Abstract
In Vietnam, the Co Dinh mine is the largest chromite mine in the country. Mining, ore dressing and disposal of the tailings provide obvious sources of heavy metal contamination in the mine area. The present study examined the influence of chromite mining activities on the adjacent lowland paddy field by investigating heavy metal and As levels in the mine tailings, sediments, paddy soils and water. At paddy fields located near the mine tailings, the total contents of Cr, Co and Ni were 5,750, 375 and 5,590 mg kg−1, and the contents of their water-extractable form were 12.7, 1.16 and 32.3 mg kg−1, respectively. These results revealed severe contamination of lowland paddy soils with Cr, Co and Ni as a result of mining activity, suggesting serious health hazards through agricultural products, including livestock in this area. The principal source of the pollution was sediment inflow owing to the collapse of the dike, which was poorly constructed by heaping up soil. Moreover, water flowing out from the mining area was also polluted with Cr and Ni (15.0–41.0 and 20.0–135 μg L−1, respectively). This might raise another problem of heavy metal pollution of watercourses in the area, indicating the need for further investigation and monitoring of fluctuations of water quality with seasonal changes.
Key words:
Introduction
Mining activities produce large quantities of waste materials, such as waste rock, tailings and slag, leading to metal contamination of the environment (CitationAdriano 2001; CitationChopin and Alloway 2007; CitationJung 2001). Elevated levels of toxic metals are often reported in agricultural soils, food crops and stream systems as a result of the discharge and dispersion of mine wastes into the environment (CitationJung 2001; CitationLee 2006; CitationMcGowen and Basta 2001). A number of studies have investigated the spatial distribution and behavior of heavy metals in and around mining areas to assess the potential health risks and environmental hazards caused by polluted agricultural products. For example, enrichment of Cr and Ni (86–358 and 21.2–126 mg kg−1, respectively) as a result of mine tailings was reported for surface soils taken from the Almadén mining district in Spain (CitationBueno et al. 2009). Elevated levels of Cr and Ni (182–1,029 mg Cr kg−1 and 15–432 mg Ni kg−1) were also found in soils collected from mining areas in southern Togo (CitationGnandi and Tobschall 2002). CitationBi et al. (2006) reported Cr contamination of agricultural soils (71–240 mg kg−1), resulting from zinc smelting activities in Hezhang County, western Guizhou, China. High levels of heavy metals in paddy fields nearby the Daduk Au–Ag–Pb–Zn mining area of Korea were caused by dispersion of the metals from the tailings by sedimentation and watercourses (CitationLee et al. 2001). In addition, a number of studies have investigated the vertical distribution of heavy metals in soil profiles in and round mining areas, and changes in the geochemical processes throughout the profile (CitationAdriano 2001; CitationJohnson et al. 2000; CitationKim and Jung 2004; CitationOtero et al. 2000). According to these studies, it appears that the profile distribution patterns of each heavy metal are site specific, with no consistent distribution pattern correlating with soil depth.
The toxicity and bioavailability of heavy metals in soils are influenced by the metal’s mobility and reactivity with various environmental factors. Therefore, information about metal speciation as well as its total content is necessary for the assessment of heavy metal toxicity and bioavailability. The proportion of a metal that is mobile and bioavailable will provide practical information for evaluating its potential environmental risks. CitationKien et al. (2009) studied and reported the form and horizontal distribution of Cu and As in paddy soils and watercourses resulting from the exploitation of tin and tungsten ores in Daitu district, in the northern part of Vietnam. However, in Vietnam, few studies on the form and distribution of heavy metals and metalloids have been carried out for agricultural soils affected by mining activities.
In Vietnam, a wide variety of minerals have been found to contain various elements (e.g. antimony, chromite, copper, tin, tungsten), and a lot of metalliferrous mines have been established over the country. Some are currently being mined, whereas others have been abandoned. Many of these mines are located in mountainous areas or in the upper reaches of lowland streams, where various types of crops are cultivated, including lowland rice, which is the major crop. Although dikes are usually constructed around mine areas to prevent the release of tailings, waste water and solid waste into the surrounding environment, frequent occurrences of flooding during the rainy season have caused some of these dikes to collapse and not function properly, resulting in heavy metal pollution in lower streams and farmland areas. Therefore, it is imperative that we accumulate more data related to heavy metal contamination around mining sites where various types of metals are extracted.
In the present study, we assessed the influence of heavy metal contamination on lowland paddy fields in the downstream areas of the Co Dinh chromite mine and clarified possible pathways that the contaminants might take. The chemical forms of the contaminants were also determined to evaluate their mobility and any potential risks to the surrounding environment.
Materials and methods
Co Dinh chromite mine
Mining activity at the Co Dinh chromite mine (19o43′N, 105o36′E; ) was initiated in the early 20th century (∼1930) with ore extraction from the ground surface, followed by open-pit mining. Large-scale and intensive mining activities commenced in the 1990s. Recently, the Co Dinh Chromite Mining Company temporarily closed the mine with the aim of re-construction and installation of new technology. However, local people living in the vicinity of the mine continue small-scale activities using small adits and open pits.
The chromite concentrate produced from this mine contains 46% Cr2O3 and <27% Fe2O3, 5% SiO2 and 0.4% H2O; the estimated ore reserve of the mine is approximately 20.8 metric tons of Cr2O3 (CitationWu 2002, 2004). Annual production of the concentrate reached 80,000 tons per year.
Surface and open-pit mining, followed by on-site ore processing have left a huge number of waste rock piles, spoil heaps and tailing ponds within approximately 2 km2 of the mining area. These mine waste residues are transported by small streams, eventually reaching and being deposited into a large lake (approximately 0.5 km2); the lake was formed by the intensive mining activities (). A number of local people constructed a dike at the border of the mining site by heaping up soil to prevent pollutant inflow into the adjacent agricultural lands. However, the dike sometimes collapses as a result of flooding during the rainy season, resulting in the inflow of sediments, including mine wastes, into the adjacent drainage canals and paddy field areas.
Study sites and sampling
Field surveys and sampling were conducted in November 2006 and November 2007 at study sites selected within the Co Dinh chromite mine and in the adjacent lowland paddy fields (; ). The climate is tropical monsoon with a mean annual precipitation and temperature of 1,600–2,000 mm and 23–24°C, respectively (CitationThanh Hoa Department of Culture, Sport and Tourism 2009). At the time of the field surveys and sampling (November 2006 and November 2007), rice plants had just been harvested and the paddy fields were submerged for the next rice cultivation.
Three representative sites were examined to characterize the soil properties of the study area and to investigate the vertical distribution of heavy metals and As with soil depth: one site was located in the mining area (Sp1) and two sites were located in the areas used for paddy fields, severely affected (Sp2) and non-affected (Sp3) by mine wastes. At each site, a soil pit was prepared and its profile was described. Soil samples were taken from each horizon of the soil profiles, placed into a plastic bag and stored at 4°C until analysis.
To assess the influence of heavy metals and As from the mining areas and to clarify the pathways of contamination, samples of mine tailings, sediment, paddy soil and water were collected from established sites at various distances from the mining area. Tailings was taken from a depth of 0–25 cm at the mine tailings site (designated as MT). Stream sediments were sampled from the bottom of streams running through the mining area (SD1–4 and SD6). In addition, one sediment sample was taken from the lake (SD5; ). In total, eight paddy fields were selected as study sites: six paddy fields (P1–6) were assumed to be affected by sediment inflow as a result of the dike collapse, and two paddy fields (P7–8) were assumed to be unaffected because the transportation road formed an effective barrier. At each paddy field, surface (0–5 cm) and subsurface (20–25 cm) soils were collected. In addition to sampling the tailings, sediments and soils, water samples were collected from each site, including stream water near the MT, SD1 and SD3–4 sites, lake water at SD5 site, and standing water in the paddy fields (P1–2 and P4–8).
Table 1 List of the sampling sites
Sample analyses
Soil and sediment analyses
General physicochemical properties.
The soil and sediment samples were air-dried at room temperature and crushed to pass through a 2-mm mesh sieve. The particle size distribution was determined using the pipette method (CitationGee and Bauder 1986). The clay mineral composition was identified by X-ray diffraction (XRD) analysis using CuKα radiation (XD-D1w; Shimadzu, Kyoto, Japan). The electrical conductivity (EC) and pH (H2O) values were determined using a platinum electrode and a glass electrode, respectively, at 1:5 (w/v) ratio of soil/sediment : water. The pH and redox potential (Eh) of the soils taken from the three soil profiles and from some selected sediment samples were determined in situ. Exchangeable (Ex-) cations (K, Na, Ca and Mg) were extracted with 1 mol L−1 ammonium acetate at pH 7.0, and the contents were determined using an atomic absorption spectrometer ([AAS] AA-6800; Shimadzu). After removing excess NH4 +, the sample was extracted with 100 g L−1 NaCl solution and the supernatant was used to determine the cation exchange capacity (CEC) using the Kjeldahl distillation and titration method (CitationRhoades 1982). The contents of total carbon (TC) and total nitrogen (TN) were analyzed on an NC analyzer (Sumigraph NC-80; Sumitomo Chemical, Osaka, Japan).
Total heavy metals and As.
After the samples were digested in a mixture of HNO3 and HF (9:1) and heated in a microwave (Multiwave, Perkin-Elmer, Yokohama, Japan), the total concentration of the heavy metals (Cr, Co, Cu, Ni and Pb) was determined using AAS. The accuracy of the method was assessed using certificated reference soils (JSO-1, provided by the National Institute of Advanced Industrial Science and Technology, Tsukuba, Japan) and marine sediment (NIES No.12, provided by the National Institute for Environmental Studies, Tsukuba, Japan). The recoveries of Co, Cr, Cu, Ni and Pb were in the ranges 92.6–117, 96.7–101, 96.6–101, 96.2–104 and 92.9–96.2%, respectively.
For the analysis of total As content in the soils and sediments, samples were digested in a mixture of HClO4–HNO3–HF (2:3:5) with the addition of 20 g L−1 KMnO4 in a teflon vessel at 100°C. The concentration of As in the acid digest was determined using an inductively coupled plasma-atomic emission spectrometer ([ICP-AES] ICPS-1000IV; Shimadzu) equipped with a hydride vapor generator (HVG-1; Shimadzu). The standard reference materials JSO-1 and JSO-2 (provided by the National Institute of Advanced Industrial Science and Technology, Tsukuba, Japan) were used to verify the accuracy of the As determination. The recovery rates of As were within 90–95%.
Water-extractable forms of the heavy metals and As.
Water-extractable forms of the heavy metals and As were examined using fresh (moist) soil and sediment samples. The procedure for this extraction was adapted from a previous report by CitationKim et al. (2003). In brief, a 10-g portion of each sample and 50 mL of deionized water were placed into a 100 mL plastic vessel. All samples were shaken using a platform shaker at room temperature for 1 h. The suspended mixtures were filtered through 0.45 μm cellulose membrane filters. The concentrations of the heavy metals and As in the leachates were determined by AAS and ICP-AES, respectively.
Extraction of hexavalent chromium.
Extraction and determination of total hexavalent chromium (Cr(VI)) in the soils and sediments were conducted based on the EPA Method 3060A/7196A with some modifications. The protocol applied here was described in detail by CitationJames et al. (1995). In brief, 2.5 g of homogenized fresh (moist) soil or sediment sample was placed into a 250 mL beaker. Fifty milliliters of a solution of 0.28 mol L−1 Na2CO3 in 0.5 mol L−1 NaOH (pH 12) was added and mixed (unheated) for approximately 10 min. The beakers were then transferred to a preheated 150°C hot plate and maintained at 90–95°C for 60 min with continuous stirring. Then, the solution was cooled and replenished with distilled water to the initial volume. After centrifugation for phase separation (20 min, 2000 g), the supernatant was filtered through a 0.45-μm cellulose membrane filter. The solution was then adjusted to a pH of 7.5 ± 0.5 with 5.0 mol L−1 HNO3 solution and topped up to 100 mL with distilled water. The sample digests were then analyzed using the colorimetric method. One milliliter of diphenylcarbazide solution was added to 80 mL of digest in 100 mL Erlenmeyer flasks. A 1.8 mol L−1 H2SO4 solution was added to the digests until the solution reached a pH value between 1.6 and 2.2, then it was topped up to 100 mL with distilled water. After 10 min, the absorbance at 540 nm was measured using a spectrophotometer (V-360 BIO; Jasco, Tokyo, Japan) and the Cr(VI) concentration was determined against standard solutions ranging from 0.05 to 2 mg L−1.
Water analysis
Water samples were filtered through a 0.45-μm membrane filter and divided into two portions. One portion was acidified with 0.03 mol L−1 HNO3 for analysis of heavy metal and As concentrations, and the other portion was left unacidified for pH and EC measurements. The water samples were then stored in a refrigerator at 4°C until analysis. The total concentrations of heavy metals (Co, Cr, Cu, Ni and Pb) and As were determined by AAS and ICP-AES, respectively.
Results
General physicochemical properties of the soils
The soil profile at the Sp1 site was highly disturbed as a result of mining activity; the A horizon was mainly composed of colluvial materials from the upper slope, whereas the BC and C horizons contained abundant rock fragments of various sizes. The soil profiles from the Sp2 and Sp3 sites resembled each other. However, the profile at the Sp2 site appeared to be more affected by redox reactions owing to uncontrolled flooding caused by the collapse of the dike. The Ap horizon was thicker at the Sp2 site than at the Sp3 site. It should be noted that the soil matrix color was greenish gray or bluish gray throughout the profile at the Sp2 site, but was brownish gray at the Sp3 site.
The physicochemical properties were determined for soils collected from three soil profiles in the Sp1, Sp2 and Sp3 sites (). The Sp1 soils had a clayey texture throughout the profile, with a higher content of TC in the A horizon. The soil pH values were neutral in reaction, with the highest Ex-Mg content among the exchangeable bases. Compared with the Sp1 soils, the Sp3 soils had lower clay contents and a more acidic nature, with higher Ex-Ca contents, which were the highest among the exchangeable bases. The Sp2 soils showed intermediate properties between the Sp1 and Sp3 soils in terms of pH and exchangeable bases. However, the clay content of the Sp2 soils was the highest throughout the profile among the three sites. The XRD analysis revealed that the clay minerals were composed mainly of montmorillonite, kaolin minerals, mica minerals and quartz for the Sp1 soils and kaolin minerals, mica minerals, quartz and gibbsite for the Sp3 soils. In the case of the Sp2 soils, both montmorillonite and gibbsite were detected in addition to the other minerals, which were detected commonly in the Sp1 and Sp3 soils. Based on the USDA soil classification system, paddy soils at both the Sp2 and Sp3 sites were classified as Typic Endoaquepts, whereas the soil at the Sp1 site was determined to be a Lithic Ustorthents (CitationSoil Survey Staff 2006).
Table 2 Physicochemical properties of the soils taken from three soil profiles
As a whole, the physicochemical properties of the mine tailings (MT) and sediments (SD1–6), the P1–6 paddy soils and the P7–8 paddy soils were found to resemble those of the Sp1, Sp2 and Sp3 soils, respectively (; ).
Vertical distribution of total heavy metals and As in the soils
The vertical distributions of total Cr, Co, Ni, Cu, Pb and As were determined in the soil profiles at the Sp1, Sp2 and Sp3 sites. There were different tendencies in the distribution patterns between Cr, Co and Ni and Cu, Pb and As ().
The levels of Cr, Co and Ni were considerably higher in the Sp1 soils than in the Sp3 soils, the respective ranges were 1,616–4,331 mg kg−1, 162–286 mg kg−1 and 4,226–4,700 mg kg−1. At the Sp1 site, the content of Cr and Co was higher in the A horizon than in the BC and C horizons, whereas the Ni content was constant with depth. In contrast, at the Sp3 site, levels of Cr, Co and Ni did not vary appreciably throughout the profile. The Sp2 soils had intermediate levels of these heavy metals between the Sp1 and Sp3 soils, but they were higher in the Ap horizon than in the lower horizons.
Table 3 Physicochemical properties of the mine tailings, sediments and paddy soils
In contrast to Cr, Co and Ni, the levels of Cu, Pb and As were lower in the Sp1 soils than in the Sp3 soils. In the Sp1 soils, Cu and As did not vary with depth, whereas Pb content was high in the A horizon and decreased slightly with depth. At the Sp3 site, the Cu content was highest in the Ap horizon and almost constant in the deeper horizons, whereas Pb content had a minimum value in the B2gir horizon. The total As content at this site was lower in the Ap horizon than in the B1g to B3gir horizons. In the case of the Sp2 site, although the Pb and As contents in the Ap horizon were equivalent or similar to those at the Sp1 site, these values increased with depth, and reached similar levels to those of the Sp3 site in the deeper horizons. Similar to Pb and As, Cu at the Sp2 site tended to be lowest in the Ap horizon, but with relatively high levels throughout the profile.
Horizontal distribution of total heavy metals and As in the soils
and record the levels of total Cr, Co and Ni and Cu, Pb and As, respectively, in the P1–8 soils, tailings (MT) and sediments (SD1–6) collected from the sites shown in .
High contents of Cr, Co and Ni were found in the MT tailings (10,428, 340 and 4,200 mg kg−1, respectively) and in the SD1–6 sediments (2,674–6,700, 294–1,000 and 3,477–7,800 mg kg−1, respectively). The paddy field sites could be classified into three groups based on the content of these heavy metals, which decreased significantly with increasing distance from P1 (). The first group contained the P1–4 sites and the levels of these heavy metals were high compared with the levels in the sediment from SD6. The second group contained the P7 and P8 sites, where the soils were low in Cr, Co and Ni content, similar to the Sp3 site. The P5 and P6 sites were in the last group, and the levels of heavy metals at these sites were higher than those in the second group, but were considerably lower than those in the first group.
Figure 2 Vertical distribution of heavy metals (Cr, Co, Ni, Cu and Pb) and As in three soil profiles (Sp1–Sp3).
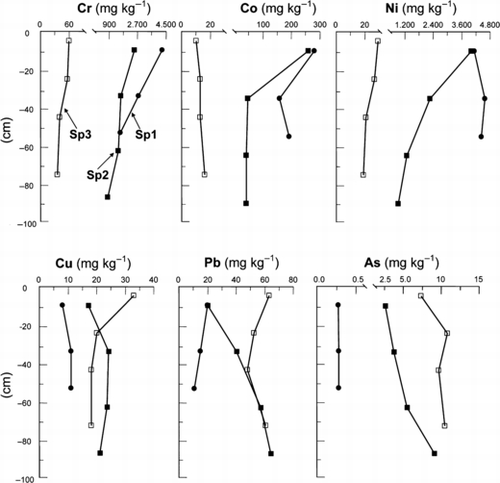
In contrast, the contents of Cu, Pb and As (ranges of 4.12–17.5, 7.14–17.2 and 1.35–4.79 mg kg−1, respectively) in the MT tailings and SD1–6 sediments were almost comparable to the levels found in the Sp1 soils. In the P1–8 sites, the paddy soil ranges were 14.4–64.9 mg kg−1 for Cu, 14.4–93.1 mg kg−1 for Pb and 3.03–11.3 mg kg−1 for As in the surface layers, and 21.2–41.6 mg kg−1 for Cu, 19.2–77.0 mg kg−1 for Pb and 3.41–13.8 mg kg−1 for As in the subsurface layers. The contents of these elements increased significantly with increasing distance from P1 as illustrated by the correlation coefficients in .
Water-extractable heavy metals and As
As a whole, small amounts of heavy metals and As were detected in water-extractable form in the sediment samples (SD2 and SD6) and soil samples (Sp1–Sp3) compared with their total content ().
The levels of Cr, Co and Ni in water-extractable form were relatively high in the sediments and in the Sp1 and Sp2 soils. These metals in the Sp1 and Sp2 soils decreased with depth, except for Co in the Sp1 soils. The Sp3 soils showed a similar distribution pattern for Cr, Co and Ni, but the maximum levels found in the Ap horizon were lower than the minimums found in the B3gir horizon for the Sp2 site. It is noteworthy that the Co content of the Sp1 and Sp2 soils was higher than that of the sediments, whereas the Ni content was highest in the Ap horizon at Sp2 site.
The content of water-extractable Cu and Pb in the Sp1 and Sp2 soils was higher than that in the SD2, SD6 and Sp3 soils. Within the profile, Cu content was high in the BC and C horizons at the Sp1 site, and in the B1gir and B2gir horizons at the Sp2 site, whereas Pb content was high in the A horizon at the Sp1 site and in the B1gir and B2gir horizons at the Sp2 site. At the Sp3 site, Cu and Pb tended to be high in the Ap and B1g horizons. Water-extractable As was not detected in the SD2 and SD6 sediments or in the Sp1 soils, but soils in the upper two horizons at the Sp2 site showed negligible content of As.
Figure 3 (A–C) Horizontal distribution of Cr, Co and Ni in the mine tailings, sediments and paddy soils. □, surface layer; ▪, subsurface layer. (a–c) Relationships between Cr, Co and Ni in the surface layer of the paddy soils and the distance from the P1 site.
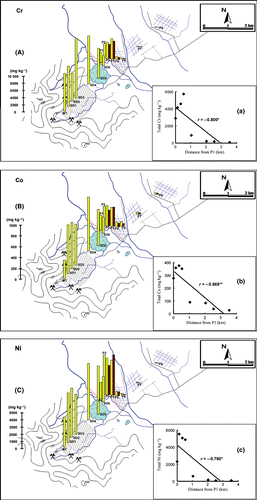
Figure 4 (A–C) Horizontal distribution of Cu, Pb and As in the mine tailings, sediments and paddy soils. □, surface layer; ▪ subsurface layer. (a‐c) Relationships between Cr, Co and Ni in the surface layer of the paddy soils and the distance from the P1 site.
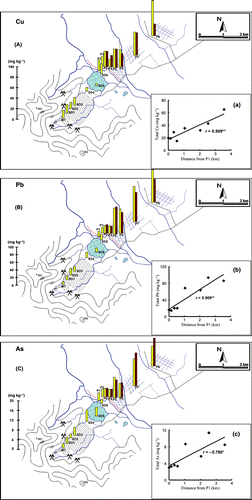
Table 4 Water-extractable contents of heavy metals and As in the sediments and soils taken from three soil profiles
Hexavalent chromium
Taking into consideration that the toxicity of Cr(VI) is much higher than that of Cr(III) (CitationAdriano 2001), the amount of total Cr(VI) was determined for the soil samples from the Sp1, Sp2 and Sp3 sites and for the sediment samples SD2 and SD6 (). also gives the results of field measurements of the pH and Eh values.
Table 5 pH, redox potential and hexavalent chromium content in the sediments and soils taken from three soil profiles
No clear relationships were found between the content of Cr(VI) and pH or Eh values. The Cr(VI) content was higher at the Sp1 and Sp2 sites than at the Sp3 site. The vertical distribution of Cr(VI) in the soil profile resembled that of water-extractable Cr. However, the Sp1 soils had much higher levels of Cr(VI) than water-extractable Cr, although the levels of these Cr fractions were similar to each other in the Sp2 soils.
Concentration of heavy metals and As in the water samples
In the water samples, as distance from the mining area increased, higher concentrations of certain elements were found; for example, moving from the MT to the P2 site for Cr and moving from the SD1 to the P4 site for Ni (). The concentration of Ni, in particular, often exceeded the regulation limit of 100 μg L−1 set by the “Vietnamese standard limitation for surface water” (CitationTCVN 5942-1995 1995). In contrast, Co, Cu, Pb and As were not detected.
Discussion
Influence of mining activity on adjacent paddy fields
Based on the results related to the vertical and horizontal distribution patterns of total heavy metals and As, as well as the general physicochemical soil properties and the soil profile descriptions, mining activity caused Cr, Co and Ni contamination of soils in adjacent paddy fields by sediment inflow at the time of the dike collapse, which happened as a result of flooding. This contamination faded away with distance from the dike, but extended for approximately 2.0 km (to the P5 and P6 sites; ). Levels of these heavy metals were considerably higher throughout the soil profile at the Sp2 site, particularly in the Ap horizon, than the levels at the Sp3 site (), indicating that the collapse of the dike and the resulting inflow of sediments had occurred repeatedly, and that such sediments had been mixed with the original soils through agricultural works such as puddling. Taking into consideration the significant concentrations of Cr and Ni in the water samples, water inflow from the breaking dike and irrigation water, if provided from the mining area, might also contribute to Cr and Ni contamination of the soils ().
Table 6 pH, electrical conductivity and concentration of heavy metals and As in the water samples
In contrast to the Cr, Co and Ni contamination, which resulted from mining activity, levels of Cu, Pb and As were low in the soil, sediment and tailing samples in the mining area. Levels of these elements in the lowland area appeared to depend on the constituents of the parent materials (CitationAdriano 2001; CitationVaselli et al. 1997) because the total contents of these elements were highest at the Sp3 site, and because the B1gir and B2gir horizons at the Sp2 site showed comparable levels of these elements to the levels recorded at the Sp3 site (). At the Sp3 site, the levels of water-extractable Cu and Pb were high in the upper two horizons (Ap and B1g), which could be ascribed to organic matter accumulation derived from plant residue and manure (CitationAdriano 2001). However, at the Sp2 site, water-extractable Cu and Pb from the soils showed a peak in content in the B1gir and B2gir horizons, and these levels were higher than at the Sp3 site, suggesting a different source for these heavy metals. Although the content of water-extractable Cu and Pb in sediments at the SD2 and SD6 sites was low, one possible explanation applicable for Cu, and probably for Pb, is the heterogeneity of these constituents in the mine ores, tailings and sediments within the mining area. This was demonstrated by the different vertical distribution patterns of the water-extractable fractions of heavy metals at the Sp1 site, that is, the decreasing trends with depth for Cr, Ni and Pb and the increasing trends with depth for Co and Cu.
Potential health hazards as a result of contamination
Paddy fields located within 2.0 km of the dike were contaminated with Cr, Co and Ni, although the contamination faded with distance. The total content of Cr, Co and Ni at the P1–P6 sites greatly exceeded the normal levels in uncontaminated soils reported by CitationBowen (1979), that is, 70 mg kg−1 for Cr, 8 mg kg−1 for Co and 50 mg kg−1 for Ni. We have previously surveyed paddy soils near the tin and tungsten mines of Daitu district, Vietnam, and established that the total Cr and Ni ranges were 20.8–27.3 and 5.67–7.48 mg kg−1, respectively (CitationKien et al. 2009). In contrast, CitationPhuong et al. (2009) examined metal pollution of paddy soils in the Red River Delta caused by industrial activities and natural sources and found that the total Cr content was 23–136 mg kg−1 for the surface soils. The total contents of Cr and Ni at the P1–P4 sites of the present study were very much higher than the above-mentioned values. The severe contamination found in the present study can be clearly ascribed to the dike collapse, which allowed direct inflow into the paddy fields of sediments that had a high content of heavy metals.
Water-extractable fractions of Cr, Ni and Co from the soils were higher at the Sp1 and Sp2 sites than at the Sp3 site (). The Ap horizon at the Sp2 site in particular had the highest Ni value among all soil and sediment samples. According to a review on the behavior of Ni and Cr in soils (CitationMcGrath and Smith 1990), Ni is more soluble in water and more easily mobile through soils than Cr. Although accounting for only a small percentage of the total content, the amounts of Cr, Ni and Co in the Ap horizon were 26, 58 and 100-fold higher at the Sp2 site than at the Sp3 site. Because water-extractable metals represent the fraction weakly bound to the soil matrix and, therefore, are readily available to plants (CitationAbollino et al. 2002), it is possible that the rice plants absorb and accumulate this fraction, leading to the occurrence of a serious health hazard.
A small portion of the total Cr was extracted as the highly toxic Cr(VI), probably because a major form of Cr in the mining area is Cr2O3 minerals, and a small amount of Cr(VI) can be released into soils during the weathering process (). However, despite the low Eh value, the level of Cr(VI) in the Ap horizon at the Sp2 site was relatively high, within phytotoxic levels. CitationTurner and Rust (1971) reported that a Cr(VI) level of approximately 0.5 mg L−1 in solution and 5 mg kg−1 in soils could be toxic to plants. CitationAdema and Henzen (1989) evaluated the effect of Cr(VI) on the growth of lettuce (Lactuca sativa), tomato (Lycopersicon esculentum) and oats (Avena sativa) grown in humic sandy and loamy soils and reported that the growth of the lettuce, tomato and oats was inhibited, resulting in 50% yield reductions, at levels of 1.8, 6.8 and 7.4 mg kg−1 Cr(VI) in the soils, respectively. The total root weight and root length of wheat was affected by 20 mg kg−1 Cr(VI) in the soil as K2Cr2O7 (CitationChen et al. 2001).
In addition to sediment inflow, water flowing from the mining area and used for irrigation and drinking can also be a health hazard owing to the high concentrations of Cr and Ni. Furthermore, in this area polluted water would affect domestic animals, such as water buffalo, cattle and ducks, which are bred in and around the paddy fields for farm work or for food.
In contrast, the mining activities in the surveyed area in the present study would not cause severe contamination of Cu, Pb and As because the levels of these elements were below their typical levels in uncontaminated soil (30 mg kg−1 for Cu, 35 mg Pb kg−1 and 6 mg kg−1 for As), as reported by CitationBowen (1979). However, these levels were comparable to the maximum allowable limit for agricultural soils set by the Vietnamese government (CitationTCVN 7209-2002 2002; 50 mg kg−1 for Cu, 70 mg Pb kg−1 and 12 mg kg−1for As). In addition, the data support the suspicion that some portion of the Cu and Pb might originate from the sediments of the mine (). Therefore, the amounts and chemical forms of Cu, Pb and As, as well as Cr, Co and Ni, should be monitored regularly.
Conclusions
The present study revealed that mining activity at the Co Dinh chromite mine caused severe contamination of lowland paddy fields with Cr, Ni and Co, which might cause serious health hazards through agricultural products, including livestock. It is clear that the principal source of the pollution was sediment inflow as a result of the collapse of the dike, which was poorly constructed by heaping up soil. Because the affected area was limited, at most 2 km from the dike, appropriate countermeasures could be applied, probably without difficulty if official support was available. For example, although a solid dike built with concrete was constructed to prevent further inflow of sediments, the polluted area should be enclosed until appropriate decontamination measures have been applied. However, water from the mining area was also polluted with Cr and Ni. This might be another problem because the lake water flows into a river (). Although the present study provided fundamental data related to water quality, further monitoring is required, including monitoring fluctuations in water quality with seasonal changes. Furthermore, monitoring of changes in the chemical forms of the contaminants in the agricultural soils, as well as in their concentrations in the crops (e.g. rice plants), should be carried out regularly.
Acknowledgments
This research was financially supported by a Grant-in-Aid for Scientific Research to K. Iwasaki (grant no. 18380195) from the Japan Society for the Promotion of Science. The authors sincerely thank the officers at the sampling sites and our colleagues at the Hanoi University of Science, Vietnam, for their valuable help and support during sample collection.
References
- Abollino , O , Aceto , M , Malandrino , M , Mentasti , E , Sarzanini , C and Barberis , R . 2002 . Distribution and mobility of metals in contaminated sites. Chemometric investigation of pollutant profiles . Environ. Pollut. , 119 : 177 – 193 .
- Adema , DMM and Henzen , L . 1989 . A comparison of plant toxicity of some industrial chemicals in soil culture and soilless culture . Ecotoxicol. Environ. Saf. , 18 : 219 – 229 .
- Adriano , DC . 2001 . “ Introduction. Chromium. Lead. Copper ” . In Trace Elements in Terrestrial Environment: Biogeochemistry, Bioavailability, and Risks of Metals , Edited by: Adriano , DC . 22 – 23 . 315 – 348 . 350 – 403 . 500 – 539 . New York : Springer-Verlag .
- Bi , X , Feng , X Yang , Y . 2006 . Environmental contamination of heavy metals from zinc smelting areas in Hezhang County, western Guizhou, China . Environ. Int. , 32 : 883 – 890 .
- Bowen , HJM . 1979 . “ Elements in the geosphere and the biosphere ” . In Environmental Chemistry of the Elements , Edited by: Bowen. , HJM . 237 – 273 . London : Academic Press .
- Bueno , PC , Bellido , E , Rubi , JAM and Ballesta , RJ . 2009 . Concentration and spatial variability of mercury and other heavy metals in surface soil samples of periurban waste mine tailing along a transect in the Almaden mining district (Spain) . Environ. Geol. , 56 : 815 – 824 .
- Chen , NC , Kanazawa , S , Horiguchi , T and Chen , NC . 2001 . Effect of chromium on some enzyme activities in the wheat rhizospere . Soil Microorg. , 55 : 3 – 10 .
- Chopin , EIB and Alloway , BJ . 2007 . Distribution and mobility of trace elements in soils and vegetation around the mining and smelting areas of Tharsis, Ríotinto and Huelva, Iberian Pyrite Belt, SW Spain . Water Air Soil Pollut. , 182 : 245 – 261 .
- Gee , GW and Bauder , JW . 1986 . “ Particle-size analysis ” . In Methods of soil analysis: Part 1–Physical and Mineralogical Methods , Edited by: Klute. , A . 383 – 411 . Madison : American Society of Agronomy Soil Science Society of America .
- Gnandi , K and Tobschall , HJ . 2002 . Heavy metals distribution of soils around mining sites of cadmium-rich marine sedimentary phosphorites of Kpogamé and Hahotoé (southern Togo) . Environ. Geol. , 41 : 593 – 600 .
- James , BR , Petura , JC , Vitale , RJ and Mussoline , GR . 1995 . Hexavalent chromium extraction from soils: a comparison of five methods . Environ. Sci. Technol. , 29 : 2377 – 2382 .
- Johnson , RH , Blowes , DW , Robertson , WD and Jambor , JL . 2000 . The hydrogeochemistry of the Nickel Rim mine tailings impoundment, Sudbury, Ontario . J. Contam. Hydrol. , 41 : 49 – 80 .
- Jung , MC . 2001 . Heavy metal contamination of soils and waters in and around the Imcheon Au-Ag mine, Korea . Appl. Geochem. , 16 : 1369 – 1375 .
- Kien , CN , Noi , VN Bang , ND . 2009 . Arsenic and heavy metal concentrations in agricultural soils around tin and tungsten mines in the Dai Tu district, N Vietnam . Water Air Soil Pollut. , 197 : 75 – 89 .
- Kim , MJ , Ahn , KH , Jung , Y , Lee , S and Lim , BR . 2003 . Arsenic, cadmium, chromium, copper, lead, and zinc contamination in mine tailings and nearby streams of three abandoned mines from Korea . Bull. Environ. Contam. Toxicol. , 70 : 942 – 947 .
- Kim , MJ and Jung , Y . 2004 . Vertical distribution and mobility of arsenic and heavy metals in and around mine tailings of an abandoned mine . J. Environ. Sci. and Heath Part A , 39 ( 1 ) : 203 – 222 .
- Lee , S . 2006 . Geochemistry and partitioning of trace metals in paddy soils affected by metal mine tailings in Korea . Geoderma , 135 : 26 – 37 .
- Lee , CG , Chon , HT and Jung , MC . 2001 . Heavy metal contamination in the vicinity of the Daduk Au–Ag–Pb–Zn mine in Korea . Appl. Geochem. , 16 : 1377 – 1386 .
- McGowen , SL and Basta , NT . 2001 . “ Heavy metal solubility and transport in soil contaminated by mining and smelting ” . In Heavy Metals Release in Soils , Edited by: Selim , HM and Sparks. , DL . 89 – 107 . Boca Raton : Lewis Publishers .
- McGrath , SP and Smith , S . 1990 . “ Chromium and nickel ” . In Heavy Metals in Soils , Edited by: Alloway. , BJ . 125 – 150 . Canada : Blackies USA and Halsted Press .
- Otero , XL , Huerta-Diaz , MA and Macías , F . 2000 . Heavy metal geochemistry of saltmarsh soils from Ría of Ortigueira (mafic and ultrmafic areas, NW Iberian Peninsula) . Environ. Pollut. , 110 : 285 – 296 .
- Phuong , NM , Kang , Y Sakurai , K . 2009 . Levels and chemical forms of heavy metals in soils from Red River Delta, Vietnam . Water Air Soil Pollut. , DOI10.1007/s11270-009-0139-0
- Rhoades , JD . 1982 . “ Cation exchange capacity ” . In Methods of Soil Analysis: Part 2–Chemical and Microbiological Properties , Edited by: Pace , AL , Miller , RH and Keeney. , DR . 149 – 165 . Madison : American Society of Agronomy Soil Science Society of America .
- Soil Survey Staff . 2006 . Keys to soil taxonomyThe 18th World Congress of Soil Science Philadelphia, Pennsylvania
- TCVN 5942–19951995Vietnamese standard: water quality–Surface water quality standardVietnamese Ministry of Science and Technology. Available from URL [cited August 2008] (in Vietnamese) http://khcn.mt.gov.vn/tieuchuannganh/uploads/TCVN_5942-1995.pdf (http://khcn.mt.gov.vn/tieuchuannganh/uploads/TCVN_5942-1995.pdf)
- TCVN 7209–20022002Vietnamese standard: Soil quality–maximum allowable limits of heavy metals in the soilVietnamese Ministry of Science and Technology. Available from URL [cited August 2008] (in Vietnamese) http://nea.gov.vn/TCVNMT/ToanVan/7209_02.pdf (http://nea.gov.vn/TCVNMT/ToanVan/7209_02.pdf)
- Thanh Hoa Department of Culture, Sport and Tourism2009IntroductionAvailable from URL [cited September 2009] http://www.thanhhoatourism.com.vn/index.php?lan=e&id=gioithieu&catalog=37 (http://www.thanhhoatourism.com.vn/index.php?lan=e&id=gioithieu&catalog=37)
- Turner , MA and Rust , RH . 1971 . Effects of chromium on growth and mineral nutrition of soybeans . Soil Sci. Soc. Am. J. , 35 : 755 – 758 .
- Vaselli , O , Bucccianti , A and De-Siena , C . 1997 . Geochemical characterization of ophiolithic soils in a temperate climate: a multivariate statistical approach . Geoderma , 75 : 117 – 133 .
- Wu , JC . 2002 . “ The mineral industry of Vietnam ” . In U.S. Geological Survey Minerals Year Book Available from URL http://minerals.usgs.gov/minerals/pubs/country/2002/vmmyb02.pdf [cited August 2008]
- Wu , JC . 2004 . “ The mineral industry of Vietnam ” . In U.S. Geological Survey Minerals Year Book http://minerals.usgs.gov/minerals/pubs/country/2004/vmmyb04.pdf [cited August 2008]