Abstract
In an intensive dairying region, N flow through ammonia (NH3) is comparatively important because of a high-N loading condition based on livestock manure. To elucidate part of the N flow in Orchardgrass (Dactylis glomerata L.) and Italian ryegrass (Lolium multiflorum Lam.) dominant cutting grasslands in an intensive dairy farming region in central Japan, the NH3 flux was measured using a gradient method over 3 years. The 3-year means of the annual balance of NH3 fluxes as the difference between emission and deposition were −14.4 kg N ha−1 in the manure plot and −18.6 kg N ha−1 in the chemical fertilizer plot, averaging −16.5 kg N ha−1. The balance shows the dominance of NH3 dry deposition in both plots, indicating that these grasslands acted as a net sink of NH3, receiving 16.5 kg N ha−1 year−1 of NH3 from the atmosphere. Applications of chemical fertilizer and composted manure caused temporary NH3 emissions equivalent to losses of 11.6 and 4.4%, respectively, of the applied NH4 +-N. A previous study has shown that wet deposition was 18 kg N ha−1 year−1 in this intensive dairy farming region, so atmospheric N input to the grassland was estimated to be 34.5 kg N ha−1 year−1as both dry and wet deposition. This amount equalled 17.3% of the 199 kg N ha−1 year−1 generated locally as manure in the region. Assuming that 25% was selected as a representative NH3 emission factor from livestock manure, atmospheric deposition of ammoniacal N corresponded to 69.5% of the emitted NH3 from livestock manure in the study area, indicating that emission and deposition of NH3 in the area is an important internal flow of the N cycle in this intensive dairying region in central Japan.
Introduction
Agriculture is the major source of anthropogenic ammonia (NH3) emissions in the atmosphere; in particular, livestock waste accounted for 40.5% of the global source of NH3 in 1990 (CitationBouwman et al. 2002). Ammonia, derived mainly from animal manure, is a reactive gas and readily combines with nitrate and sulfate to form fine particulate matter (CitationAsman et al. 1998). These fine particles containing ammonium (NH4 +) can be transported over long distances before being dry-deposited or wet-deposited, but gaseous NH3 is usually deposited much closer to the source (CitationAsman and Van Jaarsveld 1992). When NH3 is deposited, it not only causes acidification and eutrophication of natural ecosystems (CitationSchulze et al. 1989), but also accelerates global warming through the indirect emission of nitrous oxide (Citationvan der Gon and Bleeker 2005).
According to CitationHayashi and Yan (2010), there are two types of deposition, wet and dry, that affect land loading. Wet deposition is comprised of in-cloud and below-cloud scavenging. In-cloud scavenging is the process in which cloud droplets and ice crystals take in substances during their formation in a cloud. Below-cloud scavenging is the process in which rain droplets and snowflakes take in substances on their way to the ground surface. Wet deposition observed at the ground surface is a mixture of these two types. Dry deposition is the process in which gases and particles in the atmosphere are directly deposited onto the ground surface. Unlike wet deposition, dry deposition is ruled exclusively by conditions near the ground surface, such as micrometeorological parameters and the air concentrations of reactive N. The surface conditions strongly affect the rate of dry deposition.
On a global scale, NH3 emission and deposition counterbalance each other (CitationSutton et al. 2008), but, on a local scale, whether a region acts as a net source or a sink depends on many factors, including agricultural activities, soil condition, vegetation and weather.
In Japan, the dense population, relative to that in other developed countries, and limited agricultural land necessitate intensive crop production and the importation of feed for dairy and meat production. Therefore, nitrogen (N) loading on farmland is fundamentally high: 61% comes from livestock manure, which accounts for an estimated 201 kg N ha−1 on average (CitationHojito et al. 2006a). The estimated emission of NH3 from livestock manure averages an estimated 9.6 kg N ha−1, calculated using a unit method on a prefecture level (CitationHojito et al. 2006b). Grassland for forage production can be a source of NH3 owing to N fertilization by livestock manure. In contrast, it also acts as a sink of NH3, particularly in regions with a high atmospheric concentration of NH3, such as intensive dairy farming regions where many farms emit NH3. Thus, in agriculture involving high N-loading conditions, such as that in Japan, the NH3 exchange between the land and the atmosphere is likely to be large, as previously reported in earlier studies of wet deposition and atmospheric concentrations of ammoniacal nitrogen (i.e. NH3 and NH4 +) (CitationHojito et al. 2006a,b). However, no information on dry deposition has been obtained from this intensive dairy farming area.
In this paper, 3 years of observations of NH3 exchange on grasslands in an intensive dairy farming region in central Japan are presented and, with consideration given to the results from other reports, we discuss the ratio of N deposition to the amount of NH3 emission in the area.
Materials and Methods
Site description
A field study was carried out on grasslands at the National Institute of Livestock and Grassland Science (36°55′N, 139°55′E, 320 m a.s.l.). The 30-year averages of precipitation and temperature were 1,561 mm year−1 and 12.0°C, respectively. The dominant plant species were Orchardgrass (Dactylis glomerata L.) and Italian ryegrass (Lolium multiflorum Lam.). The soil is derived from volcanic ash; CitationKurashima et al. (1993) classified it as an Entic Haplumbrept (loamy over fragmental, mixed, mesic; CitationSoil Survey Staff 1999). The site is located in a dairy region typical of Japan, with a number of dairy farms nearby and up to 5 km north-east of the site.
Field management
A manure plot (2.3 ha) and a chemical fertilizer plot (2.4 ha) were set up side by side, with no replication. The terrain of both plots was mostly flat, but had a slight downhill slant from north to south. Both plots were renovated in 2001 and had been harvested three or four times per year, receiving chemical fertilizer at an annual rate of 200 kg N ha−1. The manure plot received composted dairy cattle manure: 15 Mg ha−1 in 2004, 32 Mg ha−1 in 2005, 30 Mg ha−1 in 2006, 30 Mg ha−1 in 2007 (at the time of renovation in September) and 30 Mg ha−1 in 2008. The manure plot also received chemical N fertilizer at 139–157 kg N ha−1 each year, and the chemical fertilizer plot received 186–198 kg N ha−1 each year. The amounts of applied N from 2006 to 2009 are shown in . Urea and ammonium sulfate (7:3 as N weight) were applied together in chemical fertilizer plot, whereas in the manure plot, only ammonium sulfate was used as chemical N fertilizer. The amount of NH4 +-N applied as a chemical fertilizer was equivalent to 139–157 kg N ha−1 in the manure plot and 56–59 kg N ha−1 in the chemical fertilizer plot in 2006, 2007 and 2008, but the amount of applied NH4 +-N as composted manure was very small, although the amount of applied N as composted manure, 124–253 kg N ha−1, was on par with the amount of chemical fertilizer (). In September 2007, both plots were again renovated and re-seeded; chemical fertilizer was applied to both plots at 40 kg N ha−1, and composted manure was applied at the same time to the manure plot instead of the normal application in November. Other nutrients were also applied as follows to the manure and chemical fertilizer plots, respectively: 64 and 99 kg P2O5 ha−1 and 0 and 198 kg K2O ha−1 in 2006; 196 and 220 kg P2O5 ha−1 and 0 and 198 kg K2O ha−1 in 2007; and 57 and 95 kg P2O5 ha−1 and 0 and 189 kg K2O ha−1 in 2008. These values include the chemical fertilizer applied for the renovation in 2007, but exclude the nutrients in the composted manure.
The plots were harvested four times in 2006 and 2008 and three times in 2007. To evaluate the yield, 10–12 replicate grassland plots (10 m in length) were harvested at a height of 5 cm using a reciprocating mower with a 1.3 m width (Farm Mate HFM 1370;STAR Farm Machinery MFG, Chitose, Japan). The fresh weight was determined on site; laboratory grass samples were collected and the dry weight was measured after >48 h of oven-drying at 70°C.
Field measurement of ammonia flux
The atmospheric NH3 concentrations at two heights (0.7 and 2.4 m) were measured using the filter-pack method with filters impregnated with a mixed solution of 5% (v/v) H3PO4 and 2% (v/v) glycerol and then dried under clean conditions (CitationHayashi et al. 2009a). The air flow rate was approximately 5 L min−1 (Iwaki APN-085V-1 air pump; Iwaki, Tokyo, Japan; Yazaki VY3 gas meter; Yazaki, Tokyo, Japan). Over the air intake, we placed a polytetrafluoroethylene membrane filter with a pore size of 0.8 μm (T080A047A; Advantec, Tokyo, Japan) to remove dust and particles, including particulate ammonium.
Table 1 Application rates of nitrogen (kg N ha−1)
The NH3 concentration was measured weekly with the exception of the week immediately following the application of the chemical fertilizer and compost, when it was measured three times per day (09.00, 15.00 and 21.00 hours). The NH3 trapped on the filter paper was extracted with deionized water and measured with a flow injection analyzer (FIAstar 5000; Foss, Tokyo, Japan).
Fluctuations in the 3-D wind velocity and virtual temperature were measured at 10 Hz with a sonic anemometer–thermometer (CSAT3; Campbell Scientific, Logan, UT, USA) installed at a height of 2.5 m (manure plot) or 2.3 m (fertilizer plot). The soil temperature and moisture were measured at a depth of 5 cm in each plot with T-type thermocouples and dielectric aquameter sensors (EC-20; Decagon Devices, Pullman, WA, USA), respectively. The air temperature and relative humidity were measured at a height of 2.5 m with a temperature–humidity sensor (HMP45A; Vaisala, Helsinki, Finland) equipped with a stainless steel ventilated tube. Precipitation was measured with a tipping-bucket rain gauge (Nakaasa Instruments, Tokyo, Japan). The data were sampled with a datalogger (precipitation: 21X or CR1000; others: CR23X; Campbell Scientific). The dataloggers computed and recorded 30-min means (integrated value for precipitation).
A gradient method was used to calculate the exchange flux of NH3 between grasslands and the atmosphere. On the assumption of the similarity between the heat and mass transfers, the exchange flux of NH3, F (μg N m−2 s−1), is expressed as (CitationWyers and Duyzer 1997):
Analyses of the chemical properties of the soil
The chemical properties of the soil were measured in March 2007 and November 2008 at three depths (0–5, 5–10 and 10–15 cm) with 10 replicates in each plot. The soil pH (water) was measured with a pH meter (F-52; Horiba, Kyoto, Japan). The cation exchange capacity (CEC) was measured using SPAD Auto CEC-10 (Fujihira Scientific Company, Tokyo, Japan). The available P was measured by extracting with 0.03 mol L−1 NH4F and a 0.1 mol L−1 HCl solution (Bray No. 2). Exchangeable Ca, Mg and K were extracted with a 1 mol L−1-CH3COOH solution and detected with an atomic absorption spectrophotometer. The total nitrogen (T-N) and total carbon (T-C) were measured with a MACROCORDER JM1000CN (J-Science Lab, Tokyo, Japan). NH4-N and NO3-N were extracted with a 1 mol L−1-KCl solution and detected using a BRAN+LUEBBE AutoAnalyzer 3 (BL Tec, Osaka, Japan).
Composted manure analysis
The T-N of the composted manure applied was measured using a MACROCORDER JM1000CN (J-Science Lab). NH4 +-N and NO3 −-N were extracted with a 1 mol L−1-KCl solution and detected using a BRAN+LUEBBE AutoAnalyzer 3 (BL Tec).
Statistical analyses
Statistical analyses were carried out with EXCEL Statistics software ver. 6.0 (Esumi, Japan; 2007). Differences in the yields and soil chemical properties between the plots were analyzed using a two-sample t-test. Significant correlations were obtained for P < 0.1, P < 0.05 and P < 0.01.
Table 2 Forage yield (Mg ha−1 dry matter)
Results
Grass production and soil chemical properties
The annual dry matter yields of grass were 9.6 (manure plot) and 9.0 Mg ha−1 (chemical fertilizer plot) in 2006, 8.6 and 8.6 Mg ha−1 in 2007, and 10.9 and 12.6 Mg ha−1 in 2008 (). These yields are higher than normal yields in this region (8.0 Mg ha−1). The pH values of the surface layer (0–5 cm) were 6.11 ± 0.24 (mean ± standard deviation) (manure plot) and 6.02 ± 0.32 (chemical fertilizer plot) in 2007 and 6.30 ± 0.15 and 6.05 ± 0.41 in 2008; there were no significant differences between the plots (). The CEC (cmolc kg−1 dry soil) were 21.05 ± 2.29 (manure plot) and 17.87 ± 4.25 (chemical fertilizer plot) in 2008, showing a difference at the 10% level, which was affected by the repeated application of composted manure. The available P, exchangeable Ca and Mg showed higher values in the manure plot at the 5% and 1% level, which were also the result of manure application. The exchangeable K, NO3 −-N, NH4 +-N, T-N and T-C were not significantly different between the plots.
Table 3 Soil chemical properties
Atmospheric concentration of NH 3
From March 2006 to March 2007 (“2006”), the NH3 concentration was 2–10 μg N m−3 (0°C, 1 atm), except on four occasions just after the chemical fertilizer and composted manure were applied. This was particularly so in March, May, July and September 2006, when the NH3 concentrations increased sharply to more than 30 μg N m−3 (). Concentrations at 0.7 m were higher than those at 2.5 m at those times. The concentration exceeded 20 μg N m–3 at 0.7 m following the application of manure in November 2006 (). From March 2007 to March 2008 (“2007”), the NH3 concentration showed a similar pattern to that in 2006, with sharp peaks in June and August at 0.7 m. From March 2008 to March 2009 (“2008”), sharp peaks at 0.7 m occurred in March, May, July and September, when chemical fertilizer was applied, and in November, when composted manure was applied (data not shown). The particulate ammonium concentration averaged 1.5 μg N m−3 for the 3 years and did not differ significantly between the plots, the two heights or the seasons (shown in for the first year).
Figure 1 Seasonal variations in the atmospheric concentration of NH3 from March 2006 to March 2007 in the (a) manure and (b) fertilizer plots. Upper, 2.4 m height; lower, 0.7 m height; ‘Particle’, the averaged value of particle ammonium at 2.4 m height and 0.7 m height. The arrows with no sign show the timing of the chemical fertilizer application and the arrow with ‘M’ refers to the application of manure.
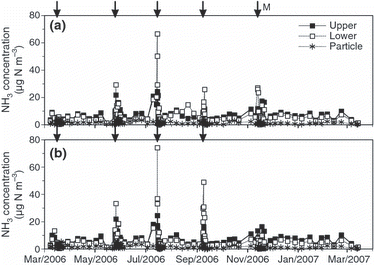
Ammonia emission fluxes
Sharp positive fluxes indicating NH3 emission were observed in both plots in May, July and September, 2006, just after the application of chemical fertilizer, and in the manure plot in November 2006 (). The peaks were particularly high in July and September (>60 g N ha−1 h−1) and even higher in the chemical fertilizer plot. In March 2007, no NH3 emission was observed immediately after the application of chemical fertilizer. At all other times in “2006,” negative fluxes ranging from 0 to −10 g N ha−1 h−1 indicated dry deposition of NH3 ().
In 2007, strong NH3 emissions (>80 gN ha−1 h−1) were observed in both plots in June and August, just after the chemical fertilizer applications, and emissions were higher in the chemical fertilizer plot (). During renovation in September 2007, a weak emission was observed only in the manure plot. Otherwise, negative fluxes of 0 to −10 g N ha−1 h−1 were observed in both plots.
In 2008, NH3 emissions following fertilization were observed (), but the peak in September (<30 g N ha−1 h−1) was smaller than the peaks recorded in previous years. A very high peak exceeding 190 g N ha−1 h−1 was observed in the manure plot immediately after the manure application in November 2008, but fluxes of 0 to −10 g N ha−1 h−1 were observed at other times.
Ammonia volatilization loss
Annual cumulative emissions ranged from 3.6 to 8.5 kg N ha−1 year−1 in the manure plot and from 4.3 to 9.4 kg N ha−1 year−1 in the chemical fertilizer plot (). The NH3 volatilization losses represented 2.5–5.4% (4.4% on average) of the applied NH4 +-N in the manure plot and 7.3–16.8% (11.6% on average) in the chemical fertilizer plot.
Table 4 Annual balance of ammonia fluxes (kg N ha−1 year−1)
Discussion
Ammonia emission from field-applied fertilizers
Marked emissions of NH3 started within 1 day of the application of the chemical fertilizer and continued for approximately 3 days. Maximum emissions occurred every July in the chemical fertilizer plot, coinciding with the timing of the maximum soil temperature at a depth of 5 cm. There was little difference in the plots with regard to the amounts of total N applied except in March; however, the amounts of NH4-N applied differed: 147–165 kg ha−1 in the manure plot and 56–59 kg ha−1 in the chemical fertilizer plot because of the difference in the chemical form from one treatment to the next. Nevertheless, the emission peaks were higher in the chemical fertilizer plot. This difference in emission peaks between the plots can be ascribed to the higher CEC (which increased the adsorption of ammonium to the soil; CitationSommer et al. 2004; CitationHayashi et al. 2009b) in the surface (0–5 cm) soil layer in the manure plot. However, it is not possible to ascribe all of the peaks of positive fluxes after the application of chemical fertilizer to the soil chemical properties, soil temperature and amount of N applied. In May 2006, May 2008 and September 2008, the maximum heights of the peak fluxes were small even at high soil temperatures; these cases can be explained by the rainfall. In May 2006, 79 mm fell over 3 days at the time of chemical fertilizer application; in May 2008, 108 mm fell, and the grassland was flooded; and in September 2008, 96 mm fell over 4 days after the chemical fertilizer was applied and the grassland was flooded. Just after the application of composted manure, a similar positive peak was observed, but there were large differences in the maximum peak values between 2006 and 2008. However, the cumulative positive flux after 3 days of manure application was 0.82 and 2.3 kg N ha−1 for 2006 and 2008, respectively, which accounts for 84% and 27% of the amount of applied NH4 +-N as composted manure. The emission rate was somewhat smaller in 2008. This result means that the emission peak (height) reflects the NH4 +-N concentration of the manure applied (0.0032% in 2006 and 0.028% in 2008), but the emission rate depends on the amount of NH4 +-N applied as composted manure (0.97 kg N ha−1 in 2006 and 8.43 kg N ha−1 in 2008). One reason for the decreasing emission rate in 2008 compared with that in 2006 is an increase in the CEC of the surface soil, which was caused by the continuous application of composted manure.
Soil pH is an important factor controlling NH3 emission from the soil, which increases above pH 7.0 (CitationSommer et al. 2004). The pH of the surface soil in the plots ranged between 6.02 and 6.32, which would be unlikely to increase NH3 emission. This range is typical of grasslands in Japan, where the initial soil pH during renovation is maintained at 6.5. CitationHayashi et al. (2008) reported that the strongest factor affecting NH3 volatilization from a submerged paddy field was the pH of the water, followed by the NH4 +-N concentration and wind speed.
In summary, the NH3 emissions from the study plots occurred immediately after the application of chemical fertilizer or composted manure and continued for approximately 3 days. The magnitudes of emission depended on the amounts of NH4 +-N applied to the plots, soil temperature, CEC and rainfall.
Dry deposition and the annual balance of NH 3 at the study plots
The 3-year means of the annual balance of NH3 exchange (emission–deposition) were −14.4 kg ha−1 in the manure plot and −18.6 kg ha−1 in the chemical fertilizer plots; the average of the two plots was −16.5 kg ha−1. The balances show the dominance of NH3 dry deposition in both plots (). The generally high concentration of atmospheric NH3 at the research site was the fundamental cause of the dominance of dry deposition. The high NH3 concentrations resulted from the emissions of NH3 from the intensive dairy farms near the research site. A similar intensity of wet deposition of 18 kg ha−1 in the same area has also been reported (CitationHojito et al. 2006a). The NH3 dry deposition at the study plots was much higher than that recorded in the non-agricultural regions of Japan, and very few values in China were higher (CitationHayashi and Yan 2010). Furthermore, the NH4 + wet deposition of 18 kg ha−1 in the study area was also higher than that in Japan, but most cases in China were higher than this value (CitationHayashi and Yan 2010). In the present study, the influence of the particulate ammonium on dry deposition was insignificant considering the results obtained, that is, concentrations showed no difference between plots, the two heights or the seasons.
The total emission of NH3 over 3 years from both plots was approximately 20 kg ha−1 year−1, which corresponded to 4.4 and 11.6% of the applied NH4 +-N (2.0 and 3.5% of T-N) in the manure and chemical fertilizer plots, respectively. The NH3 emission from the grassland appears to have been facilitated by the surface application: the emission rate of 11.6% in the chemical fertilizer plot was higher than that in a paddy field fertilized by urea (1.4%; CitationHayashi et al. 2006) or that in an upland field of bare soil fertilized with composted cattle manure with surface incorporation (9.3%; CitationHayashi et al. 2009a); however, it was lower than that recorded from surface-broadcast slurry on grassland (20–40%; CitationSaito et al. 1989 and 22–61%; CitationMatsunaka et al. 2008) and, in many cases, in China (8.8–40% from paddies and 0.6–48% from uplands; CitationHayashi and Yan 2010). It is critical that the cumulative deposition was much higher than the cumulative emission, as explained by the presence of nearby dairy farms as a strong source of atmospheric NH3.
Estimation of the ratio of N deposition to emission in the area and its uncertainty
Using the NH3 dry deposition data obtained from the two grassland areas in the present study in combination with data of NH4 + wet deposition from an early study (CitationHojito et al. 2006a), the total ammoniacal N deposition in the study area can be evaluated. The NH4 + wet deposition in this intensive dairy farming area from six monitoring sites averaged 18 kg N ha−1 year−1; however, the open-bulk samplers to collect rainwater also involved dry deposition components to some extent (CitationHojito et al. 2006a). Assuming that the measured dry deposition in the present study represents the average of the intensive dairy farming area, the total deposition of ammoniacal N was estimated to be 34.5 kg N ha−1 year−1 as the input from the atmosphere to the area. To specify the area for the NH3 generation, a set of communities was selected, corresponding to a unit of statistical data in the agricultural census (CitationMinistry of Agriculture, Forestry and Fisheries 2001) covering 8310 ha (including 2161 ha of agricultural land), which was selected as an overlap in the former report (CitationHojito et al. 2006a). The amount of N produced in livestock excreta calculated from the livestock number was 1650.5 Mg, equivalent to 199 kg N ha−1 year−1 over the entire land area (or 764 kg N ha−1 year−1 over the agricultural area), an extremely high N load. The total deposition of 34.5 kg N ha−1 year−1 corresponded to 17.3% of the amount of generated N as livestock manure. Emission factors of NH3 from livestock manure differ among livestock species and manure treatment methods. In the case of dairy cattle, the sum of NH3 emissions over the time from the livestock barn, manure treatment to the application of manure to arable lands ranges from 17 to 27% of the total N of livestock manure (CitationAmon et al. 2001; CitationBalsari et al. 2007; CitationDemmers et al. 1998; CitationKoerkamp et al. 1998; CitationKulling et al. 2001; CitationLiang et al. 2006; CitationParkinson et al. 2004; CitationRumburg et al. 2008; CitationSmith et al. 2007; CitationSommer et al. 2006; CitationWillers et al. 1996; CitationZhang et al. 2005). Assuming that 25% was selected as a representative NH3 emission factor from livestock manure, 412.6 Mg of NH3-N was emitted from livestock manure in the study area, which was equivalent to 49.7 kg N ha−1 year−1; then, the atmospheric deposition of ammoniacal N, 34.5 kg N ha−1 year−1, accounted for 69.5% of the NH3 generated and emitted to the atmosphere in the area.
This estimation, however, includes many uncertainties because not all of the deposition originates from local sources; some correction is needed to give an accurate estimate of the ratio of N deposition to emission in a target area. How well the estimated dry deposition represents the region is uncertain. As dry deposition is affected by many factors, including the type of vegetation, phenology, topography, soil and weather, a rational method of estimating dry deposition in the target region is needed. A method to accurately estimate wet deposition independently of dry deposition is also needed. Furthermore, by obtaining accurate NH3 emission rates in relation to the actual farming management of the region, we can more accurately and practically estimate the ratio of N deposition to emission through atmospheric NH3.
Conclusion
Grasslands in an intensive dairy region acted as a net sink of NH3, receiving 16.5 kg N ha−1 year−1 of NH3 from the atmosphere. Applications of chemical fertilizer and composted manure caused temporary NH3 emissions, corresponding to NH3 volatilization losses of 11.6 and 4.4%, respectively, of the applied NH4 +-N. Atmospheric deposition of ammoniacal N to the grassland was estimated to be 34.5 kg N ha−1 year−1, which corresponded to 17% of the amount of N generated as manure in this region (199 kg N ha−1). Assuming an average NH3 emission factor of 25% to the generated N as livestock manure, the atmospheric deposition of ammoniacal N corresponded to 69.5% of the emitted NH3 from livestock manure in the study area. Thus, emission and deposition of NH3 through the atmosphere within the area was an important internal flow of the N cycle in this intensive dairy farming area.
Acknowledgments
This study was partly supported by a Grant-in-Aid for Scientific Research (A) 19201008 provided by the Ministry of Education, Culture, Sports, Science and Technology, Japan; the Japan Grassland Agriculture and Forage Seed Association; and the bilateral project “Comparative Study of Nitrogen Cycling and Its Impact on Water Quality in Agricultural Watersheds in Japan and China” of the Strategic International Cooperative Program provided by the Japan Science and Technology Agency.
References
- Amon , B , Amon , T , Boxberger , J and Alt , C . 2001 . Emissions of NH3, N2O and CH4from dairy cows housed in a farmyard manure tying stall (housing, manure storage, manure spreading) . Nutr. Cycl. Agroecosys. , 60 : 103 – 113 .
- Arya , SP . 2001 . Introduction to Micrometeorology, 2nd edn. International Geophysics Series , Vol. 79 , 450 London : Academic Press .
- Asman , WAH , Sutton , MA and Schjoerring , JK . 1998 . Ammonia emission, atmospheric transport and deposition . New Phytol. , 139 : 27 – 48 .
- Asman , WAH and Van Jaarsveld , HA . 1992 . A variable resolution transport model applied for NHxin Europe . Atmos. Environ. , 26A : 445 – 464 .
- Balsari , P , Airoldi , G , Dinuccio , E and Gioelli , F . 2007 . Ammonia emissions from farmyard manure heaps and slurry stores: effect of environmental conditions and measuring methods . Biosyst. Eng. , 97 : 456 – 463 .
- Bouwman , AF , Boumans , LJM and Batjes , NH . 2002 . Estimation of global NH3volatilization loss from synthetic fertilizers and animal manure applied to arable lands and grasslands . Global Biogeochem. Cyles , 16 : 1024
- Demmers , TGM , Burgess , LR , Short , JL , Phillips , VR , Clark , JA and Wathes , CM . 1998 . First experiences with methods to measure ammonia emissions from naturally ventilated cattle buildings in the UK . Atmos. Environ. , 32 : 285 – 293 .
- van der Gon , H and Bleeker , A . 2005 . Indirect N2O emission due to atmospheric N deposition for the Netherlands . Atmos. Environ. , 39 : 5827 – 5838 .
- Hayashi , K , Hayakawa , A , Akiyama , H and Yagi , K . 2009b . Measurement of ammonia emission using a dynamic chamber technique: a case study of surface-incorporated manure and ammonium sulfate at an upland field of light-colored Andosol . Soil Sci. Plant Nutr. , 55 : 571 – 581 .
- Hayashi , K , Koga , N and Yanai , Y . 2009a . Effects of field-applied composted cattle manure and chemical fertilizer on ammonia and particulate ammonium exchange at an upland field . Atmos. Environ. , 43 : 5702 – 5707 .
- Hayashi , K , Nishimura , S and Yagi , K . 2006 . Ammonia volatilization from the surface of a Japanese paddy field during rice cultivation . Soil Sci. Plant Nutr. , 52 : 545 – 555 .
- Hayashi , K , Nishimura , S and Yagi , K . 2008 . Ammonia volatilization from a paddy field following applications of urea: rice plants are both an absorber and an emitter for atmospheric ammonia . Sci. Total Environ. , 390 : 485 – 494 .
- Hayashi , K and Yan , X-Y . 2010 . Airborne nitrogen load in Japanese and Chinese agroecosystems . Soil Sci. Plant Nutr. , 56 : 2 – 18 .
- Hojito , M , Hayashi , K , Murano , K and Mori , A . 2006a . The status of wet deposition of nitrogen compounds in an intensive dairy farming area in central Japan . Jpn. J. Soil Sci. Plant Nutr. , 77 : 47 – 52 . in Japanese with English abstract
- Hojito , M , Hayashi , K , Murano , K and Mori , A . 2006b . The status of atmospheric concentrations of ammonia in an intensive dairy farming area in central Japan . Jpn. J. Soil Sci. Plant Nutr. , 77 : 53 – 57 . in Japanese with English abstract
- Koerkamp , PWGG , Metz , JHM Uenk , GH . 1998 . Concentrations and emissions of ammonia in livestock buildings in Northern Europe . J. Agr. Eng. Res. , 70 : 79 – 95 .
- Kulling , DR , Menzi , H Krober , TF . 2001 . Emissions of ammonia, nitrous oxide and methane from different types of dairy manure during storage as affected by dietary protein content . J. Agr. Sci. , 137 : 235 – 250 .
- Kurashima , K , Ota , T Kusaba , T . 1993 . Classification and characteristics of soils at the National Grassland Research Institute . Misc Publ. Natl Grassl Res. Inst. , 3 : 1 – 47 . in Japanese
- Liang , Y , Leonard , JJ , Feddes , JJR and McGill , WB . 2006 . Influence of carbon and buffer amendment on ammonia volatilization in composting . Bioresour. Technol. , 97 : 748 – 761 .
- Matsuda , K , Watanabe , I Wingpud , V . 2005 . Ozone dry deposition above a tropical forest in the dry season in northern Thailand . Atmos. Environ. , 39 : 2571 – 2577 .
- Matsunaka , T , Sentoku , A , Mori , K and Satoh , S . 2008 . Ammonia volatilization factors following the surface application of dairy cattle slurry to grassland in Japan: results from pot and field experiments, Soil Sci . Plant Nutr. , 54 : 627 – 637 .
- Ministry of Agriculture, Forestry and Fisheries . 2001 . “ Agricultural Census 2000 Tochigi Prefecture data CD-ROM Ministry of Agriculture ” . Forestry and FisheriesGovernment of Japanin Japanese .
- Parkinson , R , Gibbs , P , Burchett , S and Misselbrook , T . 2004 . Effect of turning regime and seasonal weather conditions on nitrogen and phosphorus losses during aerobic composting of cattle manure . Bioresour. Technol. , 91 : 171 – 178 .
- Rumburg , B , Mount , GH Filipy , J . 2008 . Measurement and modeling of atmospheric flux of ammonia from dairy milking cow housing . Atmos. Environ. , 42 : 3364 – 3379 .
- Saito , G , Kimura , T and Kurashima , K . 1989 . Estimation of the ammonia volatilization loss from surface-applied cow slurry and development of a method to reduce the loss . Bull. Natl Grassl. Res. Inst. , 41 : 1 – 9 .
- Schulze , ED , De Vries , W Hauhs , M . 1989 . Critical loads for nitrogen deposition on forest ecosystems . Water Air Soil Pollut. , 48 : 451 – 456 .
- Smith , K , Cumby , T , Lapworth , J , Misselbrook , T and Williams , A . 2007 . Natural crusting of slurry storage as an abatement measure for ammonia emissions on dairy farms . Biosyst. Eng. , 97 : 464 – 471 .
- Smith , RI , Fowler , D , Sutton , MA , Flechard , C and Coyle , M . 2000 . Regional estimation of pollutant gas dry deposition in the UK: Model description, sensitivity analyses and outputs . Atmos. Environ. , 34 : 3757 – 3777 .
- Soil Survey Staff . 1999 . Key to Soil Taxonomy , 8th edn , Blacksburg : National Resources Conservation Service, United States Department of Agriculture, Pocahontas Press .
- Sommer , SG , Schjoerring , JK and Denmead , OT . 2004 . Ammonia emission from mineral fertilizers and fertilizes crop . Adv. Agron. , 82 : 557 – 622 .
- Sommer , SG , Zhang , GQ Bannink , A . 2006 . Algorithms determining ammonia emission from buildings housing cattle and pigs and from manure stores . Adv. Agron. , 89 : 261 – 335 .
- Sutton , MA , Erisman , JW , Dentener , F and Moeller , D . 2008 . Ammonia in the environment: from ancient times to the present . Environ. Pollut. , 156 : 183 – 604 .
- Thom , AS . 1971 . Momentum absorption by vegetation . Q. J. Roy. Meteor. Soc. , 97 : 414 – 428 .
- Willers , HC , Derikx , PJL , Ten Have , PJW and Vijn , TK . 1996 . Emission of ammonia and nitrous oxide from aerobic treatment of veal calf slurry . J. Agr. Eng. Res. , 63 : 345 – 352 .
- Wyers , P and Duyzer , J . 1997 . Micrometeorological measurements of the dry deposition flux of sulphate and nitrate aerosol to coniferous forest . Atmos. Environ. , 31 : 333 – 343 .
- Zhang , G , Strom , JS Li , B . 2005 . Emission of ammonia and other contaminant gases from naturally ventilated dairy cattle buildings . Biosyst. Eng. , 92 : 355 – 364 .