Abstract
Nitrous oxide (N2O) and methane (CH4) fluxes from a fertilized timothy (Phleum pratense L.) sward on the northern island of Japan were measured over 2 years using a randomized block design in the field. The objectives of the present study were to obtain annual N2O and CH4 emission rates and to elucidate the effect of the applied material (control [no nitrogen], anaerobically digested cattle slurry [ADCS] or chemical fertilizer [CF]) and the application season (autumn or spring) on the annual N2O emission, fertilizer-induced N2O emission factor (EF) and the annual CH4 absorption. Ammonium sulfate was applied to the CF plots at the same application rate of NH4-N to the ADCS plots. A three-way ANOVA was used to examine the significance of the factors (the applied material, the application season and the year). The ANOVA for the annual N2O emission rates showed a significant effect with regard to the applied material (P = 0.042). The annual N2O emission rate from the control plots (0.398 kg N2O-N ha−1 year−1) was significantly lower than that from the ADCS plots (0.708 kg N2O-N ha−1 year−1) and the CF plots (0.636 kg N2O-N ha−1 year−1). There was no significant difference in the annual N2O emission rate between the ADCS and CF plots. The ANOVA for the EFs showed insignificance of all factors (P > 0.05). The total mean ± standard error of the EFs (fertilizer-induced N2O-N emission/total applied N) was 0.0024 ± 0.0007 (kg N2O-N [kg N]−1), which is similar to the reported EF (0.0032 ± 0.0013) for well-drained uplands in Japan. The CH4 absorption rates differed significantly between years (P = 0.014). The CH4 absorption rate in the first year (3.28 kg CH4 ha−1 year−1) was higher than that in the second year (2.31 kg CH4 ha−1 year−1), probably as a result of lower precipitation in the first year. In conclusion, under the same application rate of NH4-N, differences in the applied materials (ADCS or CF) and the application season (autumn or spring) led to no significant differences in N2O emission, fertilizer-induced N2O EF and CH4 absorption.
Introduction
More efficient use and appropriate management of livestock excreta is required in the context of nutrient utilization and demand for reducing environmental pollution (e.g. nitrogen leaching, emission of greenhouse and other pollutant gases). The management system of livestock excreta can be roughly classified into two groups, aerobic treatment (e.g. composting, purification in aeration system) and anaerobic treatment (e.g. digestion treatment).
Anaerobic digestion of livestock slurry by biogas plants provides a valuable energy source of methane (CH4) and reduces the emission of CH4 from stored slurry (CitationClemens et al. 2006). The product produced after the anaerobic digestion of cattle excreta is called “anaerobically digested cattle slurry (ADCS)”, and contains nutrient elements essential for plant and crop growth (CitationMatsunaka et al. 2002). Moreover, the concentration of bad odor and pathogenic bacteria in ADCS is lower than that in undigested slurry (CitationPain et al. 1990; CitationSaxena et al. 1989). In Japan, biogas plants using stock farming as feedstock have not been widely introduced because of the high cost of building such plants (CitationTsukahara et al. 2005). However, understanding the nutrient effect and environmental pollution followed by the application of ADCS to upland and grassland is important for promoting biogas plant systems.
CitationMatsunaka et al. (2003) revealed that the nutrient effect of ADCS as N on the dry matter production of grass was similar to conventional slurry or chemical fertilizer, and that the effect depended on the net applied ammonium nitrogen (NH4-N), which was the difference between the applied NH4-N and the nitrogen loss through ammonia (NH3) volatilization. A lysimeter experiment on timothy sward, conducted in central Hokkaido (northern island) Japan, showed that nitrate (NO3-N) leaching associated with ADCS application in autumn tends to be higher than that in spring, owing to snow cover and snow melting, resulting in higher N-use efficiency of the ADCS in the spring application (CitationMatsunaka et al. 2006). It is also well known that N application and soil environmental factors (e.g. temperature, moisture, pH, oxidation–reduction potential, availability of organic carbon, NH4-N and NO3-N) affect nitrous oxide (N2O) and CH4 fluxes from the soil (CitationLe Mer and Roger 2001; CitationSahrawat and Keeney 1986). From these facts, it is suggested that N2O and CH4 fluxes may be affected by the applied material and the application season, even if the same level of NH4-N is applied to the grassland soil. To date, these effects have not been examined.
In addition, only a few peer-reviewed papers investigating both N2O emission and CH4 absorption from Japanese grasslands over the year have been published (CitationMori et al. 2005, 2008). These studies were conducted in Nasu, Tochigi prefecture, central Japan, and reported annual N2O emission and CH4 absorption. However, these studies did not report the fertilizer-induced N2O emission factor (EF). In Japan, most (92%) grassland for livestock production is located in Hokkaido (CitationStatistics Bureau 2008). Investigations of annual N2O emission rates and CH4 absorption rates and EFs in Hokkaido grassland are important for the estimation of greenhouse gas emissions from Japanese grassland.
In Hokkaido, Japan, timothy swards for making silage and/or hay are commonly fertilized in spring (after snow melting) and in early summer (June, after first cutting), and harvested in early summer (June) and late summer (August–September). However, in farms producing ADCS, it is necessary to apply ADCS to the sward in autumn because the storage tank should be emptied before the snow season. The effects of the applied materials and the application season on N2O emission and CH4 absorption have not been demonstrated. In this paper, we report the results of a 2-year field experiment in central Hokkaido, Japan, using three materials (control, ADCS or chemical fertilizer [CF]) and two application seasons (autumn or spring). The objectives of the present study were to obtain annual N2O and CH4 emission rates and to examine the effect of the applied material and the application season on the annual N2O and CH4 emission and fertilizer-induced N2O EF.
Materials and Methods
Site description
A field study was carried out in timothy (Phleum pratense L.) grassland established in 2002 at the Rakuno Gakuen University, central Hokkaido, Japan (43°04′N, 141°30′E, 61 m a.s.l.). The soil was gray upland soil (Aeric, Typic, Epiaquults). The Ap horizon was at a depth of 0–20 cm. The bulk density and texture were 0.81 Mg m−3 and loam (clay 14%, silt 43% and sand 43%), respectively. The pH (H2O), total carbon content, total nitrogen content and cation exchange capacity were 6.3, 30 g kg−1, 2.5 g kg−1 and 39 cmol(+) kg−1, respectively. The 5-year (2003–2007) averages of precipitation and air temperature were 928 mm year−1 and 7.0°C, respectively (from the nearest weather station, 7 km north-east of the site [CitationJapan Meteorological Agency 2008]).
Experimental design and field management
Experimental plots using a randomized block design were established in mid-October 2003, with three replicates (blocks) and five treatments (). Each plot was 9.0 m2 in area (3.0 m × 3.0 m). The treatments were as follows: (1) CF (as (NH4)2SO4) application in autumn (27 October 2003, 25 October 2004), (2) ADCS application in autumn (dates as above), (3) no nitrogen application (Control), (4) CF application in spring (22 April 2004, 28 April 2005), (5) ADCS application in spring (dates as above).
Both ADCS and CF were manually applied onto the surface of the grassland. The ADCS was applied at a rate of 6.0 kg m−2 (60 Mg ha−1), which is the recommended rate in Hokkaido (CitationMatsunaka et al. 1998). The concentration of ammonium nitrogen (NH4-N) and the total N were measured at each application. Ammonium sulfate was applied at the same application rate of NH4-N in ADCS. The applied NH4-N ranged from 71 to 102 kg N ha−1 (). For the ADCS treatments, four types of N application rate were calculated by considering NH3 volatilization immediately after application (mostly within 48 h; CitationMatsunaka et al. 2008) (). Phosphorus (superphosphate) and K (potassium sulfate) were applied to all plots at a rate of 60 kg P2O5 ha−1 year−1 and 180 kg K2O ha−1 year−1, respectively; two-thirds was applied in spring (at the end of April) and the remainder was applied after the first harvest. The timothy was harvested twice per year, in June (24 June 2004, 16 June 2005) and in August (26 August 2004, 23 August 2005).
Although there were five treatments in the field, six treatment datasets were obtained by considering two periods (autumn start and spring start) for the Control (). As shown in , Y2004 and Y2005 are the datasets for the first and second years, respectively. For the autumn application, Y2004 and Y2005 were obtained from 25 October 2003 to 16 October 2004 and from 17 October 2004 to 27 October 2005, respectively. For the spring application, Y2004 and Y2005 were obtained from 21 April 2004 to 24 April 2005 and from 25 April 2005 to 28 April 2006, respectively.
Field measurements and laboratory analyses
Measurement of the N2O and CH4 fluxes and soil sampling for the determination of the ammonium (NH4-N) and nitrate (NO3-N) contents in the soil were conducted from 25 October 2003 to 28 April 2006, including over the snow season. The sampling frequency was higher (at least twice per week) just after the application of CF and ADCS than in the growing season (usually once per week, at least once every 2 weeks). The total number of field samplings was 75. On most sampling dates, the flux measurement was conducted in the morning and soil sampling was conducted in the afternoon.
Table 1 Application of anaerobically digested cattle slurry (ADCS) and chemical fertilizer (CF) (NH4)2SO4
The closed chamber technique was used to determine the N2O and CH4 fluxes from the soil surface to the atmosphere. In each plot, a round shape metal collar (0.285 m in inside diameter or 0.0638 m2 in area) equipped with a small ditch on the top side was inserted into the soil surface in mid-October 2003. These collars were not removed until the end of April 2006. At each sampling time, a cylindrical polyvinyl chloride chamber (49.0 cm in height) was inserted into the ditch with some water to make it airtight, and the chamber was in place for 0.50 h. The total volume inside the chamber was 0.0392 m3. The gas samples inside the chamber (∼25 cm3) were collected into evacuated glass bottles (15 cm3) with butyl rubber stoppers using a portable syringe at 0.00 and 0.50 hours after chamber placement. A gas chromatograph equipped with an electron capture detector (GC-14B; Shimadzu, Kyoto, Japan) and a gas chromatograph equipped with a flame ionization detector (GC-14B; Shimadzu) were used to determine the N2O and CH4 concentrations, respectively. The flux was calculated using the following equation:
where F is the gas flux (μg N2O-N m−2 h−1 or μg CH4 m−2 h−1), ρ is the gas density under STP (273.15 K and 101325 Pa) (1.26 × 103 g m−3 for N2O-N, 0.717 × 103 g m−3 for CH4), V and A are the volume (0.0392 m3) and area (0.0638 m2) of the chamber, respectively, C 0.50 and C 0.00 are the N2O or CH4 concentrations (μm3 m−3) at 0.50 and 0.00 h after chamber placement, respectively, t is the time of chamber placement (0.50 h), and T is the average temperature (K) measured inside the chamber. It is important to note that CF and ADCS were manually applied to both the inside and outside of the chamber collars at the rates presented above. In the snow seasons, a similar collar was temporarily set on the snow surface in each plot and the same procedure was conducted.
The topsoil at a depth of 0–15 cm was collected on each sampling date to determine the soil NH4-N and NO3-N contents. Field-moist soil samples were extracted in 100 g L−1 KCl solution (soil : solution = 1:5) and the NH4-N and NO3-N concentrations in the extracted solution were determined using a flow injection analyzer (FIA star 5012 analyzer; FOSS, Hillerød, Denmark). Soil NH4-N and NO3-N contents (mg N kg−1) were calculated using these concentrations and the soil moisture content measured by drying soil at 105°C for >48 h. The water-filled pore space (WFPS) was calculated using soil moisture content, bulk density (0.81 Mg m−3) and soil particle density (assumed to be 2.65 Mg m−3).
At each harvest, timothy grass (1.0 m2 area) in each plot was cut at a height of 5 cm from the soil surface, and collected and weighed. One hundred grams of the grass was taken and dried at 70°C for >48 h. After weighing, a portion of the grass was milled and the N content was determined by wet ashing and the steam distillation technique. Finally, the N uptake (kg N ha−1) by timothy grass was calculated.
Daily precipitation (mm) and the daily mean air temperature (°C) of the experimental sward were obtained from the weather station at Rakuno Gakuen University.
Fertilizer-induced N 2 O emission factor and statistical analyses
The fertilizer-induced N2O-N EF was calculated in each block and year. For the ADCS treatments, four types of N application rate were calculated by considering NH3 volatilization (). According to these four values, four types of EFs can be calculated for the ADCS plots as follows:
where EF is the fertilizer-induced N2O EF (kg N2O-N [kg N]−1) and N2O-N is the annual fertilizer-induced N2O emission rate (kg N2O-N ha−1 year−1), which was obtained by subtracting the emission from the control plot in each block and year; is the amount of applied NH−4N minus NH3 volatilization (kg N ha−1 year−1); NTN is the amount of applied total N (kg N ha−1 year−1). In this paper, these four types of EF were calculated. For the CF plots,
was calculated, but all four EF types are assumed to be equal,
, because there is no NH3 volatilization from the CF plots (CitationMatsunaka et al. 2008).
A three-way ANOVA was used to examine the significance of the factors; the material (control, ADCS, CF), the season (autumn, spring) and the year (Y2004, Y2005). The PC software SigmaStat (CitationSPSS 1997) was used for the ANOVA.
Results
Seasonal changes in N 2 O and CH 4 fluxes
The daily precipitation and mean air temperature from October 2003 to April 2006 are shown in ,. Maximum daily precipitation (94.5 mm) was recorded on 7 September 2005. The highest (25.8°C) and lowest (−10.9°C) daily mean air temperatures were recorded on 9 August 2004 and 23 January 2006, respectively. The amount of precipitation in the second year (2005, 807 mm) was greater than that in the first year (2004, 564 mm), and the air temperature showed a similar pattern (). The mean air temperature of the ADCS application dates ranged from 5 to 11°C and precipitation within 12 h after the application of ADCS was not more than 1 mm. These results show that using only one value (0.32) for NH3 volatilization factor () is possible.
Ammonium and nitrate contents in the topsoil fluctuated (). Both contents sharply increased and gradually decreased within 1 month after the application of ADCS or CF, although the magnitudes of the variation differed. These increases and decreases result from the addition of NH4 with the applied materials, nitrification of the added NH4 and uptake by timothy. Little or no change was observed after harvesting of the grass.
Daily mean N2O fluxes ranged from −7 to +113 μg N2O-N m−2 h−1 (). Compared with control plots, higher N2O fluxes were observed in the ADCS and CF plots, particularly after the autumn application. These higher N2O fluxes after the application of ADCS or CF coincided with fluctuations in the NH4 and NO3 contents in the topsoil. Little or no change was observed after harvesting or heavy rain.
Daily mean CH4 fluxes ranged from −164 to +43 μg CH4 m−2 h−1 (). Many CH4 flux values showed negative values, meaning that the methane was absorbed from the atmosphere into the topsoil. There was no noticeable change in CH4 flux after the application of ADCS or CF, harvesting and heavy rain. It appears that CH4 fluxes in the first year were lower (higher absorption) than those in the second year.
Figure 2 (A) Seasonal changes in daily precipitation (bars) and mean air temperature (line), (B) NH4 and (C) NO3 contents in the topsoil (0–15 cm). Small letters and dotted lines denote management events; that is, a, autumn application of anaerobically digested cattle slurry (ADCS) or chemical fertilizer (CF); b, spring application of ADCS or CF; c, first harvest in June; d, second harvest in August. Ctrl, plots with no application; CF Autumn, plots with CF application in autumn; ADCS Autumn, plots with ADCS application in autumn; CF Spring, plots with CF application in spring; ADCS Spring, plots with ADCS application in spring. Circles and error bars show the mean and standard deviation (n = 3), respectively.
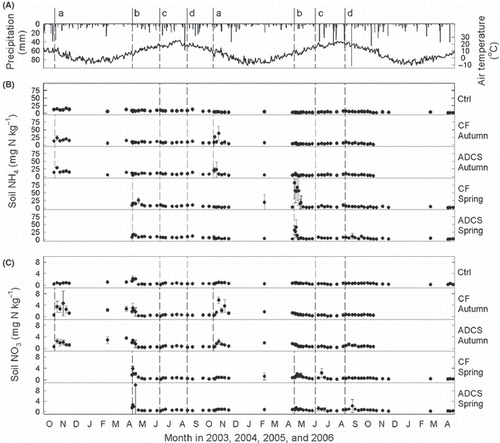
Nitrogen uptake by timothy grass
The annual dry matter production and N uptake by timothy ranged from 3.75 to 13.2 Mg ha−1 year−1 and from 59 to 204 kg N ha−1 year−1, respectively (). Dry matter production and N uptake until first harvest accounted for more than half of the annual uptake (data not shown). The ANOVA showed a significant interaction (season × material, P < 0.01) for both dry matter production and N uptake. This interaction appears to be caused by low growth in CF with autumn application (), which probably resulted from N loss through nitrate leaching followed by rainfall and snow melting before the growing season (CitationMatsunaka et al. 2006). However, the reason for the relatively high dry matter production and N uptake in ADCS with autumn application is unclear. In addition to the significant interaction, the main effects were also significant (material, season, year, P < 0.01) for both dry matter production and N uptake, that is, the application of ADCS and CF increased the dry matter and N uptake of the grass. There was no significant difference in mean N uptake between the ADCS and CF plots (). The higher dry matter production and N uptake in the first year (2004) compared with the second year (2005) is most likely to have been caused by the higher air temperature in spring. The mean air temperature in May 2004 (12.0°C) was higher than that in May 2005 (9.2°C) ().
Annual N 2 O emission and CH 4 absorption rate
Annual N2O emission rates ranged from 0.349 to 0.926 kg N2O-N ha−1 year−1 (). The ANOVA showed a significant effect of material (P = 0.042). The application of ADCS and CF increased the annual N2O emission; the mean annual N2O emission rates in the ADCS (0.708 kg N2O-N ha−1 year−1) and CF plots (0.636 kg N2O-N ha−1 year−1) were significantly higher than those in the control plots (0.398 kg N2O-N ha−1 year−1) (). There was no significant difference in mean annual N2O emission between the ADCS and CF plots. Autumn application plots had relatively higher (P = 0.134) N2O emission rates than spring application plots.
Figure 5 (A) Annual dry matter production and (B) N uptake by timothy grass. Bars and error bars show the mean and standard deviation (n = 3), respectively. The same superscript letters show no significant difference using a Fisher least significant difference test (P > 0.05). ACDS, anaerobically digested cattle slurry; CF, chemical fertilizer.
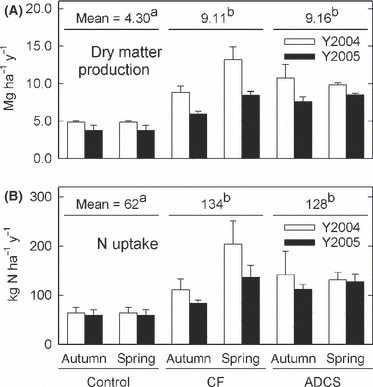
Figure 6 (A) Annual N2O emission rate and (B) CH4 absorption rate. Bars and error bars show the mean and standard deviation (n = 3), respectively. The same superscript letters show no significant difference using a Fisher least significant difference test (P > 0.05). ACDS, anaerobically digested cattle slurry; CF, chemical fertilizer.
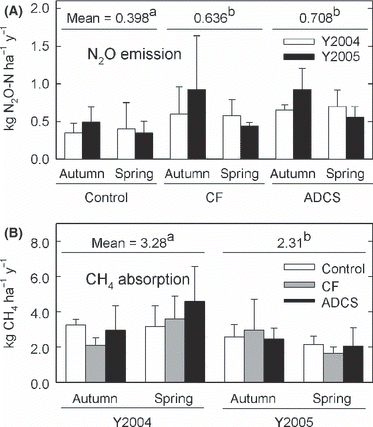
Annual CH4 absorption rates ranged from 1.65 to 4.59 kg CH4 ha−1 year−1 (). The ANOVA showed a significant effect of year (P = 0.014). Mean CH4 absorption rate in the first year (3.28 kg CH4 ha−1 year−1) was significantly higher than that in the second year (2.31 kg CH4 ha−1 year−1). The application of ADCS and CF did not affect the annual CH4 emission rate.
Fertilizer-induced N 2 O emission factor
When calculating the fertilizer-induced N2O EF, the fertilizer-induced N2O emission rate should be obtained by subtracting the emission from the control plot. A significant difference in the annual N2O emission rate between the applied materials was found, indicating that it is possible to calculate the fertilizer-induced N2O EF. The mean EF ranged from 0.0009 to 0.0089 (kg N2O-N [kg N]−1), and large variations were found (). It appears that the EFs in spring application plots were lower than those in autumn application plots. However, there were no significant factors (ANOVA; P > 0.05), although the P-values for season were relatively low (0.067–0.160). shows the total mean (± standard error) of the four types of EFs. The total mean values ranged from 0.0024 to 0.0043.
Figure 7 Fertilizer-induced N2O-N emission factor (EF). Bars and error bars show the mean and standard deviation (n = 3), respectively. ACDS, anaerobically digested cattle slurry; CF, chemical fertilizer; TN, total nitrogen.
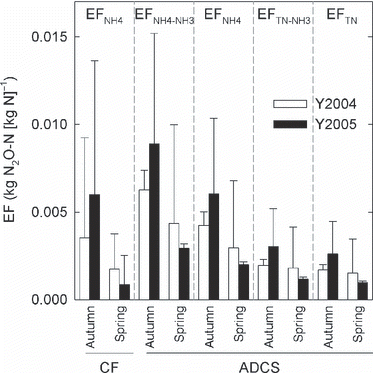
Table 2 Summary of the fertilized-induced N2O emission factors
Discussion
N 2 O and CH 4 flux
In previous reports, the N2O flux increased following rainfall after manure application in a grassland located in Tochigi (CitationMori et al. 2008). In addition, an increase in N2O flux after heavy rain and/or harvest has been observed in crop fields located in Hokkaido (CitationKatayanagi et al. 2008; CitationKusa et al. 2002, 2006; CitationMu et al. 2008). However, little or no change in N2O flux was observed after harvesting or heavy rain in the present study. In another grassland with no fertilizer application located in Tochigi (CitationMori et al. 2005) and in an arable cropping system located in Hokkaido (CitationKoga et al. 2004) the correspondence relationship between heavy rain and an increase in N2O flux was not clear as it was in our study.
Prediction of the N mineralization rate from cattle manure was proposed (e.g. CitationShiga et al. 1985), but the rate from organic matter in ADCS applied to grassland surface is unknown. However, according to the results of CitationSaigusa and Watanobe (2006), it is likely that decomposition of organic matter in the ADCS and subsequent mineral N supply to soil compensate the N loss through NH3 volatilization. However, in the present study, a distinctive increase in mineral N (NH4, NO3) and N2O flux in the ADCS plots was not observed in the growing seasons (July–September) (,).
Higher N2O fluxes were observed mainly after the application of ADCS or CF, which coincided with fluctuations in the NH4 and NO3 contents in the topsoil. Therefore, the increase in the annual N2O emission rates in the ADCS and CF plots compared with the control plots could be attributed to N2O production via nitrification of added NH4-N in the present study.
In general, it is considered that the application of organic matter to the soil increases N2O emission because the easily degradable carbon enhances de-nitrification. For example, a grassland experiment under the same N application rate in Tochigi Japan showed that annual N2O emission rates were significantly higher from manure plots than from chemical fertilizer plots (CitationMori et al. 2008). In contrast, there was no significant difference in mean annual N2O emission between the ADCS and CF plots () in the present study. In other words, it appears that organic matter (ADCS) application did not increase the N2O emission under the same NH4-N application rate. However, we should consider N loss just after ADCS application through NH3 volatilization. Therefore, comparing
in the CF plots with
in the ADCS plots () is suitable for checking the effect of organic matter. No significant effect of the material (P = 0.195) was found because of high variation; however,
in the ADCS plots tended to be higher than
in the CF plots (). These results suggest that the application of organic matter onto grassland surfaces increases N2O emission to some extent. The effect of organic mater application onto grassland surfaces on N2O emission should be analyzed and predicted quantitatively in the future.
Negative CH4 fluxes were observed, which were considered to result from CH4 consumption (oxidation) by methanotrophs in the soil. In general, it is well known that both methanotrophs and methanogens exist in soils and that this bacterial activity and the CH4 flux are related to soil water content and redox condition (CitationLe Mer and Roger 2001). A positive correlation between the CH4 flux and soil water content (CitationMori et al. 2005, 2008) and an increase in CH4 flux after heavy rain (CitationMori et al. 2008) have been observed in a grassland in Nasu, Tochigi prefecture, central Japan. In the present study, a noticeable increase in CH4 flux after heavy rain was not recorded. However, the annual CH4 absorption rate in the first year was significantly higher than that in the second year, which probably resulted from a smaller amount of precipitation and thus a more aerobic soil, and this was favorable for methanotroph activity in the first year. In fact, as shown in , soil WFPS and CH4 flux tended to be higher in the second year (2005) than in the first year (2004) (P = 0.08 for WFPS, P < 0.01 for CH4 flux using a t-test), and a significant positive relationship between WFPS and CH4 flux was found.
Many papers have reported that CH4 oxidation potential and CH4 flux in upland soils are reduced and increased, respectively, by ammonium N fertilizer application (CitationHu et al. 2002; CitationLe Mer and Roger 2001; CitationMosier et al. 1991) and by the NH4-N content in the soil (CitationMori et al. 2005, 2008). However, in the present study, ammonium N fertilizer as ADCS or CF did not reduce the annual CH4 absorption rate compared with the control plots. A possible explanation is that the period with high soil NH4-N content was very short (approximately 1 month after the application).
Figure 8 Relationship between soil water-filled pore space (WFPS) and CH4 flux in the growing season (April–November) in 2004 and 2005. In the box plots, the boundary of the box closest to zero indicates the 25th percentile, the line within the box marks the median and the boundary of the box farthest from zero indicates the 75th percentile. Whiskers above and below the box indicate the 90th and 10th percentiles, respectively.
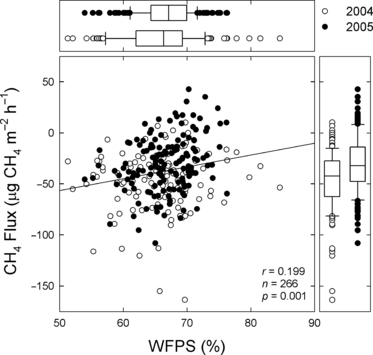
Annual rate of N 2 O emission and CH 4 absorption
Annual N2O emission rates ranged from 0.349 to 0.926 kg N2O-N ha−1 year−1 in the present study. Compared with the N2O emission rates in Japanese grasslands and pastures reported in peer-reviewed papers, the rates in the present study are similar to those in a grassland located in central Hokkaido (0.15–1.54 kg N2O-N ha−1 6-months−1; CitationMu et al. 2008) and in a grassland with no fertilizer application located in Tochigi (0.39–1.59 kg N2O-N ha−1 year−1; CitationMori et al. 2005); and lower than those in a grassland with manure and chemical fertilizer application located in Tochigi (4.7–11.0 kg N2O-N ha−1 year−1; CitationMori et al. 2008) and in grasslands and pastures located in southern Hokkaido (1.1–42.8 kg N2O-N ha−1 year−1; CitationKatayanagi et al. 2008).
The total mean of the annual CH4 absorption rate was 2.80 kg CH4 ha−1 year−1 (ranged from 1.65 to 4.59). Compared with the CH4 absorption rates in Japanese uplands (grassland, arable field, forest) reported in peer-reviewed papers, the rates in the present study were higher than those in an onion field in Hokkaido (−0.78 to 0.36 kg CH4 ha−1 6-month−1; CitationHu et al. 2002), several cropping systems in Hokkaido (0–1.1 kg CH4 ha−1 6-months−1; CitationMu et al. 2006) and a grassland fertilized with manure and ammonium sulfate in Tochigi (0.16–0.84 kg CH4 ha−1 year−1; CitationMori et al. 2008); similar to those in an arable cropping system in Hokkaido (1.4–2.4 kg CH4 ha−1 year−1; CitationKoga et al. 2004), non-fertilized grassland in Tochigi (1.8–2.4 kg CH4 ha−1 year−1; CitationMori et al. 2005) and in 10 forests in Hokkaido (1.4–6.6 kg CH4 ha−1 6-months−1; CitationMorishita et al. 2004); and lower than those in 25 forests in Japan (2.7–24.8 kg CH4 ha−1 year−1; CitationIshizuka et al. 2009).
These annual N2O emission and CH4 absorption rates, including the rates from our study, show high variation, suggesting that N2O and CH4 fluxes from grassland are regulated by many factors, such as plant species, soil type and properties, climate condition, management practices, and any interactions between these factors. Collaborations between field measurements and model analyses (e.g. CitationSaggar et al. 2009) will be required to estimate the amount of N2O emission and CH4 absorption in Japanese grasslands in the future.
Fertilizer-induced N 2 O emission factor
High variation was observed in the calculated fertilizer-induced N2O EFs, which could be a reason for the lack of significance of all factors in the analysis. However, the EFs in the autumn application plots tended to be higher than those in the spring application plots, which is considered to result from relatively higher N2O emission and the lower N application in the autumn application plots compared with the spring application plots (). Relatively higher N2O emission in the autumn application plots may be produced by higher N2O fluxes just after the autumn application. The rate of grass growth (N uptake) following the spring application is usually higher than that following the autumn application (CitationMatsunaka 1987), which would suppress soil NH4-N or NO3-N available for N2O production and flux.
Which type of EF is the most suitable for evaluation of N2O emission from the ADCS plots? In the present study, the NH3 volatilization factor was assumed to be 0.32, but the rate can change with different field conditions, such as temperature and rainfall (CitationMatsunaka et al. 2008). In addition, mineralization of organic matter in ADCS occurs, which can be considered in medium-term to long-term evaluations, although the mineralization rate is unknown at present. Taking these facts into account, EFTN will be the most explicit, universal and convenient measure. The total mean ± standard error of EFTN was 0.0024 ± 0.0007 (kg N2O-N [kg N]−1), which is similar to the reported EF (0.0032 ± 0.0013) for well-drained uplands in Japan (CitationAkiyama et al. 2006). CitationAkiyama et al. (2006) also reported a high EF (0.014) for poor-drained uplands in Japan. CitationToma et al. (2007) investigated variation in the EFs derived from chemical N fertilizer (EFF) and organic matter (EFO) in two soils located in Hokkaido. They suggested that EFF and EFO were related to the mean annual air temperature and mean annual relative humidity, respectively. Along with field measurements and model analyses, considering these factors for EF will contribute to increasing the precision of the estimation of N2O emission from grasslands.
Conclusions
Under the same level of application of NH4-N, differences in the applied materials (ADCS or CF) and application season (autumn or spring) did not lead to any significant difference in N2O emission, fertilizer-induced N2O EF or CH4 absorption.
Acknowledgments
This work was partially supported by the New Energy and Industrial Technology Development Organization as part of a project entitled “Development of Technology to Assess and Verify Life Cycle CO2Emissions; Development of demonstrate the CO2emission output on lifecycle of products and others; Application of LCA methodology to local policies: Case studies on biogas plants in Betsukai-Chou, Hokkaido” (FY2003-2005).
References
- Akiyama , H , Yan , X and Yagi , K . 2006 . Estimations of emission factors for fertilizer-induced direct N2O emissions from agricultural soils in Japan: summary of available data . Soil Sci. Plant Nutr. , 52 : 774 – 787 . doi:10.1111/j.1747-0765.2006.00097.x
- Clemens , J , Trimborn , M , Weiland , P and Amon , B . 2006 . Mitigation of greenhouse gas emissions by anaerobic digestion of cattle slurry . Agric. Ecosyst. Environ. , 112 : 171 – 177 . doi:10.1016/j.agee.2005.08.016
- Hu , R , Hatano , R , Kusa , K and Sawamoto , T . 2002 . Effect of nitrogen fertilization on methane flux in a structured clay soil cultivated with onion in central Hokkaido, Japan . Soil Sci. Plant Nutr. , 48 : 797 – 804 .
- Ishizuka , S , Sakata , T Sawata , S . 2009 . Methane uptake rates in Japanese forest soils depend on the oxidation ability of topsoil, with a new estimate for global methane uptake in temperate forest . Biogeochemistry , 92 : 281 – 295 . doi:type 10.1007/s10533-009-9293-0
- Japan Meteorological Agency . 2008 . “ Climatic Statistics. Available in Web of Japan Meteorological Agency ” . http://www.data.jma.go.jp/obd/stats/etrn/index.php(October, 2008)
- Katayanagi , N , Sawamoto , T , Hayakawa , A and Hatano , R . 2008 . Nitrous oxide and nitric oxide fluxes from cornfield, grassland, pasture and forest in a watershed in Southern Hokkaido, Japan . Soil Sci. Plant Nutr. , 54 : 662 – 680 . doi:type,10.1111/j.1747-0765.2008.00284.x
- Koga , N , Tsuruta , H , Sawamoto , T , Nishimura , S and Yagi , K . 2004 . N2O emission and CH4uptake in arable fields managed under conventional and reduced tillage cropping systems in northern Japan . Global Biogeochem. Cycles , 18 : GB4025 doi:type.10.1029/2004GB002260
- Kusa , K , Hu , R , Sawamoto , T and Hatano , R . 2006 . Three years of nitrous oxide and nitric oxide emissions from silandic andosols cultivated with maize in Hokkaido, Japan . Soil Sci. Plant Nutr. , 52 : 103 – 113 . doi:10.1111/j.1747-0765.2006.00009.x
- Kusa , K , Sawamoto , T and Hatano , R . 2002 . Nitrous oxide emissions for 6 years from a gray lowland soil cultivated with onions in Hokkaido, Japan . Nutr. Cycl. Agroecosyst. , 63 : 239 – 247 . doi:10.1023/A:1021167202601
- Le Mer , J and Roger , P . 2001 . Production, oxidation, emission and consumption of methane by soils: a review . Eur. J. Soil Biol. , 37 : 25 – 50 . doi:10.1016/S1164-5563(01)01067-6
- Matsunaka , T . 1987 . Studies on the effective application of nitrogen fertilizer to timothy sward in Konsen district of Japan . Rep. Hokkaido Prefect. Agric. Exp. Stn. , 62 : 1 – 72 . (in Japanese with English summary)
- Matsunaka , T , Koseki , J and Kondo , H . 1998 . Effective application of cow slurry to cutting sward in Hokkaido . Jap. J. Soil Sci. Plant Nutr. , 59 : 419 – 422 . (in Japanese)
- Matsunaka , T , Kumai , M and Sentoku , A . 2003 . Evaluation of anaerobically digested cattle slurry as a nitrogen source for orchardgrass . Jap. J. Soil Sci. Plant Nutr. , 74 : 31 – 38 . (in Japanese with English summary)
- Matsunaka , T , Naruse , M and Kumai , M . 2002 . Change in nutrients content and some other properties of dairy cattle slurry following anaerobic digestion . Jap. J. Soil Sci. Plant Nutr. , 73 : 297 – 300 . (in Japanese)
- Matsunaka , T , Sawamoto , T , Ishimura , H , Takakura , K and Takekawa , A . 2006 . Efficient use of digested cattle slurry from biogas plant with respect to nitrogen recycling in grassland . Int. Congr. Ser. , 1293 : 242 – 252 . doi:10.1016/j.ics.2006.03.016
- Matsunaka , T , Sentoku , A , Mori , K and Satoh , S . 2008 . Ammonia volatilization factors following the surface application of dairy cattle slurry to grassland in Japan: results from pot and field experiments . Soil Sci. Plant Nutr. , 54 : 627 – 637 . doi:10.1111/j.1747-0765.2008.00277.x
- Mori , A , Hojito , M , Kondo , H , Matsunami , H and Scholefield , D . 2005 . Effects of plant species on CH4and N2O fluxes from a volcanic grassland soil in Nasu, Japan . Soil Sci. Plant Nutr. , 51 : 19 – 27 .
- Mori , A , Hojito , M , Shimizu , M , Matsuura , S , Miyaji , T and Hatano , R . 2008 . N2O and CH4fluxes from a volcanic grassland soil in Nasu, Japan: comparison between manure plus fertilizer plot and fertilizer-only plot . Soil Sci. Plant Nutr. , 54 : 606 – 617 . doi:10.1111/j.1747-0765.2008.00270.x
- Morishita , T , Hatano , R , Nagata , O , Sakai , K , Koide , T and Nakahara , O . 2004 . Effect of nitrogen deposition on CH4uptake in forest soils in Hokkaido, Japan . Soil Sci. Plant Nutr. , 50 : 1187 – 1194 .
- Mosier , A , Schimel , D , Valentine , D , Bronson , K and Parton , W . 1991 . Methane and nitrous oxide fluxes in native, fertilized and cultivated grasslands . Nature , 350 : 330 – 332 . doi:10.1038/350330a0
- Mu , Z , Kimura , SD and Hatano , R . 2006 . Estimation of global warming potential from upland cropping systems in central Hokkaido, Japan . Soil Sci. Plant Nutr. , 52 : 371 – 377 . doi:10.1111/j.1747-0765.2006.00046.x
- Mu , Z , Kimura , SD , Toma , Y and Hatano , R . 2008 . Nitrous oxide fluxes from upland soils in central Hokkaido, Japan . J. Environ. Sci. , 20 : 1312 – 1322 . doi:10.1016/S1001-0742(08)62227-5
- Pain , BF , Misselbrook , TH , Clarkson , CR and Rees , YJ . 1990 . Odour and ammonia emissions following the spreading of anaerobically-digested pig slurry on grassland . Biol. Wastes , 34 : 259 – 267 . doi:10.1016/0269-7483(90)90027-P
- Saggar , S , Hedley , CB , Giltrap , DL and Lambie , SM . 2009 . Measured and modelled estimates of nitrous oxide emission and methane consumption from a sheep-grazed pasture . Agric. Ecosyst. Environ. , 122 : 357 – 365 . doi:10.1016/j.agee.2007.02.006
- Sahrawat , KL and Keeney , DR . 1986 . “ Nitrous oxide emission from soils ” . In Advances in Soil Science , Edited by: Stewart , BA . Vol. 4 , 103 – 148 . New York : Springer-Verlag .
- Saigusa , T and Watanobe , K . 2006 . Fertilizer efficiency and effective split application of anaerobically digested dairy slurry to timothy (Phleum pretenseL.) medow . Bull. Hokkaido Prefect. Agric. Exp. Stn. , 90 : 29 – 39 . (in Japanese with English summary)
- Saxena , KK , Nath , K and Srivastava , SK . 1989 . The effect of using dung from cattle fed high-, low-or no-concentrate rations, on the quality and nutritive value of slurry from a biogas plant . Biol. Wastes , 28 : 73 – 79 . doi:http://dx.doi.org/10.1016/0269-7483(89)90051-7 10.1016/0269-7483(89)90051-7
- Shiga , H , Ohyama , N , Maeda , K and Suzuki , M . 1985 . An evaluation of different organic materials based on their decomposition pattern in paddy soils . Bull. Natl Agric. Res. Cent. , 5 : 1 – 19 . (in Japanese with English summary)
- SPSS . 1997 . SigmaStat 2.0 for Windows User’s Manual , Chicago IL, , USA : SPSS Inc. .
- Statistics Bureau and Statistical Research and Training Institute . 2008 . “ Table 7–8 (Area by type of cultivated land 1980–2005), 7 Agriculture, Forestry and Fisheries ” . In Japan Statistical Yearbook 2008 , 23 Tokyo, , Japan : Statistics Bureau, Ministry of Internal Affairs and Communications . http://www.stat.go.jp/data/nenkan/pdf/yhyou07.pdf(October, 2008)
- Toma , Y , Kimura , SD , Hirose , Y , Kusa , K and Hatano , R . 2007 . Variation in the emission factor of N2O derived from chemical nitrogen fertilizer and organic matter: a case study of onion fields in Mikasa, Hokkaido, Japan . Soil Sci. Plant Nutr. , 53 : 692 – 703 . doi:http://dx.doi.org/10.1111/j.1747-0765.2007.00184.x10.1111/j.1747-0765.2007.00184.x
- Tsukahara , K , Yagishita , T and Sawayama , S . 2005 . Current status of biogas plants in Japan . J. Jap. Inst. Energy , 84 : 537 – 543 . (in Japanese with English summary)