Abstract
In the present paper we show how P and NPK fertilizers can be prepared from thermochemically treated sewage sludge ashes (SSA) and which chemical reactions occur during these post-treatment steps. The SSA used for this investigation was treated thermochemically at a temperature of 1,000°C in a rotary kiln after the addition of calcium chloride hydrate or magnesium chloride hydrate to remove heavy metals and to increase the bioavailability of phosphorus (hereafter the products of thermochemical treatment are referred to as SSA-Ca and SSA-Mg). The major P-bearing mineral phase in SSA-Ca was chlorapatite (Ca5(PO4)3Cl), whereas in SSA-Mg the major P-bearing mineral phases were farringtonite (Mg3(PO4)2) and chlorapatite. Transformations of the mineral phases were observed when the P content of the products was adjusted by the addition of phosphoric acid (PA). The products of the reaction of SSA-Ca with PA were brushite (dicalcium phosphate dihydrate) and monocalcium phosphate monohydrate (MCPM). Further reaction products were found after the addition of PA to SSA-Mg: newberyite (MgHPO4·3H2O) and garyansellite ((Mg,Fe)3(PO4)2(OH)1.5·1.5H2O)). In addition, NPK fertilizers were prepared from SSA-Ca by mixing with molten NH4NO3 at approximately 180°C. No reaction occurred with the P-bearing mineral phase chlorapatite. When SSA-Ca was spiked with MCPM (to adjust the P content) before mixing it with molten NH4NO3, water-soluble NH4H2PO4 (monoammonium phosphate) was detected as a reaction product.
Introduction
In general, the non-renewable resource phosphate rock is used to produce P fertilizer. Owing to significant increases in the price of phosphate rock over the last years and to save limited resources (CitationCordell et al. 2009; CitationGilbert 2009) new options for the production of phosphate fertilizers from secondary resources must be found (CitationRömer 2006). An important secondary phosphate source is sewage sludge. To protect local receiving waters from eutrophication, phosphorus is removed from waste water by enhanced biological phosphorus removal (EBPR) and/or by precipitation with Ca2+, Fe2+, Fe3+ or Al3+ salts in waste water treatment plants (WWTP) and is accumulated in sewage sludge. In the European Union (EU) more than 11 Mio Mg of sewage sludge is generated annually. However, the direct agricultural application of sewage sludge as a P fertilizer has become a controversial issue mainly because of the presence of organic pollutants (e.g. hormones, antibiotics, endocrine disrupters and persistent organic pollutants) and heavy metals.
In the 6th EU Framework (FP6) project SUSAN (Sustainable and Safe Re-use of Municipal Sewage Sludge for Nutrient Recovery) (CitationSUSAN 2009) a two-step thermal process was developed, transferring the important nutrient phosphorus, which is contained in sewage sludge, into mineral phases that are available for plants, and separating the heavy metals (CitationAdam et al. 2007). In the first step the sewage sludge is incinerated (mono-incineration) at 800–900°C and all of the organic matter is destroyed, including the organic pollutants. The resulting sewage sludge ashes (SSA) are rich in phosphorus and other nutrients (e.g. calcium, magnesium, potassium), but still contain most of the heavy metals that were present in the sludge. Subsequently, the sewage sludge ashes are thermochemically treated at approximately 1,000°C for approximately 30 min after mixing with calcium chloride hydrate or magnesium chloride hydrate (hereafter the products of this treatment are referred to as SSA-Ca and SSA-Mg, respectively). Heavy metals are effectively removed and new mineral phosphate phases are built up by this type of thermochemical treatment (CitationAdam et al. (2009)). The nutrients present in SSA, most importantly phosphorus, remain in the treated ashes. The Austrian company ASH DEC Umwelt AG (a partner in the SUSAN project) already operates this patented process at a technical scale. The price for the thermochemically treated SSA is approximately 0.80 euro per kg P2O5. With the prospective increase in the phosphate rock price over the next years (Citationvon Horn and Sartorius 2009) phosphate fertilizers from SSA will become competitively priced. In a special campaign ASH DEC produced approximately 200 Mg of a NPK fertilizer that was manufactured by mixing thermochemically treated ash (SSA-Ca) with molten ammonium nitrate. This first charge of a newly designed ash-based NPK fertilizer was tested in agriculture in Hungary and also used for field experiments in Austria (M. Kuderna, 2009, unpubl. data). The improved P-fertilization performance of the thermochemically treated sewage sludge ashes was ascertained using pot and field experiments (CitationAdam et al. 2008, CitationSchick et al. 2008). Pot trials with thermochemically treated ashes (SSA-Mg) showed dry matter yields comparable to those variants fertilized with super phosphate. In general, SSA-Mg had higher fertilization performance than SSA-Ca, most likely because of the different mineral phases formed by the thermochemical treatments.
CitationPeplinski et al. (2009) applied the X-ray diffraction (XRD) technique and analyzed the (trans)formation of mineral phosphate phases accompanying the thermochemical treatment of an aluminium-bearing and iron-bearing sewage sludge ash using as a chlorine donor either calcium chloride hydrate or magnesium chloride hydrate. In SSA-Ca, chlorapatite (Ca5(PO4)3Cl) was detected as the main P-bearing mineral phase with some traces of whitlockite (Ca3(PO4)2). In SSA-Mg, farringtonite (Mg3(PO4)2) and chlorapatite were the main P-bearing mineral phases (given chemical formulae are nominal compositions, whereas the actual composition of these mineral phases can deviate considerably owing to solid solution formation; e.g. Mg can be replaced by Fe).
The present study focuses on aspects relevant for the production of ash-based recycling P and NPK fertilizers of merchantable quality from thermochemically treated sewage sludge ashes. For that, SSA-Ca and SSA-Mg materials were treated with various amounts of phosphoric acid (PA) and, in some cases, with additional additives. Subsequently, the resulting products as well as the initial materials were tested for their suitability for P or NPK fertilizers. Their P solubility in water, in citric acid and in alkaline ammonium citrate solutions (Joulie) was determined and in addition their mineral phase composition was analysed by XRD. Conclusions about the nature of the involved P-bearing or NH4-bearing compounds and the occurring chemical reactions were reached.
Materials and methods
Materials and instrumentation
An aluminum-bearing and iron-bearing, organic pollutant free SSA was used after thermochemical treatment with calcium chloride (SSA-Ca) or magnesium chloride (SSA-Mg). The thermochemical treatment was carried out in a gas-tight, laboratory-scale rotary furnace equipped with a quartz glass reactor (Carbolite HTR 11/150; Ubstadt-Weiher, Germany). For both experiments, a 400 g mixture of SSA with calcium or magnesium chloride hydrate was heated up to 1,000°C at a rate of 20°C min−1, followed by an isothermal segment at this temperature for 30 min. The quartz glass reactor was continuously moved during thermal treatment (alternating rotation of 315° in both directions) and flushed with air at a gas flow rate of 3 L min−1. The off-gas was discharged into a polytetrafluoroethylene (PTFE) tube for cooling and condensation of the heavy metal compounds followed by an acid scrubber for HCl removal. The elemental compositions of the thermochemically treated SSAs were measured by inductively coupled plasma optical emission spectrometry (ICP-OES) (Thermo IRIS Intrepid II XSP in combination with Thermo Timberland IIS Autosampler, Thermo Fisher Scientific GmbH, Dreieich, Germany) after total digestion (HNO3/HCl/HF) in a microwave (mikroPrepA; MLS GmbH, Leutkirch, Germany; heating with 1,000 W; 20 min isotherm segment at 210°C). The mass fractions of the matrix elements and heavy metals and the P solubilities in water and 2% citric acid for the raw and the thermochemically treated SSAs are given in .
Two series of P fertilizers were prepared from SSA-Ca or SSA-Mg by the addition of five different amounts of PA (Geyer Chemsolute, Renningen, Germany, 85%, p.a.) and water. The amount of PA added varied between 0% PA and 100% PA in relation to the masses of SSA-Ca or SSA-Mg used for the trial (see ,). The amounts of water added were adjusted to maintain the water content of the mixtures at a comparable level.
The NPK fertilizers (see ) were prepared by adding different mixtures of SSA-Ca (18% P2O5, 0.6% K), monocalcium phosphate monohydrate ([MCPM] Ca(H2PO4)2·H2O; Alfa-Aesar, Karlsruhe, Germany, 55% P2O5), potassium sulfate (Sigma-Aldrich, Steinheim, Germany, p.a.) and gypsum (Knauf, Iphofen, Germany) to molten ammonium nitrate (Sigma-Aldrich) at approximately 180°C in an oil bath under constant stirring. Gypsum was used only as a filling material to keep constant the total amount of the solid materials added to the melt.
Table 1 Mass fractions of the main elements and heavy metals contained in raw sewage sludge ashes (SSA), SSA-Ca and SSA-Mg, and phosphorus solubility in water and 2% citric acid
Table 2 Mass fractions of P2O5 and CaO contained in sewage sludge ashes (SSA) SSA-Ca-1 to SSA-Ca-5
Samples analyzed by XRD were pulverized and homogenized in a vibratory disc mill using a tungsten carbide grinding set. The XRD measurements were carried out on a Bruker-AXS D-5000 diffractometer (Bruker AXS GmbH, Karlsruhe, Germany) in Bragg-Brentano geometry using a 1.0 mm aperture, a 0.1 mm receiving slit, a sample spinner (0 or 15 r.p.m.), a curved graphite monochromator in the diffracted beam and a scintillation counter. Data were collected in the 2Θ range from 5° to 80° in steps of 0.02° for 40 s per step using copper Kα1,2 radiation with 40 kV × 30 mA. Selected samples were re-measured using cobalt Kα1,2 radiation. Diffraction patterns collected with Cu and Co radiation were found to be identical if represented as ‘intensity versus d-value curves’.
Table 3 Mass fractions of P2O5 and CaO contained in sewage sludge ashes (SSA) SSA-Mg-1 to SSA-Mg-5
Table 4 Amounts of the educts used to prepare the NPK fertilizers
The P solubility in citric acid was measured by ICP-OES after extraction of 5 g sample with 500 mL citric acid (2%) for 30 min in a rotary shaker (35–40 turns per min) and immediate filtration through a phosphate-free filter (according to EU directive 2003/2003 method 3.1.3). The P solubility in water was determined in a similar way using distilled water instead of citric acid (according to EU directive 2003/2003 method 3.1.6). The P solubility according to Joulie (EU directive 2003/2003 method 3.1.5.3) was measured by ICP-OES after extraction of 1 g sample with alkaline ammonium citrate (this solution contains 400 g of citric acid and 153 g of NH3 L–1). Five times 20 mL of the alkaline ammonium citrate solution was added and mixed to a paste followed by settling for approximately 1 min. The supernatant was decanted into a 500 mL graduated flask. By the end of the fifth extraction all product was transferred into the flask. The pestle and mortar were rinsed above the graduated flask with 40 mL of distilled water. The stoppered flask was then shaken for 3 h on the rotary shaker (35–40 turns per min). After 15–16 h the flask was shaken again under the same conditions for 3 h. After this it was filled up to the graduation mark with distilled water and filtered through a dry filter. The total P content measured by ICP-OES after total digestion was used to calculate soluble P (Psoluble/Ptotal).
Results and discussion
Significant removal of the heavy metals Cd, Cu, Pb, Sn and Zn was achieved by thermochemical treatment of SSA with both chlorine donors, magnesium and calcium chloride (see ). Although As, Cr and Ni were not, or just slightly, removed, this result is in accordance with thermodynamic considerations (CitationAdam et al. 2009), their mass fractions were below the limits of the German fertilizer ordinance. The phosphorus content decreased slightly from 9% in the raw ash to 8.1 and 8.5%, respectively, as a result of dilution of the ash by the Ca or Mg stemming from the added chlorine donor. The P solubility in water was not improved by the thermochemical treatment. However, P solubility in citric acid significantly increased, particularly for SSA-Mg, which reached nearly 100% ().
The P and Ca contents of the P fertilizers prepared by mixing SSA-Ca and SSA-Mg with various amounts of PA are listed in and, respectively. Owing to the addition of PA the P2O5 content increased from 18% (SSA-Ca-1) and 19% (SSA-Mg-1) to 37% (SSA-Ca-5 and SSA-Mg-5). At the same time the Ca content decreased as a result of dilution. As a consequence the CaO/P2O5 ratio (w/w) dropped from 1.6 to 0.38 in the SSA-Ca series and from 0.89 to 0.24 in the SSA-Mg series.
The P-bearing mineral phases detected by XRD in SSA-Ca-1 to SSA-Ca-5 are shown in . SSA-Ca-1 (no PA treatment) contained chlorapatite as the main P-bearing mineral phase (for the diffraction pattern see the upper part of ). In addition, in all samples, hematite (Fe2O3) and quartz (SiO2) were found (data not shown). After treatment with small amounts of PA (SSA-Ca-2 and 3), brushite ([DCPD] CaHPO4·2H2O) was mainly formed together with traces of MCPM (for the reactions see EquationEqs (1), Equation(2)).
At higher rates of PA dosing (SSA-Ca-4 and 5) MCPM became the main P-bearing mineral phase (for the diffraction pattern of SSA-Ca-4 see the middle part of ). Close ups of the diffraction patterns of SSA-Ca-1 to SSA-Ca-5, showing the characteristic reflection lines of MCPM and brushite, are displayed in . As chlorapatite was the only P-bearing mineral phase in SSA-Ca-1 (no PA treatment), no reflections of MCPM and brushite were detected in this XRD pattern. The intensity of the brushite reflection increased for SSA-Ca-2 owing to the addition of PA, but subsequently decreased after further additions of PA (SSA-Ca-3 and 4). In SSA-Ca-5, brushite was no longer detected. This decrease in the intensity of the brushite reflection was accompanied by an increase in the MCPM reflection. Within the series SSA-Ca-1 to SSA-Ca-5, the CaO/P2O5 ratio decreased from 1.60 to 0.38 and thus the described results are in agreement with former investigations using the pure watery CaO/P2O5 system (CitationElmore and Farr 1940; CitationD′Jans and Knütter 1953; CitationGmelin 1961).
Table 5 Mineral phases detected by the X-ray diffraction in sewage sludge ashes (SSA) SSA-Ca-1 to SSA-Ca-5
These XRD results fit well with the results of the extraction tests with water and 2% citric acid (). SSA-Ca-1 had a P solubility in water of 0%. After treatment with PA the formed P fertilizers showed an increase in P solubility in water. This can be explained by the formation of MCPM (water-soluble; Finck 1992; CitationRichter 2005), the amount of which increases at higher levels of PA dosing. Furthermore, SSA-Ca-1 had a P solubility in citric acid of 76%. Similar to the P solubility in water, the P solubility in citric acid increased to 100% after treatment with PA as a result of the formation of brushite (CitationRichter 2005) and MCPM, two compounds with a high solubility in citric acid and high plant availability.
The P-bearing mineral phases detected by XRD in SSA-Mg-1 to SSA-Mg-5 are shown in . A section of the diffraction pattern of SSA-Mg-1 (no PA treatment) is displayed in . This sample contained three P-bearing mineral phases: farringtonite (Mg3(PO4)2), chlorapatite and some whitlockite (Ca3(PO4)2). Similar to trial SSA-Ca-2, brushite was formed when 10% PA was added (SSA-Mg-2), see also . The fact that no brushite was detected in SSA-Mg-3 to SSA-Mg-5 can be explained by the lower CaO/P2O5 ratio for the SSA-Mg series compared with the SSA-Ca series (see ). In addition to the above-mentioned calcium phosphates, newberyite (MgHPO4·3H2O) was detected in SSA-Mg-2, which is the product of the reaction of farringtonite with PA (see Eq. Equation3). With increasing amounts of PA (SSA-Mg-3), farringtonite and brushite disappeared and traces of MCPM became visible. The content of MCPM increased intensely with 50% PA added (SSA-Mg-4), see also . In this sample only traces of newberyite were detectable, but the formation of a new magnesium–iron phosphate, garyansellite ((Mg,Fe)3(PO4)2(OH)1.5·1.5H2O) (see Eq. Equation4) was detected. In SSA-Mg-5, garyansellite and MCPM were the only P-bearing mineral phases present.
Figure 1 Sections of the X-ray diffraction patterns of sewage sludge ashes (SSA) (a) SSA-Ca-1 (0% PA), (b) SSA-Ca-4 (50% PA) and (c) SSA-Mg-1 (0% phosphoric acid [PA]). B, brushite; C, chlorapatite; F, farringtonite; M, monocalcium phosphate monohydrate; W, whitlockite; 1, anhydrite; 2, anorthite; 3, hematite; 4, quartz.
![Figure 1 Sections of the X-ray diffraction patterns of sewage sludge ashes (SSA) (a) SSA-Ca-1 (0% PA), (b) SSA-Ca-4 (50% PA) and (c) SSA-Mg-1 (0% phosphoric acid [PA]). B, brushite; C, chlorapatite; F, farringtonite; M, monocalcium phosphate monohydrate; W, whitlockite; 1, anhydrite; 2, anorthite; 3, hematite; 4, quartz.](/cms/asset/4c562b16-ffd8-4ea4-ba31-e5cc3f9b1693/tssp_a_10382835_o_f0001g.gif)
The results of the extraction trials for SSA-Mg-1 to SSA-Mg-5 are shown in . SSA-Mg-1 (no PA treatment) had a P solubility in water of 0%. Similar to the SSA-Ca series, P solubility in water increased with higher amounts of PA. but at a higher level. The higher solubility resulted from the newly formed newberyite, which is, in contrast to farringtonite, chlorapatite and brushite, moderately water soluble. The P solubility in 2% citric acid of SSA-Mg-1 was 93%. This higher P solubility compared with SSA-Ca-1 can be explained by the presence of farringtonite, which has a much better solubility in citric acid than chlorapatite. The good citric acid solubility of magnesium phosphates has previously been described by CitationBar-On and Pelly (1979). Stepwise increases in the amount of PA added (SSA-Mg-2 to SSA-Mg-5) result in 100% P solubility in citric acid (see ) because of the formation of newberyite, garyansellite and MCPM (see ). These results for SSA-Mg variants of ash-based fertilizers are in agreement with previous pot experiments showing that phosphate rock based magnesium phosphates and calcium–magnesium phosphates lead to crop yield responses equivalent to single superphosphate and triple superphosphate (CitationBolland et al. 1992; CitationMondrzak-Rosenberg and Hagin 1964).
Figure 2 Close up of the X-ray diffraction patterns of sewage sludge ashes (SSA) SSA-Ca-1 to SSA-Ca-5 showing diffraction lines characteristic of monocalcium phosphate monohydrate (left) and brushite (right). PA, phosphoric acid.
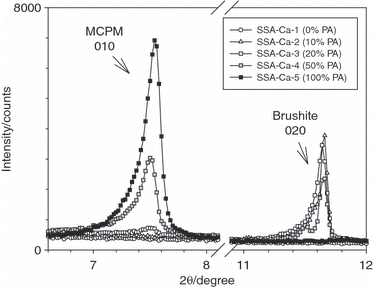
Figure 3 Close up of the X-ray diffraction patterns of sewage sludge ashes (SSA) SSA-Mg-1 to SSA-Mg-5 showing diffraction lines characteristic of monocalcium phosphate monohydrate (left) and brushite (right). PA, phosphoric acid.
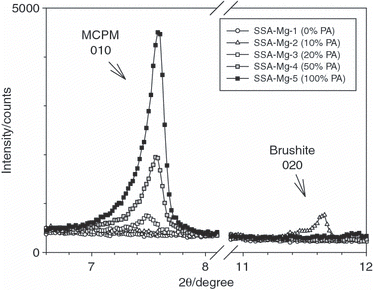
Table 6 Phosphorus solubility in water and citric acid (2%) for sewage sludge ashes (SSA) SSA-Ca-1 to SSA-Ca-5
Table 7 Mineral phases detected by the X-ray diffraction in sewage sludge ashes (SSA) SSA-Mg-1 to SSA-Mg-5
Table 8 Phosphorus solubility in water and citric acid (2%) for sewage sludge ashes (SSA) SSA-Mg-1 to SSA-Mg-5
During treatment of the ashes with PA the release of some HCl gas was observed. Equations Equation1 and Equation2 show the chemical equilibrium of the reactions of chlorapatite with PA to brushite and MCPM, respectively, which are accompanied by the formation of some HCl. Normally the stronger acid moves the weaker acid out of its salts, but hydrochloric acid gas leaves the reaction zone whereby the chemical equilibrium changes to the right side and consequently more brushite and MCPM are formed.
In addition to the investigations on P fertilizers described in the present study, three different NPK fertilizers (NPK-1 to NPK-3) were prepared using mixtures of SSA-Ca and MCPM as the starting materials. The mass fractions of the educts used for their production are given in . These NPK fertilizers were analyzed by XRD and extraction trials with water, citric acid and alkaline ammonium citrate (Joulie) were carried out. NPK-1 contained only one P-bearing educt, SSA-Ca. In NPK-2, 50% of the P input came from SSA-Ca and the other 50% was added in the form of MCPM. For NPK-3, MCPM was the only P-bearing reactant. The following mineral phases were detected for NPK-1 (see ): chlorapatite, nitrammite (NH4NO3), hematite (data not shown), quartz (data not shown) and some traces of gwihabaite ((NH4,K)NO3). A section of the diffraction pattern of NPK-1 is presented in the upper part of and clearly shows that chlorapatite did not react in the molten ammonium nitrate and was still the only P-bearing mineral phase. In contrast, the diffraction pattern of NPK-2 shown at the bottom of contained not only the P-bearing mineral phase chlorapatite, but also a small amount of biphosphammite (monoammonium phosphate [MAP] NH4H2PO4) (see ). Biphosphammite is formed from MCPM and NH4NO3 according to Eq. Equation5:
Furthermore, in NPK-2, (NH4,K)NO3 and some (NH4)5(NO3)3SO4 were detected as newly formed ammonium-bearing mineral phases. In addition, the diffraction pattern showed reflections of bassanite (CaSO4·0.5 H2O) and anhydrite (CaSO4), which were added as a filling material. NPK-3, which was prepared using MCPM as the only P source, contained biphosphammite as the only P-bearing mineral phase. Similar to NPK-2, gwihabaite ((NH4,K)NO3), (NH4)5(NO3)3SO4, arcanite (K2SO4) and bassanite were also detected. In , close ups of the diffraction patterns of NPK-1 to NPK-3 are displayed. shows the reflection of biphosphammite, which is the product of the reaction between MCPM and ammonium nitrate. Reflections of chlorapatite are shown at the center and bottom of . The XRD results presented in show that in NPK-1 and NPK-2 chlorapatite did not react with the molten ammonium nitrate and in NPK-2 and NPK-3 MCPM was completely converted to biphosphammite, which is water soluble.
The mineral phases detected by XRD in these NPK fertilizers are in accordance with the results of the extraction trials with water and alkaline ammonium citrate (Joulie). However, the XRD results did not reflect the results of the P solubility in citric acid (see ). The P solubility in water for NPK-1 was 0% because the only P-bearing mineral phase chlorapatite is insoluble in water. With the addition of MCPM the P solubility in water increased to 30% (NPK-2) and 93% (NPK-3), which resulted from increasing mass fractions of biphosphammite, a water-soluble and plant-available phosphate (CitationRichter 2005; CitationSikora and Mullins 1995), the reaction product of MCPM and ammonium nitrate. The results of the extraction trials according to Joulie were in agreement with the P solubility in water resulting in 3, 30 and 65% P solubility (NPK-1 to NPK-3, respectively).
The P solubility in citric acid of NPK-1 and NPK-3 was almost 100%. In contrast, NPK-2 had a P solubility of 85% only. This result does not reflect the lower solubility of chlorapatite (present in NPK-1 as the only P-bearing mineral phase) compared with the water-soluble biphosphammite that was present in traces in NPK-2, in addition to chlorapatite. The lower value could be a result of the interaction of chlorapatite with calcium ions from the additive calcium sulfate present in NPK-2. In contrast to NPK-2 and NPK-3, calcium sulfate was not used as a filling material for the preparation of NPK-1 (see ). Thus, in the case of NPK-1 the P solubility in citric acid was not depressed by a surplus of Ca ions. In contrast, in the case of NPK-3, water-soluble biphosphammite was the only P-bearing mineral phase and thus its solubility in citric acid was not influenced by a surplus of Ca ions coming from the added calcium sulfate. In previous papers, CitationBraithwaite et al. (1989), CitationBolan et al. (1990) and CitationChien (1993) described similar phenomena for phosphate rocks. They found that high Ca concentrations can depress the dissolution of apatite-based Ca phosphates in citric acid only. Investigations at our institute have shown that in the case of apatite-based P fertilizers and SSA-based products, determination of P solubility in citric acid is sensitive to the solid/liquid ratio, the duration of the extraction and the temperature. However, this particular issue was not investigated in detail in the present study.
Figure 4 Sections of the X-ray diffraction patterns of (a) NPK-1 and (b) NPK-2. A, arcanite; C, chlorapatite; G, gwihabaite; N, nitramite; P, biphosphamite; S, (NH4)5(NO3)3SO4; 1, anhydrite; 3, hematite; 4, quartz; 5, bassanite.
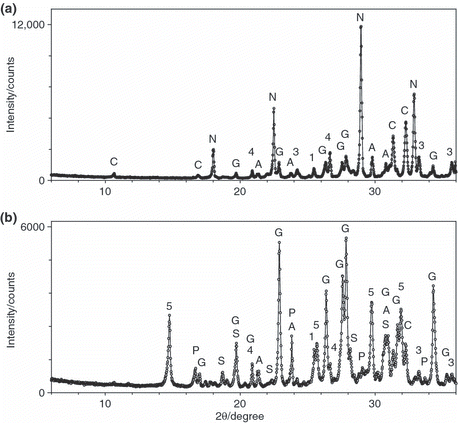
Figure 5 Close up of the X-ray diffraction patterns of three NPK fertilizers (♦, NPK-1, NPK-2, ○, NPK-3) showing diffraction lines characteristic of (a) biphosphammite and (b,c) chlorapatite.
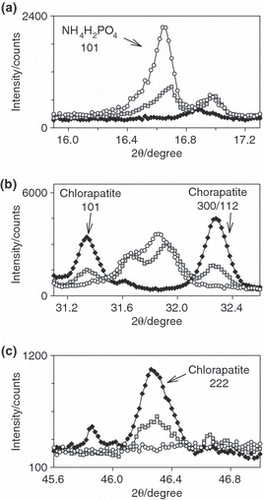
Table 9 Mineral phases detected by the X-ray diffraction in the fertilizers NPK-1 to NPK-3
Table 10 Phosphorus solubility in water and citric acid (2%) for the fertilizers NPK-1 to NPK-3
Conclusions
The present investigation showed that for thermochemically treated sewage sludge ashes of SSA-Ca or SSA-Mg improved P solubility in water, citric acid and alkaline ammonium citrate solutions (Joulie) can be achieved if the ashes are submitted to a post-treatment with PA and, in some cases, with additional additives. During the manufacturing process of P and NPK fertilizers, phase transformations took place that probably have a major influence on the solubility and plant availability of the final fertilizers.
During the preparation of P fertilizers, the formation of brushite (CaHPO4·2H2O) and water-soluble MCPM (Ca(H2PO4)2·H2O) was observed for both SSA-Ca and SSA-Mg. The formation of MCPM was found to proceed in SSA-Mg even at lower levels of PA dosing than in SSA-Ca. In addition, after PA treatment of SSA-Mg the formation of two magnesium-bearing phosphates was observed.
The preparation of NPK fertilizers from SSA-Ca using only ammonium nitrate and potassium sulfate in the melt led to no chemical reaction of the P-bearing mineral phase chlorapatite. However, when MCPM was used as an additional or as the only P source, biphosphammite (NH4H2PO4) was formed at the expense of chlorapatite.
Acknowledgments
The authors wish to acknowledge assistance and support from the partners of the SUSYPHOS consortium and financial support from the European Commission within the 7thEU Framework Programme for Research and Technological Development (FP7 Eurostars, SUSYPHOS E!4314).
References
- Adam , C , Kley , G and Simon , FG . 2007 . Thermal Treatment of Municipal Sewage Sludge Aiming at Marketable P-Fertilizers . Mater. Trans. , 48 : 3056 – 3061 .
- Adam , C , Peplinski , B , Kley , G , Kratz , S , Schick , J and Schnug , E . 2008 . Phosphorrückgewinnung das Klärschlammaschen – Ergebnisse aus dem EU-Projekt SUSAN . Österr Wasser und Abfallwirtschaft , 60 ( 3-4 ) : 55 – 64 .
- Adam , C , Peplinski , B , Michaelis , M , Kley , G and Simon , FG . 2009 . Thermochemical Treatment of Sewage Sludge Ashes for Phosphorus Recovery . Waste Manag. , 29 ( 3 ) : 1122 – 1128 .
- Bar-On , P and Pelly , I . 1979 . Production of magnesium phosphate from apatite and carallite. 2. solubility in citric acid . J. Agric. Food. Chem. , 27 : 152 – 157 .
- Bolan , NS , Hedley , MJ , Harrison , R and Braithwaite , AC . 1990 . Influence of manufacturing variables on characteristics and the agronomic value of partially acidulated phosphate fertilizers . Fertilizer Res. , 26 : 119 – 138 .
- Bolland , MDA , Glencross , RN , Gilkes , RJ and Kumar , V . 1992 . Agronomic effectiveness of partially acidulated rock phosphate and fused calcium-magnesium phosphate compared with superphosphate . Fertilizer Res. , 32 : 169 – 183 .
- Braithwaite , AC , Eaton , AC and Groom , PS . 1989 . Some factors associated with the use of the extractants 2% citric acid and 2% formic acid as estimors of available phosphorus in fertilizer products . Fertilizer Res. , 19 : 175 – 181 .
- Chien , SH . 1993 . Solubility assessment for fertilizer containing phosphate rock . Fertilizer Res. , 35 : 93 – 99 .
- Cordell , D , Drangert , JO and White , S . 2009 . The story of phosphorus: global food security and food for thought . Glob. Environ. Change , 19 : 292 – 305 .
- D′Jans , J and Knütter , R . 1953 . Zur Abgrenzung der Existenzgebiete der Dicalciumphosphate und des Apatites im System H2O-Ca(OH)2-H3PO4bei 25°C . Angew. Chem. , 65 : 578 – 581 .
- Elmore , KL and Farr , TD . 1940 . Equilibrium in the System Calcium Oxide – Phosphorus Pentoxide – Water . Ind. Eng. Chem. , 32 : 581 – 586 .
- Fink , A . 1992 . “ Dünger und Düngung ” . Weinheim, , Germany : VCH Verlagsgesellschaft .
- Gilbert , N . 2009 . The disappearing nutrient . Nature , 461 : 716 – 718 .
- Gmelins Handbuch der anorganischen Chemie1961Ca [B], 1133
- Richter , C . 2005 . “ Agrikulturchemie und Pflanzenernährung ” . Roßdorf, , Germany : Margraf Publishers .
- von Horn , J and Sartorius , C . 2009 . “ Impact of supply and demand on the price development of phosphate (fertilizer) ” . In International Conference on Nutrient Recovery from Wastewater Streams , Edited by: Ashley , K , Mavinic , D and Koch , F . 45 – 49 . London : IWA Publishing .
- Mondrzak-Rosenberg , G and Hagin , J . 1964 . Comparison and evaluation of several phosphatic fertilizers . Isr. J. Agric. Res. , 14 : 37 – 44 .
- Peplinski , B , Adam , C , Michaelis , M , Kley , G , Emmerling , F and Simon , FG . 2009 . Reaction sequences in the thermochemical treatment of sewage sludge ashes revealed by X-ray powder diffraction – A contribution to the European project SUSAN . Z Kristallogr. Suppl. , 30 : 459 – 464 .
- Römer , W . 2006 . Vergleichende Untersuchungen zur Pflanzenverfügbarkeit von Phosphat aus verschiedenen P-Recycling-Produkten im Keimpflanzenversuch . J. Plant Nutr. Soil Sci. , 169 : 826 – 832 .
- Schick , J , Kratz , S , Adam , C , Hermann , L , Kley , G , Schnug , E , WEHBlum , MH and Gerzabek , M . Agronomic potential of P-fertilizers made from sewage sludge ashes – The EU-Project SUSAN Proceedings of EUROSOIL 2008 . August 25–29 2008 , Vienna. Vodrazkap 28.G.01
- Sikora , FJ and Mullins , GL . 1995 . Bioavailability of citrate-insoluble phosphorus in monoammonium phosphate and triple superphosphate fertilizer . Soil Sci. Soc. Am. J. , 59 : 1183 – 1188 .
- SUSAN 2009 (Accessed 25 February 2010 http://www.susan.bam.de (http://www.susan.bam.de)