groups in which the sequences differed by ≤3% from the nucleotide sequences. Phylogenetic inferences were calculated from confidently aligned regions of homologous proteins and nucleic acid sequences using the mega4 software. Neighbor-joining bootstrap values were calculated from 1,000 resampled datasets using mega4. Rarefaction analysis was carried out using Analytic Rarefaction 1.3 (http://www.uga.edu/strata/software/index.html) to confirm the shape of the rarefaction curve.
Statistical analysis
One-way anovas and Tukey’s tests were used to determine significant differences.
Nucleotide sequence accession numbers
The amoA gene sequences were submitted to DDBJ (DNA Data Bank of Japan)/EMBL (European Molecular Biology Laboratory)/GenBank and have been assigned the following accession numbers: AB516240 to AB516282.
Results and discussion
Changes in the abundances of AOA and AOB amoA genes
The abundance of the AOA amoA gene in bulk soil of the paddy field ranged from 2.7 × 106 to 7.9 × 107 (copy [gram dry soil]−1), whereas that of the AOB amoA gene ranged from 1.0 × 105 to 9.3 × 105 (copy [gram dry soil]−1) (). We confirmed that the result did not depend on any difference in the effect of DNA extraction among the soil samples. The abundances of AOA amoA genes were significantly higher than those of AOB amoA genes throughout the present study (P < 0.05). There was a difference in the succession of abundances between AOA and AOB amoA genes. The AOA : AOB amoA gene ratios changed from 4 to 346 over the present study. The numerical dominance of AOA over AOB was in agreement with previous studies of agricultural soils (CitationChen et al. 2008; CitationHe et al. 2007; CitationJia and Conard 2009; CitationLeininger et al. 2006; CitationShen et al. 2008).
Figure 2 Phylogenetic tree based on crenarchaeotal amoA gene sequences (579 bp) determined by neighbor-joining analysis. Boldface type indicates representative amoA clones obtained in the present study. Numbers and symbols in parentheses denote the total number of clones detected from soils on 7 April, 6 June, 28 August, 28 November 2007, and 27 February, 23 April and 9 May 2008. The scale bar represents an estimated sequence divergence of 5%. Numbers at the nodes indicate the bootstrap values (≥50%).
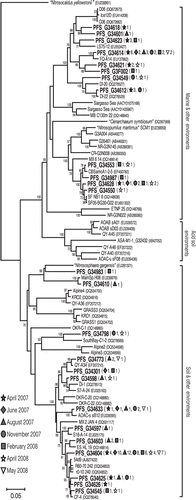
A marked increase in the abundance of AOA amoA was observed in upland bulk soil in October 2007, and in April, September and November 2008 (). This phenomenon occurred after plowing, drainage and rice harvesting. On the contrary, there was a marked increase in the abundance of AOB amoA on 9 May 2008, which arose after an increase in the abundance of AOA amoA as a result of plowing (). It has been previously hypothesized that AOA are influenced more than AOB by exudation (e.g. oxygen, carbon dioxide) in the paddy soil (CitationChen et al. 2008). It is therefore likely that the marked increase in the abundance of AOA amoA rather than AOB amoA might be attributed to a higher affinity of AOA for oxygen or carbon dioxide in upland bulk soil. A marked increase in the concentration of NO3 − was observed after plowing in April and on 9 May 2008 (), which could be attributed to an increase in soil temperature, oxygen supply in the soil and ammonification of organic nitrogen. The concentration of NO3 − and AOB amoA copy numbers in upland bulk soil were roughly correlated (r = 0.575, P = 0.000). There was also a rough correlation between NO3 − and AOA copy numbers in upland bulk soil, although this correlation was not significant (r = 0.296, P = 0.068). Although microbial ammonia oxidation and carbon dioxide assimilation by AOB rather than AOA in agricultural soil has recently been reported (CitationJia and Conard 2009), it appears that both AOA and AOB affected the nitrification in upland soil in the present study.
The abundances of both AOB and AOA amoA genes in flooded bulk soil decreased immediately after flooding on 13 and 16 May 2008, respectively (). It is well known that nitrification occurs in a thin surface layer of soil covered by floodwater in irrigated paddy fields during rice growth (CitationKimura 2000; CitationNicolaisen et al. 2004; CitationRevsbech et al. 1999). It is therefore likely that the marked decrease in the abundance of both AOA and AOB amoA genes might reasonably be attributed to the rapid depletion of oxygen in the bulk soil after flooding. In the middle period of flooding, the abundance of the AOA amoA gene increased slightly in the flooded bulk soil (). However, at the end of flooding, the abundances of the AOA amoA gene declined, whereas those of AOB amoA gene increased. The concentration of NO3 − in the flooded bulk soil rose in July 2007 and 2008 after a decrease in the concentration of NH4 + in the soil (). The supernatant of the soil after centrifugation contained 11 μmol L–1 NO3 − in July 2008. However, no significant increase in the abundance of either AOA or AOB amoA in flooded bulk soil was observed. It has been reported that AOA are more numerically dominant than AOB in the rhizosphere of plant roots (CitationChen et al. 2008; CitationHerrmann et al. 2009). This indicates the need to investigate the relationship between the NO3 − concentration and the abundance of the AOA amoA gene in the rhizosphere of rice roots and in the surface layer of soil to determine whether AOA are involved in nitrification in flooded soil.
There was a difference in the succession of abundances between AOA and AOB amoA genes in flooded bulk soil in the present study (). A recent study in agricultural soil has demonstrated that AOB play an important role in ammonia oxidation and carbon dioxide assimilation rather than AOA, despite the numerical dominance of crenarchaeotal amoA over bacterial amoA genes (CitationShen et al. 2008). In addition, it has been reported that the abundance and diversity of AOA do not differ significantly among different fertilization treatments (CitationJia and Conard 2009). Thus, further studies are needed to clarify how fertilization affects the activity of AOA in flooded paddy soil.
Table 1 Frequency changes in ammonia-oxidizing archaea and ammonia-oxidizing bacteria clone libraries obtained from bulk paddy field soil
Phylogeny of ammonia-oxidizing archaea
The AOA amoA clone libraries of bulk soil were generated in April, June, August and November 2007, and in February, April and 9 May 2008. Of the 122 individual AOA amoA gene sequences obtained from the bulk soil, 78 clones fell into a large cluster “soil and other environments” with sequences from soil samples, whereas 44 clones fell into a large cluster “marine and other environments” with sequences from marine sediment and water column samples (). We obtained a total of 25 OTUs (based on a 3% cutoff) from these 122 individual AOA amoA gene sequences. The rarefaction curves revealed the richness of the AOA amoA genes (data not shown). The most abundant AOA amoA clone, PFS_G34604, in “soil and other environments” was found to be closely related to the environmental clone 54d9 derived from German soil (CitationTreusch et al. 2005) and to the clone South Bay-CR-16 derived from South San Francisco Bay sediment in the USA (CitationPark et al. 2006). In contrast, the clone PFS_G34614 had a relatively higher frequency than other clones in “marine and other environments”. Thus, the occurrence of both “marine and other environments” and “soil and other environments” in the present study was in agreement with the results of previous studies in freshwater sediments (CitationHerrmann et al. 2009). There was a difference in the community composition of AOA depending on the sampling date (). The frequencies of AOA clones affiliated with “soil and other environments” were higher than those of “marine and other environments” in July and August 2007, and in May 2008.
Phylogeny of ammonia-oxidizing bacteria
The AOB amoA clone libraries of bulk soil were generated in August and November 2007, and in February, April and 9 May 2008. Of the 116 individual amoA gene sequences obtained from the bulk soil, 97 clones were affiliated with the genus Nitrosospira and 19 were affiliated with the genus Nitrosomonas (). We obtained a total of 18 OTUs (based on a 3% cutoff) from these 116 individual AOB amoA gene sequences. The most abundant amoA clone, PFS_G34877, within the genus Nitrosospira was found to be closely related to the environmental clone RR45-2 derived from rice roots (CitationRotthauwe et al. 1997). The other most abundant amoA clone, PFS_G34712, obtained on 9 May 2008 was closely related to a strain of Nitrosospira sp. PJA1 isolated from the rhizosphere of barley plants (CitationSatoh et al. 2003). In contrast, the clone PFS_G34876 within the genus Nitrosomonas was related to the clone S38 derived from Chinese flooded rice soil (CitationWang et al. 2009). The community composition of AOB differed depending on the sampling date (). Although the frequencies of clones affiliated with the genus Nitrosospira reached more than 90% in February, April and May 2008, the frequencies of clones affiliated with the genus Nitrosomonas increased in August and November 2007. It has been previously reported that environmental clones within the genus Nitrosomonas rather than Nitrosospira are predominantly detected in paddy field soil treated with high N fertilizer and on the surface of rice roots (CitationBriones et al. 2002; CitationNicolaisen et al. 2004). Further studies, however, are needed to clarify the major determinant of AOB community structure in paddy soil.
Figure 3 Phylogenetic tree based on betaproteobacterial amoA gene sequences (450 bp) determined by neighbor-joining analysis. Boldface type indicates representative amoA clones obtained in the present study. Numbers and symbols in parentheses denote the total number of clones detected from soils on 28 August, 28 November 2007, and 27 February, 23 April and 9 May 2008. The scale bar represents an estimated sequence divergence of 5%. Numbers at the nodes indicate the bootstrap values (≥50%).
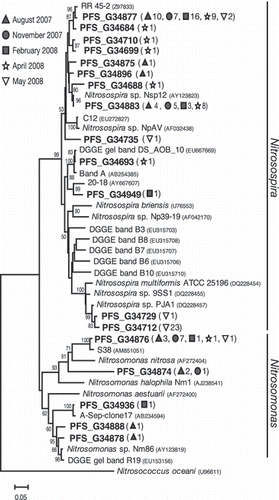
Conclusions
The present study revealed that there was a difference in the succession of abundances between AOA and AOB amoA genes in Japanese bulk paddy soil. This result suggests the need to investigate both AOA and AOB in Japanese paddy fields to provide a comprehensive understanding of nitrification in the flooded and upland soils of Japanese rice paddies.
Acknowledgments
We wish to thank the staff of the General Research Institute (Nihon University) for their support with the sequencing. This study was supported by a Nihon University Grant for Assistants and Young Researchers 2008 (to T. Nakagawa).
References
- Adair , KL and Schwartz , E . 2008 . Evidence that ammonia-oxidizing archaea are more abundant than ammonia-oxidizing bacteria in semiarid soils of Northern Arizona, USA . Microb. Ecol. , 56 : 420 – 426 .
- Altschul , SF , Madden , TL Schäfer , AA . 1997 . Gapped BLAST and PSI-BLAST: a new generation of protein database search programs . Nucleic Acids Res. , 25 : 3389 – 3402 .
- Bouldin , DR . 1986 . The chemistry and biology of flooded soils in relation to the nitrogen economy in rice fields . Fertil. Res. , 9 : 1 – 14 .
- Bowatte , S , Jia , Z , Ishihara , R , Nakajima , Y , Asakawa , S and Kimura , M . 2006 . Molecular analysis of the ammonia oxidizing bacterial community in the surface soil layer of a Japanese paddy field . Soil Sci. Plant Nutr. , 52 : 427 – 431 .
- Briones , AM , Okabe , S , Umemiya , Y , Ramsing , N-B , Reichardt , W and Okuyama , H . 2002 . Influence of different cultivars on populations of ammonia-oxidizing bacteria in the root environment of rice . Appl. Environ. Microbiol. , 68 : 3067 – 3075 .
- Cassman , KG , Kropf , MJ , Gaunt , J and Peng , S . 1993 . Nitrogen use efficiency of rice reconsidered: what are the key constraints? . Plant Soil , 155/156 : 359 – 362 .
- Chen , X-P , Zhu , Y-G , Xia , Y , Shen , J-P and He , J-Z . 2008 . Ammonia-oxidizing archaea: important players in paddy rhizosphere soil? . Environ. Microbiol. , 10 : 1978 – 1987 .
- George , T , Ladha , JK , Buresh , RJ and Garrity , DP . 1993 . Nitrate dynamics during the aerobic soil phase in lowland rice-based cropping systems . Soil Sci. Soc. Am. J. , 57 : 1526 – 1532 .
- Hallam , SJ , Mincer , TJ Schleper , C . 2006 . Pathways pf carbon assimilation and ammonia oxidation suggested by environmental genomic analyses of marine Crenarchaeota . PLoS Biol. , 4 : 520 – 536 .
- He , JZ , Shen , JP Zhang , LM . 2007 . Quantitative analyses of the abundance and composition of ammonia-oxidizing bacteria and ammonia-oxidizing archaea of a Chinese upland red soil under long-term fertilization practices . Environ. Microbiol. , 9 : 2364 – 2374 .
- Herrmann , M , Saunders , AM and Schramm , A . 2009 . Effect of lake trophic status and rooted macrophytes on community composition and abundance of ammonia-oxidizing prokaryotes in freshwater sediments . Appl. Environ. Microbiol. , 77 : 3127 – 3136 .
- Ikenaga , M , Asakawa , S , Muraoka , Y and Kimura , M . 2003 . Phylogenetic study on CTO primer amplified ammonia oxidizing bacteria and β-Proteobacteria associated with rice roots grown in a flooded paddy soil . Soil Sci. Plant Nutr. , 49 : 719 – 727 .
- Jia , Z and Conard , R . 2009 . Bacteriarather than Archaeadominate microbial ammonia oxidation in an agricultural soil . Environ. Microbiol. , 11 : 1658 – 1671 .
- Kandeler , E and Gerber , H . 1988 . Short-term assay of soil urease activity using colorimetric determination of ammonium . Biol. Fertil. Soils , 6 : 68 – 72 .
- Keeney , DR and Sahrawat , KL . 1986 . Nitrogen transformations in flooded rice soils . Fertil. Res. , 9 : 15 – 38 .
- Kimura , M . 2000 . “ Anaerobic microbiology in waterlogged rice fields ” . In Soil Biochemistry , Edited by: Bollag , J and Stotzky , G . 35 – 138 . New York : Marcel Dekker .
- Könneke , M , Bernhard , AE , de la Torre , JR , Walker , CB , Waterbury , JB and Stahl , DA . 2005 . Isolation of an autotrophic ammonia-oxidizing marine archaeon . Nature , 437 : 543 – 546 .
- Kronzucker , HJ , Glass , ADM , Siddiqi , MY and Kirk , GJD . 2000 . Comparative kinetic analysis of ammonium and nitrate acquisition by tropical lowland rice: implications for rice cultivation and yield potential . New Phytol. , 145 : 471 – 476 .
- Le Roux , X , Poly , F Currey , P . 2008 . Effects of aboveground grazing on coupling among nitrifier activity, abundance and community structure . ISME J , 2 : 221 – 232 .
- Leininger , S , Urich , T Schloter , M . 2006 . Archaea predominate among ammonia-oxidizing prokaryotes in soils . Nature , 442 : 806 – 809 .
- Nakagawa , T , Mori , K , Kato , C , Takahashi , R and Tokuyama , T . 2007 . Distribution of cold-adapted ammonia-oxidizing microorganisms in the deep-ocean of the northeastern Japan Sea . Microbes Environ. , 22 : 365 – 372 .
- Nakaya , A , Onodera , Y Nakagawa , T . 2009 . Analysis of ammonia monooxygenase and archaeal 16S rRNA gene fragments in nitrifying acid-sulfate soil microcosms . Mirobes Environ. , 24 : 168 – 174 .
- Nicolaisen , MH , Risgaard-Petersen , N , Revsbech , NP , Reichardt , W and Ramsing , NB . 2004 . Nitrification-denitrification dynamics and community structure of ammonia oxidizing bacteria in a high yield irrigated Philippine rice field . FEMS Microbiol. Ecol. , 49 : 359 – 369 .
- Park , H-D , Wells , GF , Bae , H , Criddle , CS and Francis , CA . 2006 . Occurrence of ammonia-oxidizing archaea in wastewater treatment plant bioreactors . Appl. Environ. Microbiol. , 72 : 5643 – 5647 .
- Rao , PSC , Jessup , RE and Reddy , KR . 1984 . Simulation of nitrogen dynamics in flooded soils . Soil Sci. , 138 : 54 – 62 .
- Revsbech , NP , Pedersen , O , Reichardt , W and Briones , A . 1999 . Microsensor analysis of oxygen and pH in the rice rhizosphere under field and laboratory conditions . Biol. Fertil. Soils , 29 : 379 – 385 .
- Rotthauwe , JH , Witzel , KP and Liesack , W . 1997 . The ammonia monooxygenase structural gene amoAas a functional marker: molecular fine-scale analysis of natural ammonia-oxidizing populations . Appl. Environ. Microbiol. , 63 : 4704 – 4712 .
- Satoh , K , Yanagida , T Isobe , K . 2003 . Effect of root exudates on growth of newly isolated nitrifying bacteria from barley rhizoplane . Soil Sci. Plant Nutr. , 49 : 757 – 762 .
- Schauss , K , Focks , A Leininger , S . 2009 . Dynamics and functional relevance of ammonia-oxidizing archaea in two agricultural soils . Environ. Microbiol. , 11 : 446 – 456 .
- Schleper , C , Jurgens , G and Jonuscheit , M . 2005 . Genomic studies of uncultivated archaea . Nat. Rev. Microbiol. , 3 : 479 – 488 .
- Shen , JP , Zhang , LM , Zhu , YG , Zhang , JB and He , JZ . 2008 . Abundance and composition of ammonia-oxidizing bacteria and ammonia-oxidizing archaea communities of an alkaline sandy loam . Environ. Microbiol. , 10 : 1601 – 1611 .
- Stephen , JR , McCaig , AE , Smith , Z , Prosser , JI and Embley , TM . 1996 . Molecular diversity of soil and marine 16S rRNA gene sequences related to beta-subgroup ammonia-oxidizing bacteria . Appl. Environ. Microbiol. , 62 : 4147 – 4154 .
- Tamura , K , Dudley , J , Nei , M and Kumar , S . 2007 . MEGA4: Molecular Evolutionary Genetics Analysis (MEGA) software version 4.0 . Mol. Biol. Evol. , 24 : 1596 – 1599 .
- Treusch , AH , Leininger , S , Kletzin , A , Schuster , SC , Klenk , HP and Schleper , C . 2005 . Novel genes for nitrite reductase and Amo-related proteins indicate a role of uncultivated mesophilic crenarchaeota in nitrogen cycling . Environ. Microbiol. , 7 : 1985 – 1995 .
- Tripathi , BP , Ladha , JK , Timsina , J and Pascua , SR . 1997 . Nitrogen dynamics and balance in intensified rainfed lowland rice-based cropping systems . Soil Sci. Soc. Am. J. , 61 : 812 – 821 .
- Venter , JG , Remington , K Heidelberg , JF . 2004 . Environmental genome shotgun sequencing of the Sargasso Sea . Science , 304 : 66 – 74 .
- Wang , Y , Ke , X , Wu , L and Lu , Y . 2009 . Community composition of ammonia-oxidizing bacteria and archaea in rice field soil as affected by nitrogen fertilization . Syst. Appl. Microbiol. , 32 : 27 – 36 .
- Wang , MY , Siddiqi , MY , Ruth , TJ and Glass , ADM . 1993 . Ammonium uptake by rice roots. I. Fluxes and subcellular distribution of 13NH4 + . Plant Physiol. , 103 : 1249 – 1258 .
- Wuchter , C , Abbas , B Coolen , MJL . 2006 . Archaeal nitrification in the ocean . Proc. Natl. Acad. Sci. USA , 103 : 12317 – 12322 .