Abstract
The aim of the present study was to investigate the relationship between the uptake of phosphorus (P) from rock phosphate (RP) by plants and the enhancement of the biological properties of the soil by compost amendments. In experiments conducted in Wagner’s pots (200 cm2), the enrichment of Andosols with RP at 0, 100, or 200 mg P kg−1 soil was combined with the addition of one of four types of compost at a rate equivalent to 13.3 mg P kg−1 soil or with no compost treatment, and the amended soils were used to grow African millet (Eleusine coracana Gaertn, cv. Yukijirushi) for 60 days. The composts added to the soil were based on poultry manure (PM), cattle manure (CM), sewage sludge (SS), or P-adjusted sawdust (PSD). In an incubation experiment, RP was added at 0 or 150 mg P kg−1 of soil in combination with each of the four types of compost at the same rate of application as in the pot experiment, or with no compost. The uptake of P from RP (RP-P) by plants was highest with PM, followed by CM and PSD, and then SS; it was four- to five-fold more with compost addition than no compost addition. Microbial biomass P was significantly higher when RP was added with PM or CM. RP-P uptake by plants showed a positive correlation (r = 0.72, P < 0.05) with the microbial biomass P in the soil. In the soil incubation, the population density of phosphate-solubilizing bacteria (PSB) was significantly higher in compost-amended soils than in the absence of compost treatment, and was highest with the addition of PM. The available P in soil showed a positive correlation (r = 0.70, P < 0.05) with the population density of PSB. These results show that the uptake of P from RP by plants is enhanced by amendment with compost, especially PM or CM compost, and is strongly related to the biological properties of the soil, such as the microbial biomass P and the population density of PSB.
Introduction
Phosphorus (P) is the least mobile of the major plant nutrients, and is unavailable to plants under most soil conditions (CitationKhan et al. 2006). The utilization of applied fertilizer is particularly low in many Andosols, because these soils are rich in allophanes that contain active aluminum and show a high retention of P (CitationLukito et al. 1998). Crops cultivated on Andosols therefore require a continuous supply of P, because the accumulated P exists mainly in a nonlabile inorganic form such as Al- and Fe-bound P (CitationHirata et al. 1999).
Although rock phosphate (RP) mainly consists of carbonated fluoroapatite, RP is an inorganic source of phosphate that could be useful as a replacement for chemical P fertilizers. Conventionally, RP is chemically processed by treatment with sulfuric acid to give a water-soluble chemical phosphate fertilizer (CitationXiao et al. 2008), because the application of unprocessed RP is less effective than application of chemical phosphate fertilizers, particularly in soils that have a high P sorption capacity, a low cation-exchange capacity, a high pH, a low content of organic matter, and a low microbial activity (CitationVassilev and Vassileva 2003). The use of soil microorganisms to improve the availability of RP is considered to be a more environmentally friendly technique than chemical treatment.
A deficiency of P in plants as a result of a shortage of labile P could be avoided through solubilization of P from RP added to the soil or accumulated soil P. Effects of organic matter in enhancing the availability of soil P have been reported by various researchers (CitationKouno et al. 2002; CitationLee et al. 1990; CitationWu et al. 2007). The density of microorganisms is high in soils amended with organic matter because readily available substrates are released into the soil (CitationNishio and Kusano 1980). The solubilization of phosphate through the production of organic acids (citric, oxalic, lactic, and succinic acids) by phosphate-solubilizing bacteria (PSB) has been demonstrated in media supplemented with insoluble phosphate (CitationFankem et al. 2006; CitationHu et al. 2006). The commonly isolated PSB generally belong to the genera Bacillus or Pseudomonas (CitationChen et al. 2006; CitationPeix et al. 2004). In addition, a number of fungal species solubilize P; these species generally belong to the genera Aspergillus or Penicillium (CitationReyes et al. 1999; CitationVazquez et al. 2000). In terms of plant nutrition, PSB are considered to form one of most important groups of microorganisms among the various functional groups of soil microorganisms (CitationChen et al. 2006). Therefore, the stimulation of PSB, a greater turnover of soil P, and the production of organic acids that dissolve inorganic phosphates could be expected to occur in soils that are amended with compost.
The chemical and biological composition of the particular type of compost added could affect the biological properties of the soil. The soil bacterial community structures were significantly affected by organic C content and C/N ratio of the added organic matter (CitationMarschner et al. 2003). CitationHameeda et al. (2008) found that the soil amendments of PSB isolated from composts were efficient in enhancing plant growth. However, the relationships between the biological properties of compost-amended soils and the bioavailability of P have not been studied in detail. In particular, interactions between the soil microbial community and the microbial community in the compost have received little attention. CitationDilly et al. (2004) made use of recent developments in culture-independent methods to explore possible relationships between compost amendments, and the soil microbial community. These techniques of molecular biology offer new opportunities for analyzing changes in the microbial communities of soils.
CitationAyaga et al. (2006) found that the availability of fertilizer added to a low-input cropping system was significantly influenced by enhanced biological cycling. A better understanding of the relationship between enhancement of soil biological properties through addition of organic amendments and plant P uptake could be useful in increasing utilization by plants of P from phosphate fertilizer added to the soil or from accumulated P present in the soil.
This study was conducted to investigate the effects of compost amendment of Andosols on the utilization of RP by plants, and to identify the relationship between the uptake of P from RP by plants and the enhancement of the biological properties of soil by means of compost amendments.
Materials and methods
Soils, composts, and rock phosphate
The soil that had never received any P fertilizer amendment was collected from the Miyoshi area, Hiroshima prefecture, Japan. The soil is classified as an Andosols in the Food and Agriculture Organization of the United Nations (FAO) soil classification. The chemical and physical properties of the soil are listed in .
The four types of compost added to the soil were poultry manure compost (PM), cattle manure compost (CM), sewage sludge compost (SS), and P-adjusted sawdust compost (PSD). The weight of sawdust compost incorporated into the soil was controlled by mixing it with autoclaved crab waste in a ratio of 1:1 by weight to minimize the effects of the treatment on the physical and chemical properties of the soil. Composts were crushed to a particle size of <2 mm before application. The chemical and biological properties of the various composts are presented in .
The various types of compost were obtained from composting centers located in the Hiroshima Prefecture. Basically, they were produced by composting in heaps that were turned periodically during 2–3 months until they reached maturity. The PM compost was made from poultry manure and poultry litter. The CM compost was produced from manure and straw bedding from dairy cattle. The stabilized sewage sludge manure was the product of a municipal wastewater treatment facility, and the sawdust compost was produced from residue of culture media for Maitake (Grifola frondosa) mushrooms.
Table 1 Chemical and physical properties of Andosols used in the experiment
Table 2 Chemical and biological properties of compost used as compost-amendment treatments in the experiment
Rock phosphate was obtained from China and was ground to a particle size of <1 mm before application. The total P content in the RP was 150 g P kg−1 RP by weight, and the citric acid-soluble P content was 17.4 g P kg−1 RP.
Treatments and experimental design: Pot experiment
In the pot experiment, RP was added at a rate of 0, 100, or 200 mg P kg−1 soil. This was combined with one of four compost-amendment treatments (PM, CM, SS or PSD) or with no compost (NC) treatment. Each type of compost was added to the soil at a rate equivalent to 13.3 mg P kg−1 soil (0.43 g of PM, 1.30 g of CM, 0.78 g of SS, or 0.98 g of PSD kg−1 of soil). A chemical fertilizer (CF) treatment with P (KH2PO4) added at the rate of 200 mg P kg−1 soil was used as a control. Each of the treatments except the CF was supplemented with dry coffee residue (CR) (CR; 49% carbon [C] and 0.08% P (w/w) as a C source at a rate of 1500 mg C kg–1 soil. Each Wagner’s pot (200 cm2) was filled with an amount of Andosols equivalent to 1.5 kg of dry soil, and the experiments were conducted with three replicates. To ensure that other nutrients were not limited, the soil in each pot was supplemented with N as (NH4)2SO4 (0.2 g N kg−1 soil), K as K2SO4 (0.07 g K kg−1 soil) and dolomite (0.7 g dolomite kg−1 soil). African millet (Eleusine coracana Gaertn, cv. Yukijirushi) was sown at a rate of 100 seeds per pot. The pots were kept in a glasshouse at 25°C from 28 September to 29 November 2007.
Plant shoots and roots were sampled separately, dried at 80°C for at least 48 h, weighed, and stored for chemical analysis. Soil samples taken at the completion of the pot experiments were sieved (<2 mm) after sampling. Samples were either stored at 4°C for subsequent biological analysis or air dried for chemical analysis.
Incubation experiments
Soils were untreated or treated with RP at 150 mg P kg−1 soil, and these treatments were combined with one of four types of compost amendment treatments (PM, CM, SS, or PSD) or with no compost (NC) treatment. Composts were added to the soil at a rate equivalent to 13.3 mg P kg−1 soil (the same rate of application as in the pot experiment). The soil was also amended with dry CR as a C source at a rate of 3000 mg C kg−1 soil together with an aliquot each of N as (NH4)2SO4 (0.2 g N kg−1 soil) and K as K2SO4 (0.07 g K kg−1 soil). The soil water content was adjusted to 50% of the water-holding capacity (WHC) (). The WHC was determined as explained by CitationBremner and Shaw (1958). The experiments were conducted with three replicates for each treatment.
Portions of Andosols equivalent to 50 g on an oven-dried basis were placed in 120-mL glass bottles, and each bottle was placed in a 1-L jar containing 10 mL of distilled water. The samples were incubated in darkness at 25°C. The CO2 evolved by soil microorganisms during the incubation was trapped by 1 m aqueous NaOH placed inside the jar. Soil samples were taken before incubation and on the 5th, 10th, and 20th days of incubation. Samples were subsequently stored either at 4°C for soil biological and chemical analyses, or at −20°C for the analysis of the structure of the bacterial community.
Chemical analysis
Plant samples
For P determination, plant samples (300 mg) were digested until clear with a 1:1 mixture of concentrated H2SO4 and concentrated HNO3 (10 mL) in a Kjeldahl flask.
Soil, compost, and rock phosphate samples
Soil, compost, and RP samples (200 mg each) were digested with concentrated HNO3 (8 mL) for 1 h in a microwave digester system (Start D, Milestone, Sorisole, Italy) for chemical analysis. Available P from the soil was extracted with 0.5 m aqueous NaHCO3 (pH 8.5) (CitationOlsen et al. 1954). Wet compost (2 g dry basis) was extracted with water (100 mL) for analysis of water-soluble P (CitationKleinman et al. 2007). RP (1 g) was extracted with 2% aqueous citric acid (100 mL) for 30 min for the determination of citric acid-soluble P (CitationBraithwaite et al. 1989). The P contents of the extracts were then determined spectrophotometrically (UVmini-1240, Shimadzu) by the ammonium molybdate–ascorbic acid method (CitationMurphy and Riley 1962). The pH of the soil (1:2.5 w/v of water) or the compost (1:5 w/v of water) was measured using a pH meter (Horiba, F-14, Kyoto, Japan) with a glass electrode.
The total C and N contents of soil and compost samples were determined by a combustion method with a CN analyzer (Yanaco, MT-700, Kyoto, Japan).
Calculations
Within each of the five compost treatments, the uptake of P from RP (RP-P) by plants was calculated as follows:
The RP-P uptake efficiency was calculated as the proportion of RP-P taken up by plants relative to the total amount of RP-P added to the soil. This was calculated as follows:
Biological analysis: Microbial biomass phosphorus
Microbial biomass P was extracted from the soil samples collected at the end of the pot experiments. The biomass P was measured as described by CitationBrookes et al. (1982). Briefly, soil samples were weighed separately into three 50-mL glass vials. The first set was extracted with Olsen reagent and the second set with Olsen reagent spiked with P added as KH2PO4 equivalent to 25 μg P g−1 oven-dry soil. The third set was fumigated with ethanol-free chloroform for 24 h at 25°C. The fumigant was removed, and the sample was extracted with Olsen reagent. All extractions were carried out after fumigation by using a 1:20 soil/Olsen reagent ratio and subsequent shaking at 150 rpm in an orbital incubator for 30 min at 25°C. Biomass P was estimated as the difference between the inorganic P of the fumigated soil and that of the non-fumigated soil. Soil samples collected from the pot experiment, which were stored under refrigeration, were pre-conditioned at room temperature for one week before biomass P extraction.
Analysis of microbial density
To determine the populations of PSB, serially diluted samples of compost and soil were plated onto National Botanical Research Institute phosphate (NBRIP) growth medium (CitationNautiyal 1999), and those bacterial colonies that were surrounded by clear zones were counted as PSB. The NBRIP medium (1 L) consisted of 10 g of glucose, 5 g of Ca3(PO4)2, 5 g of MgCl2·6H2O, 0.25 g of MgSO4·7H2O, 0.2 g of KCl, and 0.1 g of (NH4)2SO4. The pH of the media was adjusted to 7.0 before autoclaving. The NBRIP medium was supplemented with 100 μg mL−1 of cycloheximide (Sigma-Aldrich, St. Louis, MO, USA) (CitationJorquera et al. 2008) to prevent growth of fungi. To determine the size of the total bacterial population, serially diluted compost samples were plated onto nutrient agar (Sigma-Aldrich).
Analysis of the structure of the microbial community
The structures of the microbial communities in compost and in compost-amended soil were determined by amplification of the 16S rDNA for the total bacterial community by polymerase chain reaction (PCR), followed by denaturing gradient gel electrophoresis (DGGE). All analyses were conducted with two replicates for each sample.
DNA extraction
DNA was extracted from 0.4 g of soil or 0.2 g of compost by using an ISOIL for Beads Beating kit (Nippon Gene Co. Ltd., Tokyo, Japan), and samples were purified according to the manufacturer’s instructions.
Polymerase chain reaction
Bacterial 16S rDNA was amplified from extracted DNA by the PCR technique. The primer pair 341F (GC) and 518R (CitationMuyzer et al. 1993) was used for amplification of the variable V3 region. The thermal cycle for the PCR was as follows: initial activation of polymerase at 95°C for 9 min, followed by one cycle of denaturing at 94°C for 1 min, annealing at 65°C for 1 min, and extension at 72°C for 3 min. Nineteen such cycles were performed with a decrease in the annealing temperature of 1°C every second cycle until a temperature of 55°C was reached. Ten additional cycles were carried out at 55°C, and the extension phase of the final cycle was at 72°C for 10 min. The mixtures (50 μL) for the PCR reaction consisted of 0.5 μm of each primer, 200 μm of each dNTP, 2.5 mm of MgCl2, 5 μL of 10× PCR buffer, 1.25 units of AmpliTaq Gold (Applied Biosystems, Foster City, CA, USA), and 1 μL of DNA template.
The PCR amplification was carried out with a Gene-Amp PCR System 2700 (Applied Biosystems). PCR products were verified and quantified by agarose gel electrophoresis with a standard in the gel (Loading Quick; Toyobo, Osaka, Japan).
Denaturing gradient gel electrophoresis
Samples from the compost-treated soil and from the composts were loaded onto a single gel for comparison of their DGGE fragments. DGGE was conducted using a DCode System (Bio-Rad Laboratories, Hercules, CA, USA) with an 8% (w/v) polyacrylamide gel (acrylamide/bisacrylamide, 37.5:1) in 1× TAE (Tris base/acetic acid/ethylenediaminetetraacetic acid [EDTA]) buffer. A denaturing gradient ranging from 30% to 70% was produced with 80% denaturant solution containing 5.6 m urea and 32% formamide. After each lane of the gel was loaded with 20 μL of the PCR products, electrophoresis was performed at a constant temperature of 60°C and a constant voltage of 100 V for 16 h. After electrophoresis, the gels were stained with SYBR Gold nucleic acid gel stain (Molecular Probes, Eugene, OR, USA). Gel images and photographs were recorded by using a UV transillumination system with a Print-graph AE-6932GXCF (ATTO Corporation, Tokyo, Japan).
Statistical analysis
Data were statistically analyzed by using Minitab statistical software, release 14 (Minitab Inc., State College, PA, USA). Results, by treatment, were analyzed by one-way analysis of variance (anova). Dunnett’s method was used to compare the control and treatments, and Fisher’s least significant difference method was used to identify treatments for which the mean values differed from one another at a 5% level of significance (P < 0.05).
Results
Plant dry matter and phosphorus uptake
The total plant (shoots and roots) dry matter (DM) increased with increasing rate of application of RP, and the increase was greater with compost treatments than in their absence (NC treatment) (). The increase in DM was highest in the PM treatments followed by CM and PSD, and then by SS.
Shoot or root P concentrations showed no significant variation with the rate of application of RP. However, the P concentrations in shoots and roots were higher with compost treatments than with NC treatment. The P concentration in the shoots ranged from 668 to 771 mg P kg−1 DM with compost treatments, and from 538 to 559 mg P kg−1 DM without compost treatment (NC).
The P uptake by plants was also greater with compost treatments than without it, although it was not significantly different among the compost treatments at 0 mg P of RP (). However, uptakes of P by plants with compost treatments were significantly lower than those with CF treatment.
The uptake of P was significantly increased by PM treatment at 100 mg P of RP, and by all compost treatments at 200 mg P of RP. RP-P uptake was four- to five-fold greater with compost treatment than with NC treatment at 200 mg P of RP. The RP-P uptake was 1.12 mg pot−1 in the PM treatment; this fell in the order CM ≈ PSD > SS > NC, and was 24–44% higher with PM treatment than with the other compost treatments.
RP-P uptake efficiency
The RP-P uptake efficiency was 0.06% at an RP application rate of 100 mg P of RP, and 0.07% at an RP application rate of 200 mg P of RP (). However, the RP-P uptake efficiency was greatly improved in the compost-amended soil, and was 0.53% with PM treatment, 0.45% with CM treatment, 0.31% with SS treatment, and 0.24% with PSD treatment at 100 mg P of RP. At an RP application rate of 200 mg P of RP, the RP-P uptake efficiency was 0.37% with PM treatment, 0.30% with CM or PSD treatments, and 0.26% with SS treatment.
Table 3 Shoots and roots dry weight (DM) of African millet (Eleusine coracana) grown with four compost treatments and a non-compost control at three rates of rock phosphate (RP) application
Figure 1 Uptake of P by African millet (Eleusine coracana) grown at three rates of rock phosphate (RP) application with no-compost (NC), chemical fertilizer (CF), and four different compost treatments; CM, cattle manure; PM, poultry manure; PSD, phosphorus-adjusted sawdust; SS, sewage sludge. Error bars represent standard deviation (n = 3). Asterisks indicate significant difference to the non-RP-amended treatment within each compost treatment group (Dunnett’s test, P < 0.05).
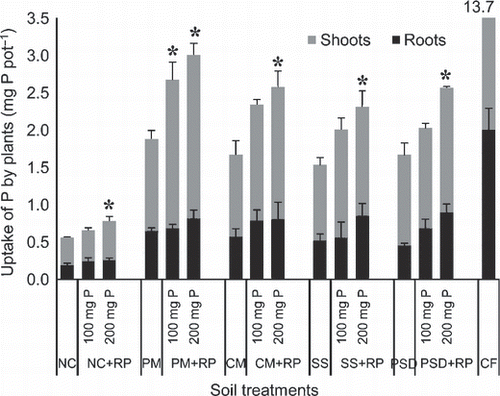
Microbial biomass phosphorus
In the absence of added RP, levels of microbial biomass P did not differ significantly between the compost treatments and the NC treatment (). However, microbial biomass P increased with the addition of RP, and the increase was greater with compost treatments than with NC treatment. The microbial biomass P for the PM and CM treatments was significantly higher than that for other treatments at an RP application rate of 200 mg P of RP.
Population density of phosphate-solubilizing bacteria
The population density of PSB was 0.02 × 105–1.5 × 105 cfu g−1 soil, and this increased sharply to between 7 × 105 and 19 × 105 cfu g−1 soil during the 5th through the 10th days of soil incubation (). The population density of PSB was also greater with compost treatments than with NC treatment. The population density of PSB declined to between 2 × 105 and 13 × 105 cfu g−1 soil during the 10th through the 20th days of incubation, and the decline was sharper with NC treatment than with compost treatments. The population density of PSB was slightly higher in the RP-amended soil than in the non-RP-amended soil.
Table 4 Effect of compost amendments on uptake of P from rock phosphate (RP-P) and RP-P uptake efficiency for African millet (Eleusine coracana) at two rates of RP addition
Bacterial community structure analysis with PCR-DGGE
The band positions of the DGGE profiles generated by the various types of compost showed the existence of differences in the structures of the bacterial community present in the various composts (data not shown). However, distinct differences in 16S rDNA banding patterns among the compost treatments could not be identified from the DGGE profiles generated from the pot experiment or from the incubation experiment (data not shown).
Table 5 Soil microbial biomass P with four compost treatments and a non-compost control at three rates of rock phosphate (RP) application in the pot experiment
Table 6 Population density of phosphate-solubilizing bacteria (PSB) with four compost treatments and a NC in the untreated or treated with rock phosphate (RP) at 150 mg P kg−1 soil (+RP) during soil incubation
Discussion
The amounts of P uptake in the NC and compost treatments were significantly lower than that which occurred with CF treatment at the standard rate of application of P (). This shows that the experiment was conducted under conditions of low available P. Plant P uptake was extremely low in the NC treatment, and increased slightly with application of RP. Plant growth in the NC treatments was inhibited by the limited availability of P in the soil.
Our results show that the increase of P uptake in the compost amended soils did not differ significantly because each compost that was applied contained a similar amount of P, and the composts were applied to the soil at a very low rate (<0.13% w/w). However, we found that the plant P uptake increased with RP amendment and the increase differed significantly among the various compost treatments. The availability of soil P is largely controlled by biologically mediated processes (CitationZou et al. 1992). For example, the biological mobilization of mineral phosphate is carried out by organic acids, either by a reduction in the pH or by chelation of the cations associated with P (CitationKhan et al. 2006). The addition of compost to the soil is known to increase the microbial biomass and various soil activities (CitationCrecchio et al. 2001; CitationNayak et al. 2007). We also observed that there were no significant differences in the initial soil pH values (1:2.5 of H2O) of compost-amended soils in the incubation experiment, and that the pH gradually decreased from 5.1–5.3 to 4.3–4.5 during the incubation period.
We therefore assumed that biological differences among the compost treatments had significant effects on the variations in the uptake of RP-P by plants. The differences in RP-P uptake by plants were compared by calculating the RP-P uptake efficiency, and were examined in relation to the soil microbial biomass P, the structures of the microbial community, and the population densities of PSB.
Efficiency of uptake of phosphorus from rock phosphate
The RP-P uptake efficiency was greatly improved in the compost-amended soil, and was highest in the PM treatment, followed by CM and PSD (which were similar), and then by SS treatments (). The RP-P uptake efficiency declined at higher rates of application of RP in the PM, CM, and SS treatments. The RP-P uptake efficiency was inversely proportional to the rate of application of RP. This result is supported by the findings of CitationBolland and Barrow (1998), who found that the relative effectiveness of RP declined as its rate of application was increased.
Uptake of phosphorus from rock phosphate and soil microbial biomass phosphorus
The relationship between RP-P uptake by plants and soil microbial biomass P was examined by means of the pot experiments. The difference in RP-P uptake by plants was positively correlated to the microbial biomass P content (r = 0.72; P < 0.05) (). Microbial biomass P has a strong relation with ability of soils to supply P, because microbial biomass is an important component of the labile P in the soil (CitationKwabiah et al. 2003). CitationAyaga et al. (2006) also suggested that the flow of P from inorganic fertilizer P to plant P through microbial P has a significant influence on plant P uptake, especially when available P is low.
Figure 2 Relationship between uptake of P from rock phosphate (RP-P) by plants and soil microbial biomass P. The relationship was examined by means of the pot experiment.
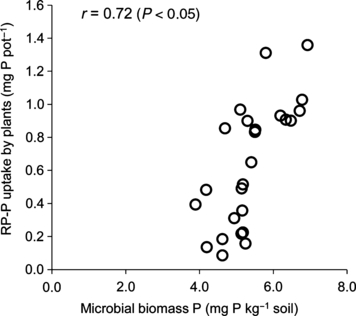
Levels of microbial biomass P did not differ significantly between compost treatments with no RP amendment. However, microbial biomass P was significantly higher in the PM and CM treatments than in the other treatments with RP amendment (). CitationJorquera et al. (2008) suggested that the bioavailability of P depends on the ability of soil microbial biomass to immobilize non-labile forms of soil P. We can therefore say that RP-P is efficiently immobilized by microorganisms in the PM and CM treatments.
The possibility of altering the resident microflora in soil by inoculations of microorganisms has been demonstrated by CitationSrivastava et al. (2007). The four types of compost contained different population densities of PSB () and showed differences in their microbiological contents (data not shown). There is a high possibility that the different microbial communities of the composts can alter the resident microflora of compost-amended soil. For example, CitationRivera-Cruz et al. (2008) have reported that application of a biofertilizer can successfully introduce PSB into a soil.
Uptake of phosphorus from rock phosphate and the structure of the microbial community
No clear differences in microbial communities among the various compost treatments could be identified from the DGGE profiles generated from the pot experiment or from the incubation experiment. CitationCrecchio et al. (2004) also showed that the compost treatments did not affect the structure of soil microbial community. However, significant differences in the RP-P uptake by plants and in the soil microbial biomass P were observed with the various treatments. The differences in the PSB population density were also observed with the various treatments in the incubation experiment. The diversity of the microbial communities of the soil may have been underestimated because of the low ability of DGGE to resolve all the sequences of the partial small subunit (SSU) rRNA genes.
The generation of DGGE profiles with specific primer sets for PSB might produce more appropriate results for the differences in the microbial community for the various treatments. The presence of two genes (gdh and pqqE) involved in P solubilization has been detected in PSB by using specific primer sets (CitationPerez et al. 2007). These primers were originally used on isolated bacteria for which P solubilization was confirmed by using culture media. Further studies are therefore needed to confirm that these primers would be suitable for use with soil DNA extracts in population studies on PSB.
Available phosphorus in soils and the population density of phosphate-solubilizing bacteria
In our experiments, the population density of PSB was greater in the compost-amended soil, especially with PM or PSD compost amendments. CitationKavitha and Subramanian (2007) reported that the population density of PSB was greater in a municipal solid waste enriched with composted poultry litter, microbial inoculants, and rock phosphate. The PSB population density of PM- and CM-amended soil was slightly increased by RP amendment, although the difference was not statistically significant.
Figure 3 Relationship between available P (Olsen) in soil and population density of phosphate-solubilizing bacteria (PSB). The relationship was examined by means of the population density of PSB at the 10th day of the incubation experiment.
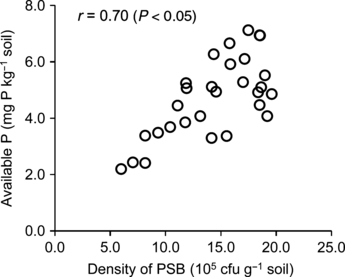
The conditions that promote a high population density of PSB would be expected to enhance the availability of P in a soil, because PSB will solubilize more insoluble phosphate than is required for their growth and metabolism (CitationJorquera et al. 2008). The results of soil-incubation experiment showed that the differences in the PSB population density were positively correlated (r = 0.70, P <0.05) with the available P in the soil (). The population density of PSB is therefore an important biological property of a soil that is strongly related to the available P of soils amended with RP.
The present work has shown that the combined application of RP and compost enhances RP-P utilization by plants. Compost amendment enhances the microbial biomass P and the population density of PSB. The biological properties enhanced by the compost amendment are strongly related to plant P uptake and soil available P.
In conclusion, utilization of RP by plants is enhanced by amendment of the soil with compost. Among the compost amendments, PM amendment produced the greatest enhancements of the RP-P uptake and of the biological properties of the soil. The uptake of P from RP by plants was strongly related to the biological properties of the soil, particularly the microbial biomass P and the population density of PSB.
Acknowledgments
We gratefully acknowledge the helpful suggestions of Dr Jun Wasaki, Hiroshima University, who also provided the technical facilities for the PCR-DGGE experiment. The authors would like to acknowledge the financial support of the Ministry of Education, Sports, Culture, Science and Technology, Japan.
References
- Ayaga , G , Todd , A and Brookes , PC . 2006 . Enhanced biological cycling of phosphorus increases its availability to crops in low-input sub-Saharan farming systems . Soil Biol. Biochem. , 38 : 81 – 90 .
- Bolland , MDA and Barrow , NJ . 1988 . Effect of level of application on the relative effectiveness of rock phosphate . Fertil. Res. , 15 : 181 – 192 .
- Braithwaite , AC , Eaton , AC and Groom , PS . 1989 . Some factors associated with the use of the extractants 2% citric acid and 2% formic acid as estimator of available phosphorus in fertilizer products . Fertil. Res. , 19 : 175 – 181 .
- Bremner , JM and Shaw , K . 1958 . Denitrification in soil. I. Methods of investigation . J. Agric. Sci. Camb. , 51 : 22 – 39 .
- Brookes , PC , Powlson , DS and Jenkinson , DS . 1982 . Measurement of microbial biomass phosphorous in soil . Soil Biol. Biochem. , 14 : 319 – 329 .
- Chen , YP , Rekha , PD , Arun , AB , Shen , FT , Lai , WA and Young , CC . 2006 . Phosphate solubilizing bacteria from subtropical soils and their tricalcium solubilizing abilities . Appl. Soil Ecol. , 34 : 33 – 41 .
- Crecchio , C , Curci , M , Mininni , R , Rucciuti , P and Ruggiero , P . 2001 . Short-term effects of municipal solid waste compost amendments on soil carbon and nitrogen content, some enzyme activities and genetic diversity . Biol. Fertil. Soils , 34 : 311 – 318 .
- Crecchio , C , Curci , M , Pizzigallo , MDR , Ricciuti , P and Ruggiero , P . 2004 . Effects of municipal solid waste compost amendments on soil enzyme activities and bacterial genetic diversity . Soil Biol. Biochem. , 36 : 1595 – 1605 .
- Dilly , O , Bloem , J , Vos , A and Munch , JC . 2004 . Bacterial diversity in agricultural soils during litter decomposition . Appl. Environ. Microbiol. , 70 : 468 – 474 .
- Fankem , H , Nwaga , D , Deubel , A , Dieng , L , Merbach , W and Etoa , FX . 2006 . Occurrence and functioning of phosphate solubilizing microorganisms from oil palm tree (Elaeis guineensis) rhizosphere in Cameroon . Afr. J. Biotechnol. , 5 : 2450 – 2460 .
- Hameeda , B , Harini , G , Rupela , OP and Reddy , G . 2008 . Growth promotion of maize by phosphate-solubilizing bacteria isolated from composts and macrofauna . Microbiol. Res. , 163 : 234 – 242 .
- Hirata , H , Watanabe , K , Fukushima , K , Aoki , M , Imamura , R and Takahashi , M . 1999 . Effect of continues application of farm yard manure and inorganic fertilizer for 9 years on changes in phosphorus compounds in plow layer of an upland Andosol . Soil Sci. Plant Nutr. , 45 : 577 – 590 .
- Hu , X , Chen , J and Guo , J . 2006 . Two phosphate- and potassium-solubilizing bacteria isolated from Tianmu Mountain, Zhejiang, China . World J. Microbiol. Biotechnol. , 22 : 983 – 990 .
- Jorquera , MA , Hernandez , MT , Rengel , Z , Marschner , P and Mora , ML . 2008 . Isolation of culturable phosphobacteria with both phytate-mineralization and phosphate-solubilization activity from the rhizosphere of plants grown in a volcanic soil . Biol. Fertil. Soils , 44 : 1025 – 1034 .
- Kavitha , R and Subramanian , P . 2007 . Bioactive compost – A value added compost with microbial inoculants and organic additives . J. Appl. Sci. , 7 : 2514 – 2518 .
- Khan , MS , Zaidi , A and Wani , PA . 2006 . Role of phosphate solubilizing microorganisms in sustainable agriculture – A review . Agron. Sustain. , 26 : 1 – 15 .
- Kleinman , P , Sullivan , D Wolf , A . 2007 . Selection of water extractable phosphorus test for manures and biosoilds as an indicator of runoff loss potential. Technical report: waste management . J. Environ. Qual. , 36 : 1357 – 1367 .
- Kouno , K , Wu , J and Brookes , PC . 2002 . Turnover of biomass C and P in soil following incorporation of glucose and ryegrass . Soil Biol. Biochem. , 34 : 617 – 622 .
- Kwabiah , AB , Palm , CA , Stoskopf , NC and Voroney , RP . 2003 . Response of soil microbial biomass dynamics to quality of plant materials with emphasis on P availability . Soil Biol. Biochem. , 35 : 207 – 216 .
- Lee , D , Han , XG and Jordan , CF . 1990 . Soil phosphorus fractions, aluminum and water retention as affected by microbial activity in an Ultisol . Plant Soil , 121 : 125 – 136 .
- Lukito , HP , Kouno , K and Ando , T . 1998 . Phosphorus requirements of microbial biomass in a Regosol and an Andosol . Soil Biol. Biochem. , 30 : 865 – 872 .
- Marschner , P , Kandeler , E and Marschner , B . 2003 . Structure and function of the soil microbial community in a long-term fertilizer experiment . Soil Biol. Biochem. , 35 : 453 – 461 .
- Murphy , J and Riley , JP . 1962 . A modified single solution method for the determination of phosphate in natural waters . Anal. Chim. Acta , 27 : 31 – 36 .
- Muyzer , G , Waal , EC and Uitterlinden , AG . 1993 . Profiling of complex microbial populations by denaturing gradient gel electrophoresis analysis of polymerase chain reaction-amplified genes coding for 16S rRNA . Appl. Environ. Microbiol. , 59 : 695 – 700 .
- Nautiyal , CS . 1999 . An efficient microbial growth medium for screening phosphate solubilizing microorganisms . FEMS Microbiol. Lett. , 170 : 265 – 270 .
- Nayak , DR , Babu , YJ and Adhya , TK . 2007 . Long-term application of compost influences microbial biomass and enzyme activities in a tropical Aeric Endoaquept planted to rice under flooded condition . Soil Biol. Biochem. , 39 : 1897 – 1906 .
- Nishio , M and Kusano , S . 1980 . Fluctuation patterns of microbial numbers in soil applied with compost . Soil Sci. Plant Nutr. , 26 : 581 – 593 .
- Olsen , SR , Cole , CV , Watanabe , FS and Dean , LA . 1954 . “ Estimation of available phosphorus in soils by extraction with sodium bicarbonate ” . USDA Circulation No. 939 119 Washington : Government Printing Office .
- Peix , A , Rivas , R Santa-Regina , I . 2004 . Pseudomonas luteasp. nov., a novel phosphate-solubilizing bacterium isolated from the rhizosphere of grasses . Int. J. Syst. Evol. Microbiol. , 54 : 847 – 850 .
- Perez , E , Sulbaran , M , Ball , MM and Yarzabal , LA . 2007 . Isolation and characterization of mineral phosphate-solubilizing bacteria naturally colonizing a limonitic crust in the south-eastern Venezuelan region . Soil Biol. Biochem. , 39 : 2905 – 2914 .
- Reyes , I , Bernier , L , Simard , RR and Antoun , H . 1999 . Effect of nitrogen source on the solubilization of different inorganic phosphates by an isolate of Penicillium rugulosumand two UV-induced mutants . FEMS Microb. Ecol. , 28 : 281 – 290 .
- Rivera-Cruz , MC , Narcia , AT , Ballona , GC , Kohler , J , Caravaca , F and Roldan , A . 2008 . Poultry manure and banana waste are effective biofertilizer carriers for promoting plant growth and soil sustainability in banana crops . Soil Biol. Biochem. , 40 : 3092 – 3095 .
- Sekiya , K . 1970 . “ Phosphoric acid (in Japanese) ” . In Analysis methods for measuring soil fertility , Edited by: Ishizawa , S . 251 – 253 . Tokyo : Yokendo co. Ltd .
- Srivastava , R , Roseti , D and Sharma , AK . 2007 . The evaluation of microbial diversity in a vegetable based cropping system under organic farming practices . Appl. Soil Ecol. , 36 : 116 – 123 .
- Vassilev , N and Vassileva , M . 2003 . Biotechnological solubilization of rock phosphate on media containing agro-industrial wastes . Appl. Microbiol. Biotechnol. , 61 : 435 – 440 .
- Vazquez , P , Holguin , G , Puente , ME , Lopez-Cortes , A and Bashan , Y . 2000 . Phosphate solubilizing microorganisms associated with the rhizosphere of mangroves in a semiarid coastal lagoon . Biol. Fertil. Soils , 30 : 460 – 468 .
- Wu , J , Huang , M Xiao , HA . 2007 . Dynamics in microbial immobilization and transformation of phosphorus in highly weathered subtropical soil following organic amendments . Plant Soil , 290 : 333 – 342 .
- Xiao , CQ , Chi , RA , Huang , XH , Zhang , WX , Qiu , GZ and Wang , DZ . 2008 . Optimization for rock phosphate solubilization by phosphate-solubilizing fungi isolated from phosphate mines . Ecol. Eng. , 33 : 187 – 193 .
- Zou , X , Binkley , D and Doxtader , KG . 1992 . A new method for estimating gross phosphorus mineralization and immobilization rates of soil . Plant Soil , 147 : 243 – 250 .