Abstract
Measurements of soil nitrogen (N) transformation rate are needed to be able to assess the plant availability and forest ecosystem losses of N, but little is known about lowland subtropical rainforests. The objectives of the present study were to determine the rates of N mineralization and nitrification in different seasons (January and August 2006) and in different landscape positions (footslope and summit) in the Nanjenshan forest of southern Taiwan, where vegetation types and soil properties vary among different landscape positions. Net N mineralization and nitrification were measured using 28-day in-situ open core and capped core incubations. The results from January 2006 showed that the concentrations of ammonium-N (NH4 +-N), mineral N (NH4 +-N plus NO3 −-N) and nitrate-N (NO3 −-N) were not significantly different between open and capped cores, or between summit and footslope. However, the results of August 2006 showed that the NH4 +-N concentrations of the summit soils were significantly higher than those of the footslope soils (P < 0.05), and that NO3 −-N concentrations in the open cores were significantly lower than those in the capped cores because of higher rainfall in the summer. In general, concentrations of mineral N and NO3 −-N in August were higher than those in January (both P = 0.0003), and NH4 +-N concentrations were significantly different between the different landscape positions (P < 0.05). There were larger soil C and N and microbial N pools at the summit position; however, footslope soils showed higher net N mineralization and nitrification rate as expressed on the basis of per unit C or N. Our results suggested that the substrate properties of the footslope position contributed to the higher net N mineralization and nitrification rate, and that the differences in N transformation rates between the landscape positions appeared to be related to vegetation type.
Introduction
Soil scientists and ecologists have long focused on the study of soil nitrogen (N) mineralization and nitrification rates when they seek to understand controls on terrestrial productivity, nutrient cycling and microbial function (CitationSchimel and Bennett 2004). Rates of soil N mineralization and nitrification often differ between forest types (CitationFinzi et al. 1998; CitationKnoepp and Swank 1998; CitationReich et al. 1997; CitationVenterea et al. 2003), elevations (CitationBohlen et al. 2001; CitationVenterea et al. 2003) and landscape positions (CitationOhrui et al. 1999; CitationVenterea et al. 2003; CitationZak et al. 1991) owing to site variations with different soil organic matter content (CitationBurke et al. 1999; CitationHobbie et al. 2007), temperature and soil moisture (CitationKnoepp and Swank 2002). Although a great deal of attention has focused on how little is known about tropical rainforests, even less is known about subtropical rainforests. This ignorance results primarily from the fact that, although they were once widespread, most subtropical rainforests were converted long ago to agriculture before they could be studied (CitationSun et al. 1998).
The Kenting National Park on the Hengchun Peninsula of Taiwan, located south of the Tropic of Cancer, is geographically divided into a tropical climate region. Forest ecosystems of the Kenting National Park have high species richness and plant diversity because of its special geographic location and, characteristically, the strong effect of the northeastern monsoon winds during the winter (CitationChen et al. 1997; CitationHsieh et al. 2000; CitationSu and Su 1988; CitationSun et al. 1998). The Nanjenshan Nature Reserve of Kenting National Park conserves the last native lowland subtropical rainforest in Taiwan. Moreover, it is noteworthy that the Nanjenshan forests conserve most lowland plant species of Taiwan, and there are also numerous rare and locally endemic species in the Hengchun flora (CitationSun et al. 1998). Despite the low elevation, it is surprising that temperate-zone tree taxa are also present in the subtropics (CitationLiao 1995; CitationSun et al. 1998).
Compared with vegetation research little is known about the soil nutrient cycles and dynamics in the Nanjenshan forests. To maintain and assess the ecological functions and biodiversity of the Nanjenshan forests under the impacts of global climate change, it is important to determine soil N transformation rates as basic information for further research. The objective of the present study was to examine the rates of soil N mineralization and nitrification in different seasons (summer and winter) and in different landscape positions (footslope and summit) at Mt Nanjenshan in southern Taiwan. Although net mineralization assays suffer from technical limitations when used as measures of N cycling dynamics, net N mineralization is discussed as an index of plant-available N (Schimel and Bennett 2004), and it is still a useful measure for comparing the differences among various landscape positions at the study site.
Materials and methods
Site description
The study site (22°37′N, 120°10′E) is located within the Nanjenshan Nature Reserve of Kenting National Park on the Hengchun Peninsula at the southern end of Taiwan (). Annual precipitation is approximately 3,000 mm and mean monthly air temperatures are highest in July (28°C) and lowest in January (18°C) (). Typhoons are very common during the summer and the northeastern monsoon winds usually start in late October and continue until late March of the following year ().
A transect experimental site 450 m long and 10–40 m wide was established on the northwestern ridge of Mt Nanjenshan in 1994. A vegetation census and analysis indicated that there was a phenomenon of vegetation compression with great plant species richness over a short range of 200–400 m in elevation (CitationLiao 1995). The vegetation along this 1-ha transect site consists of 139 free-standing woody species in 91 genera and 49 families, which can be divided into three distinct vegetation types (CitationLiao 1995; CitationWu et al. 2007). Vegetation families found mainly in the tropics dominate the footslope forest. Conversely, families showing definite evidence of northern and eastern Asia affinities are better represented in the summit forest. Compared with the vegetation species distributed on the footslope and summit positions, those on the backslope position show a transition status in forest structure, floristic composition and habitat.
Figure 1 Geographical location of the long-term ecological research site in Nanjenshan Mountain in southern Taiwan.
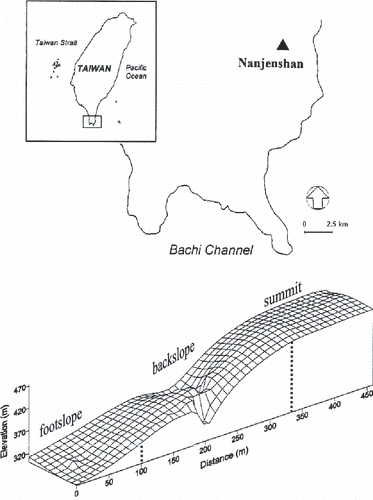
The underlying bedrock within the study area consists of sandstone and shale of Miocene age. Soils located on the summit position are classified as Typic Paleudults based on Soil Taxonomy and have an argillic horizon (Bt) resulting from strong leaching and illuvial processes of ions and clay particles. Soils located on the unstable backslope position associated with steep slopes and soils located on the footslope position are classified as Typic Dystrudepts and have a cambic horizon (Bw) resulting from weak leaching processes (CitationHseu et al. 2001). However, the clay contents of the surface soils (<15 cm) range from 27% at the summit to approximately 30% at the footslope; this difference is not significant (CitationHseu et al. 2001). CitationTsui et al. (2004) indicated that some soil chemical properties were significantly different between the summit and footslope positions of the transect plot, and CitationWu et al. (2007) further indicated that the soil nutrient status appeared to be more related to the mineral nutrient concentrations of the dominant tree species than to other environmental factors.
Field and laboratory methods
To determine net rates in situ of N mineralization and nitrification in different vegetation types and landscape positions at the study site, two sets of three 2 m × 2 m plots were established on the summit and footslope positions, respectively. All six plots were chosen under well-covered canopy with dominant tree species in December 2005. In January and August 2006, in situ soil net N mineralization and nitrification were measured using both open and capped core incubations (CitationRaison et al. 1987).
Within each plot, 20 polyvinyl chloride (PVC) cores (5 cm diameter × 24 cm depth) were driven 20 cm into the soil surface. The thin forest floor layer above the mineral soil was included in the PVC cores (CitationOllinger et al. 2002; CitationStump and Binkley 1993). Four PVC cores were removed immediately, and from each core the 0–15 cm soil layer was collected and mixed in a polyethylene bag. These bulked soil samples were kept in bags and stored in a cooling system until the soil samples were carried to the laboratory for the time zero determination of ammonium-N and nitrate-N concentrations. The focus was on soil from the surface to a depth of 15 cm because these soils usually have the highest N transformation rates. For the other 16 PVC cores, eight cores were capped with aluminum lids to prevent leaching loss by rainfall. There were two bores near the top side in each core for gas exchange. The other eight open cores were covered with 1 mm nylon nets to prevent new litterfall dropping into the core. After incubation in the field for 7, 14, 21 and 28 days, two open cores and two capped cores were removed from each plot at each sampling date. After taking out the soil, the cores were inserted into the original holes and capped to avoid water accumulating in the empty core and disturbing the soils. The soil temperature at a depth of 15 cm and the air temperature were measured, and soil volumetric moisture was also determined by a TDR (time domain reflectometry) measurement (TRIME-FM Version 3, IMKO GmbH, Ettlingen, Germany) during soil sampling. When new field incubations began, we also collected forest floor samples from within a 50 cm × 50 cm area near each plot to determine the concentrations of C, N, P, K, Ca and Mg in litter at footslope and summit landscape positions.
All collected soil samples were kept in a cooling system until we returned to the laboratory as previously described and stored at 4°C until analysis (within 48 h). Field-moist soils were sieved to <5.66 mm and visible litter and stones were removed. Duplicate 20 g fresh soil samples were shaken with 100 mL of 2 mol L−1 KCl for 1 h and then filtered. The concentrations of ammonium-N and mineral N (ammonium plus nitrate) in the filtered extracts were determined using the steam distillation method (CitationKeeney and Nelson 1982). At the beginning (0th day) and end (28th day) of the field experiment, soil microbial biomass carbon and nitrogen were measured using the chloroform fumigation incubation method (CitationJenkinson and Powlson 1976), and the procedures proposed by CitationRice et al. (1996) were followed. Two samples of field-moist soil were weighed (25 g) and adjusted to 55% of water-holding capacity (WHC) and pre-incubated for 7 days. After the pre-incubation period, one set of the duplicate soil samples was placed in a vacuum desiccator and fumigated with ethanol-free chloroform for 24 h; the other set of duplicate soil samples served as the control (unfumigated sample). After the fumigation period, the chloroform was removed and each sample was incubated in a canning jar for 10 days in the dark at room temperature (25°C). A vial containing 2 mL of 2 mol L−1 NaOH was placed in the jar to trap CO2. After the 10-day incubation the excess NaOH was titrated with a standard concentration of HCl to a phenolphthalein endpoint. To determine the amount of microbial biomass N, the soil was extracted with 2 mol L−1 KCl at a solution : soil ratio of 4:1. The differences between CO2-C evolution from the fumigated and unfumigated samples and the microbial N flush were converted to microbial biomass C and microbial biomass N using a k factor of 0.5 (CitationAdams and Laughlin 1981; CitationJenkinson and Powlson 1976).
Spare soil was air-dried, ground and sieved to <2 mm. The gravimetric soil moisture contents of the fresh and air-dried soils were determined by the mass difference before and after drying at 105°C for 48 h. Soil N was assessed using the total Kjeldahl nitrogen procedure (CitationBremner and Mulvaney 1982) and determined by the steam distillation method. Soil organic C content was determined using the modified Walkley–Black method (CitationNelson and Sommers 1982). Litter samples were oven-dried at 65°C for 48 h and milled into powder. The total N and organic C contents of the litter were determined using the same methods as those used for the soil samples. The contents of litter P, K, Ca and Mg were determined by nitric-perchloric acid digestion (CitationMiller 1998).
Net N mineralization and net nitrification were determined as the increase in ammonium plus nitrate (mineralization) or nitrate-N (nitrification) relative to time zero for each field incubation. All data were presented on an oven-dry weight basis, and soil N transformation rates and concentrations were calculated on an area basis using previously determined soil bulk density at the study site.
Statistical analyses
Statistical analyses were conducted using the SAS system (CitationSAS Institute 2003). Soil and microbial biomass concentrations of C and N were averaged within each 2 m × 2 m plot for each incubation time interval. A repeated measures multivariate analysis of variance (manova) was used to test the changes in inorganic N concentrations with different landscape positions, core methods, seasons (months) and incubation time. The landscape positions, core methods and seasons (months) served as between-subject factors, and incubation time served as the within-subject factor. The repeated measures manova was carried out using the general linear model (GLM) procedure. Comparisons of soil and litter CN and microbial properties among the different landscape positions and core incubation methods were made using Student’s t-tests. Correlations were tested with the Pearson correlation coefficient using the CORR procedure in SAS.
Results and discussion
Temperature and moisture regime during the field incubation
There was little rainfall during the field incubation in January and higher rainfall in August, particularly in the last two intervals (). The gravimetric soil moisture contents did not differ between the open and capped cores (data not shown), thus were averaged in each plot. The gravimetric soil moisture contents of the summit soils were significantly higher than those of the footslope soils (P < 0.05) owing to higher soil organic matter (SOM) content on the summit (CitationTsui et al. 2004) that increasing soil water holding capacity, however, volumetric soil moisture contents were not different. The gravimetric soil moisture content of each plot in August was slightly less than in January, probably due to the higher evapotranspiration during summer. Both soil and air temperatures on the summit position were lower than those on the footslope position, although the elevation difference was <200 m ().
Carbon and N concentration of the soil and litter before incubation
The soil organic carbon (OC) and total nitrogen (TN) concentrations of the summit position were significantly higher than those of the footslope position before incubation (), and the soil OC contents were in the range reported by CitationTsui et al. (2004). The soil C:N ratio of the summit position was also greater than the ratio of the footslope position in January, but not in August 2006. On the contrary, litter C and N concentrations did not show any differences between the summit and footslope positions in our collected samples. Only the C:N ratio of the summit litter was significantly greater than the ratios of the footslope litter in January. The N concentration of the litter was lower than the concentrations reported by CitationWu et al. (2007) who measured the fresh leaves of dominant tree species at the study site. These researchers also observed that foliar N concentrations of the tree species that dominated the footslope position tended to be higher. CitationWu et al. (2007) further indicated that the dominant tree species of the footslope position tended to accumulate some nutrient elements, including N.
Table 1 Gravimetric soil moisture (moisture; w/w%), volumetric soil moisture (TDR; v/v%), air temperature and soil temperature at a depth of 15 cm for each sampling date at the Nanjenshan transect site
Table 2 Organic carbon and total nitrogen of the soil and litter properties for the different landscape positions before incubation
Vegetation type and tree species have a great effect on surface soil C and N dynamics (CitationFinzi et al. 1998; CitationHobbie et al. 2007; CitationLovett et al. 2004; CitationOllinger et al. 2002). At the transect site, the species composition of the summit forest is discriminated from the footslope forest, although the elevation difference between the summit and footslope is small (CitationWu et al. 2007; ). Characteristically temperate zone tree taxa (e.g. Fagaceae) occur at the summit position, as well as on the windward slope of the other sites in the Nanjenshan forest (CitationChen et al. 1997; CitationSun et al. 1998). CitationKuo and Lee (2003) indicated that windward species had sclerophyllous leaves with significantly higher leaf mass per area (LMA) than leeward species, and the decomposition rate was found to be negatively correlated with LMA (CitationKurokawa and Nakashizuka 2008). Most of the windward species studied by CitationKuo and Lee (2003) were dominant tree species at the summit position at the transect site, whereas the leeward species dominated the footslope position. In view of the leaf morphology, we suggest that the leaf litter of the summit tree species is comparably less decomposable than that of the footslope tree species. In addition, litter chemical composition is related to decomposition. For example, N, P and S concentrations often limit the decomposition rate in the early phase; in contrast, concentrations of Ca and Mn, which are associated with the growth of white-rod fungal species or the activity of lignin degrading enzyme, have positive relationships to the limit value of decomposition in the very late stage (CitationBerg 2000). The mean concentrations of these elements in our litter were not significantly different in the different landscape (); however, higher concentrations of P, Ca and Mn in soils (CitationTsui et al. 2004) and P and Ca in the dominant tree species (CitationWu et al. 2007) may contribute to greater litter decomposition at the footslope position. Although the C and N concentrations of the litter between summit and footslope were similar, a difference in litter decomposability probably resulted in the higher OC accumulated in the summit soils.
Soil microbial biomass
Comparisons of soil microbial properties at the beginning and at the end of the in situ incubations of soils sampled in January and August 2006 are shown in . After a 28-day incubation in the field, soil microbial C (Cmic), soil microbial N (Nmic) and the Cmic : Nmic ratio between the open and capped core incubations did not differ significantly. The results indicated that the soil microbial properties were not influenced by the different field incubation methods used. In our study, mean microbial biomass C and N concentrations in soils in the footslope position were lower than those in the summit soils. In contrast, the Cmic : Nmic and Cmic : OC ratios in the footslope soils were significantly higher than those in the summit soils (P < 0.05) ().
Table 3 Comparisons of soil microbial properties at the beginning and end of the in-situ core incubation
The ratio of microbial C to soil organic C has been used as an indicator of C availability or decomposability of the substrate (CitationBauhus and Khanna 1999; CitationSparling 1992); it also can reflect the climatic, vegetation, cropping and management history (CitationSparling 1992). Ratios of Cmic : OC at the Nanjenshan transect site () were greater than those recorded from temperate forests (0.5–2.3%, CitationJoergensen et al. 1995; 0.3–9.9%, with a mean value of 1.6%, CitationBauhus and Khanna 1999), New Zealand pasture (0.99–4.30%; CitationSparling 1992), Alaskan boreal forest floors (2.4–3.3%; CitationVance and Chapin 2001) and tropical forest (2.8%; CitationSalamanca et al. 2002). However, there was an obvious pattern in that the Cmic : OC ratios in the summit soils (4.5–5.0%) were comparably lower than those in the footslope soils (7.0–7.7%) at our study site. CitationVance and Chapin (2001) showed that a lower Cmic : OC ratio implies a lower substrate availability in spruce forest soils corresponding with a higher C:N ratio. Although summit soils had higher OC and Cmic levels, the results suggest that the C bioavailability of footslope soils is higher than that of summit soils, and a relatively larger portion of soil OM is resistant to decomposition on the summit position, as previously suggested.
The incorporation of N into the microbial biomass is affected by the availability of C and N and can be expressed by the proportion of microbial N to total soil N (CitationJoergensen et al. 1995). The Nmic : Nt ratios, which were more variable than the Cmic : OC ratios (), were within the ranges reported by CitationBauhus and Khanna (1999) for a large dataset (0.5–10.5%, with a mean value of 3.4%), but our present study did not show any relationship with landscape position or sampling time. The Cmic : Nmic ratios of the footslope (13.7–29.6) were significantly greater than those of the summit (9.0–15.4) because of the lower concentration of Nmic in the footslope soils (). CitationAnderson and Domsch (1980) reported that the Cmic : Nmic ratios of in vitro bacteria and fungi were 5.6 and 7.1–8.3, respectively, and a recent review by CitationCleveland and Liptzin (2007) indicated that the ratios ranged from 2.6 to 20.6. Many other researchers have obtained similar values; however, direct estimates of the C:N ratios of fungi growing in situ in forests range from 14.1 to 29.0, with a mean value of 20.2 (CitationWallander et al. 2003). Our results were higher than those recorded by CitationAnderson and Domsch (1980), CitationCleveland and Liptzin (2007) and most other reports (particularly for the Cmic : Nmic ratio at the footslope), but still reasonable. The higher ratio caused by higher Cmic values possibly resulted from limitations in the chloroform fumigation incubation method, liberated degradable C from the non-living part of the OM or the higher mineralization of fungi (CitationMartens 1995). As fungi have a higher C:N ratio than bacteria (CitationWallander et al. 2003), and large microbial C:N ratios are caused by increased fungal-to-microbial biomass ratios (CitationJoergensen et al. 1995), we suggest that there is a larger proportion of fungi distributed in the footslope soil microbial communities than in the summit communities (i.e. the soil microbial populations differed with landscape position). Further research to clarify soil microbial composition in relation to landscape position and vegetation type within the transect site is necessary.
Net nitrogen mineralization and nitrification of the in situ incubations
During the field incubation in January 2006, the soil mean ammonium-N concentrations of the study site ranged from <1 to 4.51 mg N kg−1, the soil mean nitrate-N concentrations ranged from <1 to 7.86 mg N kg−1, and the mean concentrations of mineral N ranged from 1.73 to 11.5 mg N kg−1 (). Both concentrations of ammonium-N and mineral N were significantly different (P < 0.05) between the open core soils and the capped core soils in the first week of sampling, but did not different on the other sampling dates (). Soil nitrate-N concentrations did not differ significantly between different landscape positions or PVC core methods. Throughout the entire in situ incubation period in January, the soil N concentrations did not differ significantly between the landscape positions or core methods ().
During the field incubation in August 2006, soil mean ammonium-N concentrations varied from approximately 2 to 7.68 mg N kg−1, soil mean nitrate-N concentrations ranged from 3.81 to 17.4 mg N kg−1, and soil mean mineral N ranged from 5.77 to 23.8 mg N kg−1 (). Throughout the entire incubation period in the field, soil ammonium-N concentrations at the summit were significantly higher than those at the footslope (; ; P = 0.003). A sharp decrease in soil N concentrations in the open core soils on day 21 was observed and was caused by rainfall leaching starting 2 days before the sampling date and continuing for a couple of days at the site (), resulting in significantly reduced concentrations of mineral N and nitrate-N in the open cores compared with the capped cores (; ). Thus, the soil nitrate-N concentrations differed significantly between the core methods (; P = 0.04). However, soil mineral N (ammonium-N plus nitrate-N) was not significantly different in the different landscape positions or core methods sampled in August 2006.
We estimated net N mineralization and nitrification rates using a 28-day incubation. However, some of the highest N concentrations were observed before the 28th day during the incubation period (,). For example, the mineral N and nitrate-N concentrations at the footslope (in both open and capped cores) in January were highest on day 21 (), indicating that net mineralization and nitrification rates based on 28-day calculation data could be underestimated. These results also imply that soil N immobilization was greater within the PVC cores over a longer incubation period at the footslope position in January 2006. In addition, differences in the soil N concentration between the open and capped core methods show that there was N leaching in this subtropical rainforest (), as shown in other humid ecosystems. CitationRaison et al. (1987) indicated that N leaching could be assessed by comparing the N quantity presented in these two core methods; however, better field methods have been suggested, for example, the resin core method, to estimate N mineralization and N leaching simultaneously during incubation (CitationBinkley and Hart 1989). In the present study, we focused only on net N mineralization and net nitrification, and other incubation techniques are recommended to precisely assess the N leaching in the future.
As we put the data from two field incubations together and took month as one source of variation, the results of the repeated measures manova indicated that soil ammonium-N concentrations were significantly different between landscape positions (P = 0.01; data not shown), whereas soil mineral N and nitrate-N concentrations were significantly different between months (both P = 0.0003; data not shown). As there were higher concentrations of ammonium-N (particularly in August) (), OC, TN and C:N ratio () in the summit soils, our results are consistent with CitationBooth et al. (2005) who found that soil ammonium-N concentration was positively correlated to soil C and N concentration and the soil C:N ratio.
Figure 3 Concentrations of soil ammonium-N (NH4+-N), mineral N and nitrate-N (NO3−-N) during in situ incubation in January 2006 at the Nanjenshan transect site. Error bars are the standard deviation (n = 3); where no bars are shown only one data point (core) is available.
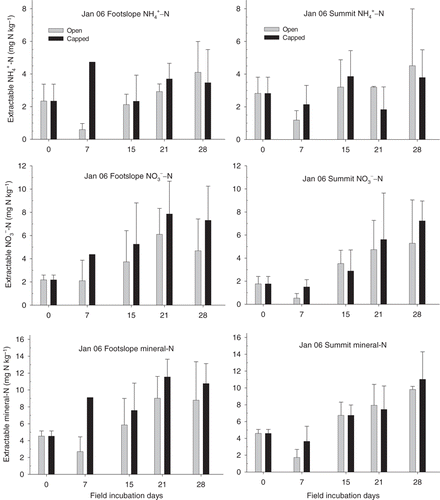
Net rates and amounts of mineralization and nitrification in the two field incubation experiments are shown in . In January 2006, the amount (mg N m−2 28 day−1) of mineralized and nitrified N and the N transformation rate (mg N m−2 day−1) at the footslope were similar to those at the summit. Comparing these two in situ incubations, the net rates or amounts of mineralization and nitrification in August were 1.3-fold and 2.1-fold higher than those in January at the footslope and 2.29-fold and 2.46-fold higher at the summit, respectively, both as a result of significantly higher concentrations in August. Our results indicated that net N mineralization and nitrification differed greatly between January and August, which represented the coldest/driest month and the hottest/wettest month of the Nanjenshan forest (). At the footslope position, the concentrations of soil mineral N and soil nitrate-N were significantly correlated with soil temperature (r = 0.43, P < 0.01 and r = 0.38, P < 0.05, respectively), but not with soil moisture. The correlations between N concentrations and soil temperatures were stronger at the summit position (r = 0.58 for ammonium-N, P < 0.01; r = 0.60 for mineral N, P < 0.001; r = 0.47 for nitrate-N, P < 0.05), and the N concentrations were not significantly correlated with soil moisture content. At our study site, air and soil temperatures in August were higher than those in January, but soil water regimes at one landscape position were not significantly different between January and August (). It appeared that at one landscape position, seasonal variation in N transformation was mainly affected by the temperature regime and less affected by the soil moisture regime at the Nanjenshan transect site. This result confirmed the results recorded from laboratory incubations showing that N mineralization was more responsive to temperature change than to moisture change (CitationKnoepp and Swank 2002; CitationWang et al. 2003).
Table 4 Statistical analysis using the general linear model procedure for soil nitrogen concentrations during in situ incubation periods at the transect site in January and August 2006
Table 5 Repeated-measures multivariate ANOVA of landscape position (landscape), core method (core) and in-situ incubation period (time) on soil nitrogen concentrations at the transect site in January and August 2006
Figure 4 Concentrations of soil ammonium-N (NH4+-N), mineral N and nitrate-N (NO3−-N) during in situ incubation in August 2006 at the Nanjenshan transect site. Error bars are the standard deviation (n = 3).
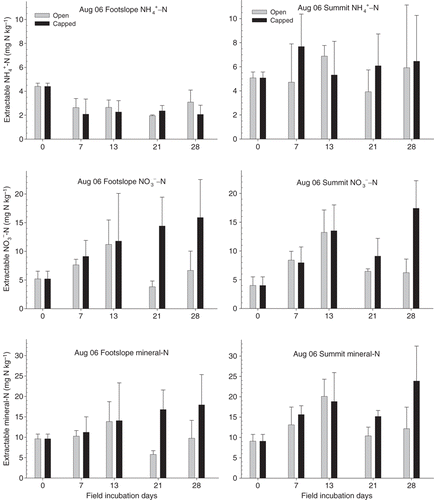
Table 6 Net rates and amounts of mineralization and nitrification at the Nanjenshan transect site
In addition to seasonal variation at the same landscape position, the differences in N transformation rates (mg N m−2 day−1) between the footslope and summit positions were greater in August, and net N mineralization rates and net nitrification rates at the summit were 1.8-fold and 1.3-fold higher than those at the footslope, respectively. In addition to substrate concentration (soil C and N), SOM composition also plays a role in regulating N mineralization, and differences in N mineralization rates among ecosystem types reflect the importance of SOM composition (CitationBooth et al. 2005). Therefore, we also expressed N mineralization and nitrification on the basis of per gram OC and per gram N (mg N g OC−1 day−1 and mg N g N−1 day−1, respectively), and net N mineralization and net nitrification rates at the footslope were both higher than the rates recorded at the summit in January and August (). This is in contrast to our previous discussion on a per square meter basis and reveals that differences in N transformation rates between landscape positions are affected by substrate quality, which is directly linked to vegetation type (CitationKnoepp and Swank 1998) and/or tree species (CitationHobbie et al. 2007). Although there were larger soil C and N pools at the summit (), the higher foliar N concentrations (CitationWu et al. 2007) and substrate quality () of the footslope soils are more favorable to decomposition, suggesting that N in the footslope soil is more labile than N in the summit soil. Our results are consistent with most observations recorded in temperate forests, which indicate that foliar N concentrations are positively correlated with mineralization and/or nitrification and that C:N ratios are negatively correlated with mineralization and/or nitrification (CitationFinzi et al. 1998; CitationLovett et al. 2004; CitationOllinger et al. 2002; CitationStump and Binkley 1993; CitationVenterea et al. 2003).
In addition to substrate quantity and quality, soil microbes play a key role in regulating N transformations. Plant detritus is an important source of labile C that drives soil microbial growth and regulates the balance of soil N mineralization and immobilization (CitationHooker and Stark 2008). Root litter and foliage litter are important input sources to soil organic C. CitationStump and Binkley (1993) found that fine-root litter was generally higher in N than foliage within tree species, and root lignin : N and C:N ratios were correspondingly lower. A recent study by CitationRussell et al. (2007) found that the quantity of fine-root inputs influenced SOC stocks more than the quantity of above-ground inputs in a lowland tropical moist forest in Costa Rica. Moreover, CitationHobbie et al. (2007) indicated that net N mineralization and net nitrification were positively related to root N concentration in both laboratory and in situ assays. Although the fine-root properties have not been determined, we suggest that the quantity and quality of root litter among vegetation types may also affect the microbial biomass and N dynamics at the Nanjenshan transect site.
Conclusions
In general, net N mineralization and nitrification at the Nanjenshan transect site differed with landscape position. Despite the short elevation difference, soil properties and vegetation compositions differed significantly between the summit and footslope positions owing to the special geographic location of the Hengchun Peninsula. The results of our 28-day field incubations indicated that the concentrations of soil ammonium-N varied significantly between the different landscape positions, whereas the concentrations of soil mineral N and nitrate-N determined in August were significantly higher than those determined in January. There were larger soil C and N and microbial N pools at the summit position; however, footslope soils showed higher net N mineralization and nitrification rates (expressed on the basis of per unit C or N). Our results suggest that the substrate properties of the footslope position contribute to the higher net N mineralization and nitrification rate, and that differences in N transformation rates between the landscape positions appear to be related to vegetation type.
Acknowledgments
We are grateful to the staff of the Soil Survey and Remediation Laboratory, Department of Agricultural Chemistry, National Taiwan University, for their assistance during soil sampling at the study site. Financial support for this work was provided by the National Science Council (NSC), Executive Yuan, Government of Taiwan, through grant number NSC 91-2621-B-002-003 and NSC 92-2621-B-002-013.
References
- Adams , TMcM and Laughlin , RJ . 1981 . The effects of agronomy on the carbon and nitrogen contained in the soil biomass . J. Agric. Sci. (Camb.) , 97 : 319 – 327 .
- Anderson , JPE and Domsch , KH . 1980 . Quantities of plant nutrients in the microbial biomass of selected soils . Soil Sci. , 130 : 211 – 216 .
- Bauhus , J and Khanna , PK . 1999 . “ The significance of microbial biomass in forest soils ” . In Going Underground – Ecological Studies in Forest Soils , Edited by: Rastin , N and Bauhus , J . 77 – 110 . Trivandrum, , India : Research Signpost .
- Berg , B . 2000 . Litter decomposition and organic matter turnover in northern forest soils . For. Ecol. Manage. , 133 : 13 – 22 .
- Binkley , D and Hart , S . 1989 . The components of nitrogen availability assessments in forest soils . Adv. Soil Sci. , 10 : 57 – 116 .
- Bohlen , PJ , Groffman , PM , Driscoll , CT , Fahey , TJ and Siccama , TG . 2001 . Plant-soil-microbial interactions in a northern hardwood forest . Ecology , 82 : 965 – 978 .
- Booth , MS , Stark , JM and Rastetter , E . 2005 . Controls on nitrogen cycling in terrestrial ecosystems: a synthetic analysis of literature data . Ecol. Monogr. , 75 : 139 – 157 .
- Bremner , JM and Mulvaney , CS . 1982 . “ Nitrogen-total ” . In Methods of Soil Analysis , Edited by: Page , AL , Miller , H and Keeney , DR . 595 – 624 . Madison : American Society of Agronomy, Incorporation, Soil Science Society of America, Incorportion Publisher . No. 9, Part 2
- Burke , IC , Lauenroth , WK , Riggle , R , Brannen , P , Madigan , B and Beard , S . 1999 . Spatial variability of soil properties in the shortgrass steppe: the relative importance of topography, grazing, microsite, and plant species in controlling spatial patterns . Ecosystems , 2 : 422 – 438 .
- Chen , ZS , Hsieh , CF , Jiang , FY , Hsieh , TH and Sun , IF . 1997 . Relations of soil properties to topography and vegetation in a subtropical rain forest in southern Taiwan . Plant Ecol. , 132 : 229 – 241 .
- Cleveland , CC and Liptzin , D . 2007 . C:N:P stoichiometry in soil: is there a “Redfield ratio” for the microbial biomass? . Biogeochemistry , 85 : 235 – 252 .
- Finzi , AC , van Breemen , N and Canham , CD . 1998 . Canopy tree- soil interactions within temperate forest: species effects on soil carbon and nitrogen . Ecol. Appl. , 8 : 440 – 446 .
- Hobbie , SE , Ogdahl , M Chorover , J . 2007 . Tree species effects on soil organic matter dynamics: the role of soil cation composition . Ecosystems , 10 : 999 – 1018 .
- Hooker , TD and Stark , JM . 2008 . Soil C and N cycling in three semiarid vegetation types: response to an in situpulse of plant detritus . Soil Biol. Biochem. , 40 : 2678 – 2685 .
- Hseu , ZY , Chen , ZS , Tsai , CC and Tsui , CC . 2001 . Using micromorphology to interpret the genesis of tropical forest soils in the Nanjenshan area of Taiwan . Taiwan J. For. Sci. , 16 : 25 – 38 . (in Chinese with English abstract)
- Hsieh , CF , Sun , IF and Yang , CC . 2000 . Species composition and vegetation pattern of a lowland rain forest at the Nanjenshan LTER site, southern Taiwan . Taiwania , 45 : 107 – 119 . (in Chinese with English abstract)
- Jenkinson , DS and Powlson , DS . 1976 . The effects of biocidal treatments on metabolism in soil. V. A method for measuring soil biomass . Soil Biol. Biochem. , 8 : 209 – 213 .
- Joergensen , RG , Anderson , TH and Wolters , V . 1995 . Carbon and nitrogen relationships in the microbial biomass in soils in beech (Fagus sylvaticaL.) forests . Biol. Fertil. Soils , 19 : 141 – 147 .
- Keeney , DR and Nelson , DW . 1982 . “ Nitrogen: inorganic forms ” . In Methods of Soil Analysis , Edited by: Page , AL , Miller , H and Keeney , DR . 643 – 698 . Madison : American Society of Agronomy, Incorporation, Soil Science Society of America, Incorportion Publisher . No. 9, Part 2
- Knoepp , JD and Swank , WT . 1998 . Rates of nitrogen mineralization across an elevation and vegetation gradient in the southern Appalachians . Plant Soil , 204 : 235 – 241 .
- Knoepp , JD and Swank , WT . 2002 . Using soil temperature and moisture to predict forest soil nitrogen mineralization . Biol. Fertil. Soils , 36 : 177 – 182 .
- Kuo , YL and Lee , YP . 2003 . Comparing dehydration tolerance and leaf mass per area between tree species inhabiting windward and leeward sites of Nanjenshan forest in southern Taiwan . Taiwan J. For. Sci. , 18 : 283 – 292 . (in Chinese with English abstract)
- Kurokawa , H and Nakashizuka , T . 2008 . Leaf herbivory and decomposability in a Malaysian tropical rain forest . Ecology , 89 : 2645 – 2656 .
- Liao , CC . 1995 . “ Altitudinal variation in composition, structure, diversity and distribution pattern of the subtropical rain forest in Nanjenshan ” . Taiwan : Graduate Institute of Botany, National Taiwan University . in Chinese with English abstract, MS thesis
- Lovett , GM , Weathers , KC , Arthur , MA and Schultz , JC . 2004 . Nitrogen cycling in a northern hardwood forest: do species matter? . Biogeochemistry , 67 : 289 – 308 .
- Martens , R . 1995 . Current methods for measuring microbial biomass C in soil: potentials and limitations . Biol. Fertil. Soils , 19 : 87 – 99 .
- Miller , RO . 1998 . “ Nitric-perchloric acid wet digestion in an open vessel ” . In Handbook of Reference Methods for Plant Analysis , Edited by: Kalra , YP . 57 – 61 . Boca Raton, , Florida : Soil and Plant Analysis Council, Incorporation, CRC press LLC .
- Nelson , DW and Sommers , LE . 1982 . “ Total carbon, organic carbon, and organic matter ” . In Methods of Soil Analysis , Edited by: Page , AL , Miller , H and Keeney , DR . 539 – 577 . Madison : American Society of Agronomy, Incorporation, Soil Science Society of America, Incorporation Publisher . No. 9, Part 2
- Ohrui , K , Mitchell , MJ and Bischoff , JM . 1999 . Effect of landscape position on N mineralization and nitrification in a forested watershed in the Adirondack Mountains of New York . Can. J. For. Res. , 29 : 497 – 508 .
- Ollinger , SV , Smith , ML , Martin , ME , Hallett , RA , Goodale , CL and Aber , JD . 2002 . Regional variation in foliar chemistry and N cycling among forests of diverse history and composition . Ecology , 83 : 339 – 355 .
- Raison , RJ , Connel , MJ and Khanna , PK . 1987 . Methodology for studying fluxes of soil mineral N in situ . Soil Biol. Biochem. , 19 : 521 – 530 .
- Reich , PB , Grigal , DF , Aber , JD and Gower , ST . 1997 . Nitrogen mineralization and productivity in 50 hardwood and conifer stands on diverse soils . Ecology , 78 : 335 – 347 .
- Rice , CE , Moorman , TB and Beare , M . 1996 . “ Role of microbial biomass carbon and nitrogen in soil quality ” . In Methods for Assessing Soil Quality , SSSA Spec. Publ. No. 49 Edited by: Doran , JW and Jones , AJ . 203 – 215 . Madison : Soil Science Society of America .
- Russell , AE , Raich , JW , Valverde-Barrantes , OJ and Fisher , RF . 2007 . Tree species effects on soil properties in experimental plantations in tropical moist forest . Soil Sci. Soc. Am. J , 71 : 1389 – 1397 .
- Salamanca , EF , Raubuch , M and Joergensen , RG . 2002 . Relationships between soil microbial indices in secondary tropical forest soils . Appl. Soil Ecol. , 21 : 211 – 219 .
- SAS Institute . 2003 . SAS Version 9.1.2 © 2002–2003 , Cary : SAS Institute .
- Schimel , JP and Bennett , J . 2004 . Nitrogen mineralization: challenges of a changing paradigm . Ecology , 85 : 591 – 602 .
- Sparling , GP . 1992 . Ratio of microbial biomass carbon to soil organic carbon as a sensitive indicator of changes in soil organic matter . Aust. J. Soil Res. , 30 : 195 – 207 .
- Stump , LM and Binkley , D . 1993 . Relationships between litter quality and nitrogen availability in Rocky Mountain forests . Can. J. For. Res. , 23 : 492 – 502 .
- Su , HJ and Su , CY . 1988 . Multivariate analysis on the vegetation of Kenting National Park . Quart. J. Chinese For. , 21 ( 4 ) : 17 – 32 . (in Chinese with English abstract)
- Sun , IF , Hsieh , CF and Hubbell , SP . 1998 . “ The structure and species composition of a subtropical monsoon forest in southern Taiwan on a steep wind-stress gradient ” . In Forest Biodiversity Research, Monitoring and Modeling: Conceptual Background and Old World Case Studies , Edited by: Dallmeier , F and Comiskey , JA . 565 – 635 . NY : UNESCO Paris and Parthenon Publishing Co .
- Tsui , CC , Chen , ZS and Hsieh , CF . 2004 . Relationships between soil properties and slope position in a lowland rain forest of southern Taiwan . Geoderma , 123 : 131 – 142 .
- Vance , ED and Chapin , FS . 2001 . Substrate limitations to microbial activity in taiga forest floors . Soil Biol. Biochem. , 33 : 173 – 188 .
- Venterea , RT , Lovett , GM , Groffman , PM and Schearz , PA . 2003 . Landscape patterns of net nitrification in a northern hardwood-conifer forest . Soil Sci. Soc. Am. J. , 67 : 527 – 539 .
- Wallander , H , Nilsson , LO , Hagerberg , D and Rosengren , U . 2003 . Direct estimates of C:N ratios of ectomycorrhizal mycelia collected from Norway spruce forest soils . Soil Biol. Biochem. , 35 : 997 – 999 .
- Wang , WJ , Smith , CJ and Chen , D . 2003 . Towards a standardized procedure for determining the potentially mineralisable nitrogen of soil . Biol. Fertil. Soils , 37 : 362 – 374 .
- Wu , CC , Tsui , CC , Hsieh , CF , Asio , VB and Chen , ZS . 2007 . Mineral nutrient status of tree species in relation to environmental factors in the subtropical rain forest of Taiwan . For. Ecol. Manage. , 239 : 81 – 91 .
- Zak , DR , Hairston , A and Grigal , DF . 1991 . Topographic influences on nitrogen cycling within an upland pin oak ecosystem . For. Sci. , 37 : 45 – 53 .