Abstract
Twenty rice cultivars, including three genetically-distinct groups (japonica, indica-1, and indica-2), were evaluated for their response to inoculation with Azospirillum sp. strain B510 in paddy fields with standard nitrogen (SN) and low nitrogen (LN) fertilization. In the SN field, the tiller numbers in most indica-2 cultivars, 37 days after transplanting (DAT), were significantly increased by the B510 inoculation, whereas those in 4 japonica cultivars were significantly decreased. A similar growth response was observed in the LN field, although the impacts of the B510 inoculation were more varied than in the SN field. At 58 DAT, the tiller numbers in most cultivars were lower or unaffected by the B510 inoculation under both SN and LN conditions, except that the tiller number of the Nipponbare cultivar, which is classified as japonica, was significantly higher in the LN field only. These results suggest that the effects of inoculation with Azospirillum sp. strain B510 on the growth of rice plants, especially on tiller numbers at the early growth stage, vary depending on the rice genotype, as well as nitrogen level. Therefore, the plant genotypes, growth stages, and fertilization managements must be considered when a plant-associated bacterium is evaluated for beneficial effects under field conditions.
Introduction
A wide range of microorganisms, including fungi and bacteria, has been found in the phytosphere (CitationMano and Morisaki 2008; CitationSaito et al. 2007, 2008). Among these, plant growth-promoting rhizobacteria (PGPR), which have beneficial effects on host plants, such as increased crop yield, have been well characterized from both scientific and practical perspectives.
The genus Azospirillum, characterized by spirillum-shaped, N2-fixing, Gram-negative alphaproteobacteria, contains species often found in the rhizosphere (CitationHartman and Baldani 2006). Inoculation with Azospirillum sp. promotes plant growth, so agronomic applications of this genus have been developed (CitationOkon and Labandera-Gonzalez 1994). Plant growth promotion using various strains of Azospirillum has been investigated on several plant species. Wheat plants treated with Azospirillum brasilense Sp245 exhibited reduced root length, increased root–hair formation, and increased above-ground biomass and ear numbers (CitationSpaepen et al. 2008). In maize, seed inoculation with Azospirillum lipoferum CRT1 induced changes in root morphology, such as increased length and biomass (CitationEl Zemrany et al. 2007). In tomatoes, Azospirillum brasilense Sp7 and Azospirillum sp. BNM-65 exhibited positive effects, such as increased shoot and root biomass (CitationRomero et al. 2003). The total nitrogen content in rice grains was improved by inoculation with Azospirillum brasilense REC3 under low nitrogen (LN) fertilization conditions (CitationPedraza et al. 2009). Treatment with a nitrogen-fixing strain of Azospirillum lipoferum to the root zone of rice plants promoted the early tillering and reproductive growth of host plants, although the total dry weight and nitrogen content in the rice plants were not markedly increased by the inoculation (CitationWatanabe and Lin 1984).
Azospirillum sp. strain B510 was isolated from a surface-sterilized stem of a rice plant (Oryza sativa cv. Nipponbare [NI]) that was grown in an experimental paddy field (CitationElbeltagy et al. 2001). The B510 strain is closely related to Azospirillum oryzae COC8 (97.7% identity in their 16S rRNA gene sequences), which was reported as a paddy soil bacterium (CitationXie and Yokota 2005). In addition to being a diazotroph under free-living conditions, B510 was found to have positive motility and to be capable of degrading plant cell walls (CitationElbeltagy et al. 2001). Inoculation with Azospirillum sp. B510 was shown to promote plant growth under both laboratory and field conditions (CitationIsawa et al. 2010). Noticeably, the field experiment in Hokkaido, Japan indicated that inoculation with B510 increases tiller number, resulting in an increase in seed yield at commercial levels (CitationIsawa et al. 2010). Moreover, the B510 inoculation enhanced disease resistance to fungal and bacterial pathogens (CitationYasuda et al. 2009). A genome analysis of B510 has been completed and revealed that the B510 strain possesses putative plant hormone-related genes, which could promote plant growth (CitationKaneko et al. 2010). Thus, Azospirillum sp. strain B510 is a likely beneficial plant-associated bacterium for agronomic applications.
Plant genotype is known to influence the plant growth-promotion effects of inoculation with Azospirillum (CitationBashan and Holguin 1997). For example, significant effects on growth and yield of maize plants were observed in one bacterium–plant combination and absent from another combination, indicating an unknown interaction between plant genotype and bacterial strain (CitationGarcia De Salomone and Dobereiner 1996). Similar phenomena were reported when local Nepalese wheat varieties and mustard were inoculated with local Azospirillum spp. (CitationBhattarai and Hess 1993; CitationKesava Rao et al. 1990).
It is also known that fertilization management can affect the community structures of plant-associated bacteria (CitationCoelho et al. 2009; CitationIkeda et al. 2010; CitationRoesti et al. 2006). There is debate regarding the impacts of fertilization strategies on the effects of PGPR, such as Azospirillum species. The plant growth promotion was observed only under LN conditions in some studies (CitationDobbelaere et al. 2001; CitationFallik and Okon 1996), whereas other studies showed that the use of nitrogen in combination with Azospirillum produced significantly higher green and dry-matter yields (CitationChela et al. 1993; CitationOkon and Labandera-Gonzalez 1994). Furthermore, little is known about the cross-effects of rice genotypes and nitrogen fertilization levels on plant growth promotion by Azospirillum sp. under field conditions. Therefore, the aim of the present study was to examine the cross-effects of rice genotype and nitrogen level on the response of rice plants to inoculation with Azospirillum sp. B510 under paddy field conditions.
Materials and methods
Plant materials and growth conditions
Plant materials were obtained from the National Institute of Agrobiological Sciences (NIAS) Genebank (Tsukuba, Japan). In total, 20 rice cultivars (10 cultivars each for japonica and indica types) were used (). The 10 indica-type cultivars were classified into two subgroups (indica-1 and indica-2), based on a principal coordinate analysis using 179 restriction fragment length polymorphism data (CitationEbana et al. 2008; CitationKanemura et al. 2007; CitationKojima et al. 2005; CitationUga et al. 2009).
Seeds from each cultivar were placed on two layers of filter paper (Toyo Roshi Kaisha, Tokyo, Japan) in a Petri dish (6-cm diameter) containing 4 mL tap water. The Petri dishes were placed in an incubator at 30°C. After 2 days (30 April 2009), the germinated seeds were sown in a commercial soil (Mitsui-Toatsu no. 3, Tokyo, Japan) in a 60 × 30 cm cell tray (cell diameter, 1.5 cm; depth, 3 cm) and grown in a greenhouse under natural light conditions for 4 weeks. After 3 weeks in the greenhouse, selected seedlings of each cultivar were inoculated with Azospirillum sp. strain B510, as described in the next section. A total of 100 seedlings of each cultivar were planted in an experimental field (37°28′N, 141°06′E) in a square pattern (5 × 5 plants). Hills were spaced 30 cm apart. The seedlings inoculated with B510 and those that were not inoculated were cultivated nearby in the same field. Rice seedlings were grown in two neighboring fields: one with standard nitrogen (SN) and the other with LN. Basal fertilizer (phosphorus pentoxide [P2O5] and potassium oxide [K2O] with or without nitrogen) was applied to the paddy fields 4 days before transplanting. In the SN paddy field, nitrogen, P2O5, and K2O (Temairazu 666; Co-op Chemical, Tokyo, Japan) were fertilized at 30, 30, and 30 kg ha−1, respectively. In the LN field, only P2O5 and K2O were added at 30 kg ha−1 each. The LN paddy field has been used for rice cultivation using the same field management procedure employed in the SN field, except that no nitrogen had been applied to the LN field since 2004. To maintain the uniformity of soil conditions in these paddy fields, we cultivated the same rice cultivar uniformly in all areas in 2008. The field soil was classified as gray lowland soil. The characteristics of the field soils used in the present study are shown in .
Table 1 Rice plant materials used this study
Table 2 Characteristics of field soils
Bacterial inoculation and plant growth measurements
Azospirillum sp. strain B510 was cultured in nutrient broth (Difco Laboratories, Detroit, MI, USA) at 30°C for 16 h, and from this culture, a bacterial suspension was prepared in sterile, distilled water (1 × 108 colony-forming units [CFU] mL−1). Three-week-old rice seedlings were inoculated with 500 mL of the bacterial suspension (final density: 1.5 × 106 CFU mL−1 on a tray containing 300 seedlings). After the inoculation, rice seedlings were incubated in a greenhouse for 5 days prior to transplantation to the experimental fields.
The effects of the inoculation on rice growth were evaluated by measuring the shoot length and tiller number of 9 plants for each cultivar with a square pattern (3 × 3 plants) inside the square pattern (5 × 5 plants) with or without inoculation under SN or LN conditions at 37 and 58 days after transplanting (DAT). The statistical difference in plant length or tiller number between non-inoculation and inoculation was determined by two-sided Student’s t-test. P < 0.05 was considered significant. The inoculation effect index (IEI) for the tiller number and shoot length was calculated for each cultivar grown under SN and LN conditions using the following equation:
To evaluate the cross-effects between the inoculation and the nitrogen fertilization levels, the nitrogen effect index (NEI) for the tiller number was calculated for each cultivar without inoculation grown under SN and LN conditions using the following equation:
Results and discussion
We compared the effects of inoculation with Azospirillum sp. strain B510 on the growth of rice under SN and LN paddy field conditions. At 37 DAT in the SN field, the tiller numbers were significantly higher (P < 0.05, t-test) in 8 cultivars (Sekiyama [SE], Khau Mac Kho [KM], Muha [MU], Bei Khe [BE], Ryou Suisan Koumai [RY], Deng Pao Zhai, Naba [NB], and Milyang 23 [MI] in ) inoculated with B510, whereas tiller numbers of 4 cultivars (NI, Koshihikari [KO], Fukkurinko [FU], and Nanatsuboshi [NN]) were significantly lower (; ). In the LN field, the tiller numbers of 4 cultivars (KM, MU, RY, and NB) were increased significantly by inoculation with B510 at 37 DAT, whereas the tiller numbers of 3 cultivars (KO, SE, and Tupa [TU]) were significantly decreased (; ). At 37 DAT, 18 cultivars in the SN field and 10 in the LN field showed noticeable, but not statistically-significant responses (positive or negative) to the B510 inoculation (; ).
Similarly, 11 cultivars in the SN field and 7 in the LN field were noticeably responsive to the inoculation at 58 DAT (; ), with significantly fewer numbers of tiller for 7 cultivars inoculated with B510 in the SN field (NI, FU, Habataki, TU, Kasalath, BE and NB) (; ). At 58 DAT in the LN field, only one cultivar (NI) inoculated with B510 significantly showed a greater number of tillers than those without inoculation, and 2 cultivars (SE and TU) showed a significantly lower number of tillers (; ).In both the SN and LN fields, the effects of the inoculation on shoot length were less clear compared to the effects on tiller numbers (; ). However, as with tiller numbers, more cultivars showed shoot length responses to the inoculation at 37 DAT (; ) than at 58 DAT (; ) in both the SN and LN fields.
Figure 1 Inoculation effect index (IEI, %) for tiller number (a–d) and shoot length (e–h) of rice plants. IEI = (tiller number or shoot length of rice plants inoculated with B510)/(tiller number or shoot length of rice plants without inoculation) × 100. IEI for tiller number (a) 37 days after transplanting (DAT) under standard nitrogen (SN) conditions; (b) 37 DAT under low nitrogen (LN) conditions; (c) 58 DAT, SN; (d) 58 DAT, LN. IEI for shoot length (e) 37 DAT, SN; (f) 37 DAT, LN; (g) 58 DAT, SN; (h) 58 DAT, LN. White and black arrowheads respectively signify that means (n = 9) of tiller number or shoot length of rice plants inoculated with B510 were significantly higher and lower than those of rice plants without inoculation (P < 0.05, t-test). AK, Akage; BA, Basilanon; BE, Bei Khe; BL, Bleiyo; DE, Deng Pao Zhai; DI, Dianyu 1; FU, Fukkurinko; HA, Habataki; KA, Kasalath; KM, Khau Mac Kho; KN, Khao Nam Jen; KO, Koshihikari; MI, Milyang 23; MU, Muha; NB, Naba; NI, Nipponbare; NN, Nanatsuboshi; RY, Ryou Suisan Koumai; SE, Sekiyama; TU, Tupa 121-3.
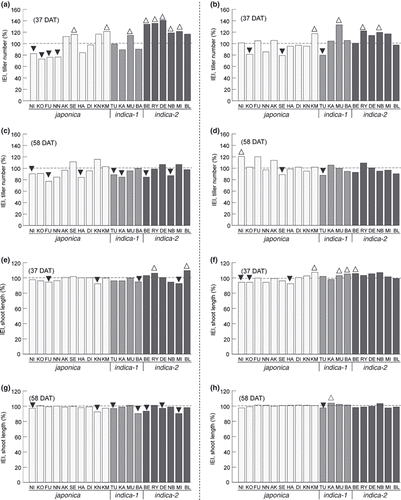
Table 3 Effects of Azospirillum sp. B510 inoculation on tiller number of rice plants
These results suggest that B510 generally has a pronounced influence on rice plant growth under SN fertilization conditions, compared to LN conditions, especially at the early growth stages. Furthermore, the impact of B510 inoculation at the later growth stage seems mostly negative or absent in most cultivars under both SN and LN conditions, except for 3 cultivars (NI, FU, and Akage) that showed a noticeably positive response under LN conditions (). Interestingly, all cultivars belonging to the indica-2 subgroup showed an increase in tiller number in the SN field following inoculation (), and 4 of 6 indica-2 subcultivars in the LN field similarly showed an increase in tiller number, with no negative responses (). These results imply that the indica-2 group might have genetic characteristics responsible for the stable and unidirectional reaction to B510 inoculation at the early growth stage, independent of nitrogen fertilization levels, suggesting the possibility of genetic manipulation for rice and B510 interaction.
In the present study, it was shown that the tiller number was more responsive to inoculation than the shoot length, especially at the early growth stage under SN conditions. The indica-1 and indica-2 subgroups include different ecotypes: indica-1 consists mainly of the aus ecotype, and indica-2 includes various ecotypes other than aus (CitationKojima et al. 2005). The aus ecotype, with early maturity, is adapted to uplands and is expected to have resistance to drought (CitationTsunoda 1987). There are differences in root characteristics between the two groups: the indica-1 group has deeper and thicker roots than the indica-2 group (CitationUga et al. 2009). The genetic and phenotypic differences between indica-1 and indica-2 might have contributed to their unique responses to the B510 inoculation observed in the present study, as the initial interaction between a rice plant and B510 takes place in the rhizosphere through root inoculation. Several previous studies have demonstrated a positive correlation between the concentration of low molecular weight dicarboxylic acids, such as succinate and malate in root exudates, and the plant growth-promotion effects in gramineous plants, including rice (CitationChristiansen-Weniger et al. 1992; CitationGyaneshwar et al. 2002; CitationSuzuki et al. 2009), and the utilization of dicarboxylic acids is considered one of the metabolic characteristics of PGPR (CitationRudrappa et al. 2008). In addition, the genome of Azospirillum sp. B510 possesses multiple symbiotic sugar transporters and a diverse set of malic enzymes, suggesting that B510 can potentially utilize C4-dicarboxylate in its symbiotic relationship with a host plant (CitationKaneko et al. 2010). Thus, root functionality, such as the secretion of dicarboxylic acids, could be affected by both rice genotype and nutrition conditions. Consequently, these environmental factors could synergistically modify and complicate the interaction between Azospirillum sp. B510 and rice plants.
Table 4 Efects of Azospirillum sp. B510 inoculation on shoot length of rice plants
CitationWatanabe and Lin (1984) reported that treatment with Azospirillum lipoferum strain 34H to the root zone of rice plants promoted early tillering and reproductive growth of the host plants. Similarly, the present study showed that the inoculation of Azospirillum sp. strain B510 to rice plants could increase the tiller number. Furthermore, this bacterium has also been shown to increase the tiller number and seed yield of NN under field conditions in Hokkaido, Japan (CitationIsawa et al. 2010), possibly because an increase in tiller number directly results in an increase in seed yield in high latitude areas, such as Hokkaido. The complex relationship between rice plants and B510 could be explained by the fact that Azospirillum sp. can interact with plants through several mechanisms, including the production of growth hormones and the assimilation of nitrogen compounds from soil and air (CitationGarcia De Salomone and Dobereiner 1996). Correspondingly, different plant genotypes can react positively or negatively with a strain of Azospirillum sp. under different environmental conditions. Interestingly, the strong positive reaction under LN conditions was observed for the NI cultivar (). Since the B510 strain was originally isolated from the NI cultivated in the same experimental field used in the present study (CitationElbeltagy et al. 2001), the result might indicate a high affinity between B510 and NI. However, it was observed that tiller numbers of some cultivars decreased by inoculation with B510. CitationLynch (1982) suggested that inoculation of bacteria inhibited growth of plants to compete nutrition in some cases, although the bacteria contributed to the growth as PGPR under other conditions. It has been shown that inoculation of PGPR reduces root volume, depending on plant genotype (CitationDubeikovsky et al. 1993; CitationElliott and Lynch 1985). Therefore, the negative effects of inoculation Azospirillum sp. strain B510 on some cultivars might cause stress by competing for nutrients between rice and B510, or changing root morphology/functionality under the experimental conditions employed.
Figure 2 Correlation analysis between the inoculation effect index (IEI, %) under standard nitrogen (SN) and low nitrogen (LN) field conditions and the nitrogen effect index (NEI, %), for tiller number and shoot length in each genotype of rice plant. (a) IEI and NEI for tiller number 37 days after transplanting (DAT) in the SN field, (b) 37 DAT in the LN field, (c) 58 DAT in the SN field, (d) 58 DAT in the LN field. (e) IEI and NEI for shoot length 37 DAT in the SN field, (f) 37 DAT in the LN field, (g) 58 DAT in the SN field, (h) 58 DAT in the LN field. In (d), the correlation coefficient in parentheses was calculated, excluding Nipponbare. IEI (%) = (tiller number of rice plants inoculated with B510)/(tiller number of rice plants without inoculation) × 100. NEI (%) = (tiller number of rice plants in SN field)/(tiller number in LN field) × 100. *P < 0.01; **P < 0.05.
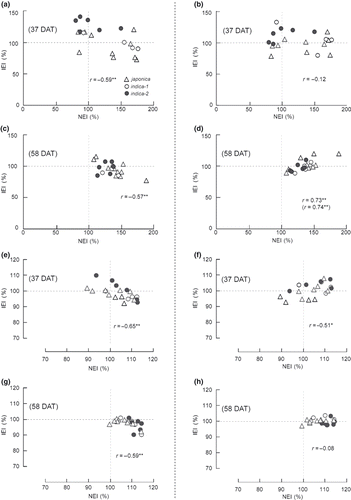
A correlation analysis revealed a significant negative correlation between IEI and NEI for tiller numbers at both 37 and 58 DAT in the SN field (). Some cultivars in the indica-2 subgroups, showing a LN effect, positively responded to inoculation with B510 under SN conditions (). These results suggest that rice genotypes, which have a weak response to nitrogen fertilization, might be positively affected by inoculation with B510 in the SN paddy field. In contrast, there was a significant, positive correlation between IEI and NEI for tiller numbers at 58 DAT in the LN field (). Furthermore, there was no significant correlation between IEI and NEI at 37 DAT in the LN field (). Some japonica rice cultivars showing a higher nitrogen effect positively responded to inoculation with B510 under LN conditions (). This result suggests that rice genotypes, which have a positive response to nitrogen fertilization, might be positively affected by inoculation with B510 in the LN paddy field. Therefore, it is considered that the impact of inoculation with B510 depends on responsiveness to nitrogen fertilization in each rice genotype of rice and the nitrogen level in the paddy field.
In conclusion, the results of the present study suggest that the effects of inoculation with Azospirillum sp. strain B510 on the growth of rice plants, especially on the tiller number at the early growth stage, vary depending on the rice genotype, as well as nitrogen level. Moreover, we observed cross-effects between the rice genotype and nitrogen fertilization level. Therefore, it is necessary to consider plant genotype and growth stage, as well as nitrogen fertilization levels, to evaluate beneficial plant-associated bacteria, such as azospirilla, under field conditions.
Acknowledgments
This work was supported by the Ministry of Agriculture, Forestry, and Fisheries of Japan (Rice Genome Project Grant PMI-0002) and in part by Special Coordination Funds for Promoting Science and Technology. We thank S. Inaba (University of Tohoku, Japan) for her technical advice regarding the analysis of soil characteristics, K. Ebana (National Institute of Agrobiological Science, Tsukuba, Japan) for providing information on rice cultivars, and T. Isawa (Mayekawa Mfg. Co., Ltd.) for his comments on the manuscript.
References
- Bashan , Y and Holguin , G . 1997 . Azospirillum-plant relationships: environmental and physiological advances (1990–1996) . Can. J. Microbiol. , 43 : 103 – 121 .
- Bhattarai , T and Hess , D . 1993 . Yield responses of Nepalese spring wheat (Triticum aestivumL.) cultivars to inoculation with Azospirillumspp. of Nepalese origin . Plant Soil , 151 : 67 – 76 .
- Chela , GS , Tiwana , MS , Thind , IS , Puri , KP and Kaur , K . 1993 . Effect of bacterial cultures and nitrogen fertility on the yield and quality of maize fodder (Zea maysL.) . Ann. Biol. , 9 : 83 – 86 .
- Christiansen-Weniger , C , Groneman , AF and van Veen , JA . 1992 . Associative N2fixation and root exudation of organic acids from wheat cultivars of different aluminium tolerance . Plant Soil , 139 : 167 – 174 .
- Coelho , MRR , Marriel , IE , Jenkins , SN , Lanyon , CV , Seldin , L and O’Donnell , AG . 2009 . Molecular detection and quantification of nifHgene sequences in the rhizosphere of sorghum (Sorghum bicolor) sown with two levels of nitrogen fertilizer . Appl. Soil Ecol. , 42 : 48 – 53 .
- Dobbelaere , S , Croonenborghs , A Thys , A . 2001 . Responses of agronomically important crops to inoculation with Azospirillum . Aust. J. Plant Physiol. , 28 : 871 – 879 .
- Dubeikovsky , AN , Mordukhova , EA , Kochetkov , VV , Polikarpova , FY and Boronin , AM . 1993 . Growth promotion of blackcurrant softwood cuttings by recombinant strain Pseudomonas fluorescens BSP53a synthesizing an increased amount of indole-3-acetic acid . Soil Biol. Biochem. , 25 : 1277 – 1281 .
- Ebana , K , Kojima , Y , Fukuoka , S , Nagamine , T and Kawase , M . 2008 . Development of mini core collection of Japanese rice landrace . Breed. Sci. , 58 : 281 – 291 .
- El Zemrany , H , Czarnes , S , Hallett , PD , Alamercery , S , Bally , R and Jocteur Monrozier , L . 2007 . Early changes in root characteristics of maize (Zea mays) following seed inoculation with the PGPR Azospirillum lipoferumCRT1 . Plant Soil , 291 : 109 – 118 .
- Elbeltagy , A , Nishioka , K Sato , T . 2001 . Endophytic colonization and in plantanitrogen fixation by a Herbaspirillumsp. isolated from wild rice species . Appl. Environ. Microbiol. , 67 : 5285 – 5293 .
- Elliott , LF and Lynch , JM . 1985 . Plant growth-inhibitory pseudomonads colonizing winter wheat (Triticum aestivumL.) roots . Plant Soil , 84 : 57 – 65 .
- Fallik , E and Okon , Y . 1996 . The response of maize (Zea mays) to Azospirilluminoculation in various types of soils in the field . World J. Microbiol. Biotechnol. , 12 : 511 – 515 .
- Garcia De Salomone , I and Dobereiner , J . 1996 . Maize genotype effects on the response to Azospirilluminoculation . Biol. Fertil. Soils , 21 : 193 – 196 .
- Gyaneshwar , P , James , EK , Reddy , PM and Ladha , JK . 2002 . Herbaspirillumcolonization increases growth and nitrogen accumulation in aluminium-tolerant rice varieties . New Phytol. , 154 : 131 – 145 .
- Hartman , A and Baldani , JI . 2006 . In the genus Azospirillum , New York : Springer .
- Ikeda , S , Okubo , T Kaneko , T . 2010 . Community shifts of soybean stem-associated bacteria responding to different nodulation phenotypes and N levels . ISME J , 4 : 315 – 326 .
- Isawa , T , Yasuda , M , Awazaki , H , Minamisawa , K , Shinozaki , S and Nakashita , H . 2010 . Azospirillumsp. strain B510 enhances rice growth and yield . Microbes Environ. , 1 : 58 – 61 .
- Kaneko , T , Minamisawa , K Isawa , T . 2010 . Complete genomic structure of the cultivated rice endophyte Azospirillumsp. B510 . DNA Res. , 17 : 37 – 50 .
- Kanemura , T , Homma , K , Ohsumi , A , Shiraiwa , T and Horie , T . 2007 . Evaluation of genotypic variation in leaf photosynthetic rate and its associated factors by using rice diversity research set of germplasm . Photosynth. Res. , 94 : 23 – 30 .
- Kesava Rao , PS , Arunachalam , V and Tilak , KVBR . 1990 . Genotype-dependent response to Azospirillumtreatment in yield and nitrogenase activity in Brassica juncea . Curr. Sci. , 59 : 607 – 609 .
- Kojima , Y , Ebana , K , Fukuoka , S , Nagamine , T and Kawase , M . 2005 . Development of an RFLP-based rice diversity research set of germplasm . Breed. Sci. , 55 : 431 – 440 .
- Lynch , JM . 1982 . “ Interactions between bacteria and plants in the root environment ” . In Bacteria and plants , Edited by: Rhodes-Roberts , ME and Skinner , FA . 1 – 23 . New York : Academic Press .
- Mano , H and Morisaki , H . 2008 . Endophytic bacteria in the rice plant . Microbes Environ. , 23 : 109 – 117 .
- Okon , Y and Labandera-Gonzalez , CA . 1994 . Agronomic applications of Azospirillum: an evaluation of 20 years worldwide field inoculation . Soil Biol. Biochem. , 26 : 1591 – 1601 .
- Pedraza , RO , Bellone , CH , Carrizo de Bellone , S , Boa Sorte , PMF and Teixeira , KRdS . 2009 . Azospirilluminoculation and nitrogen fertilization effect on grain yield and on the diversity of endophytic bacteria in the phyllosphere of rice rainfed crop . Eur. J. Soil Biol. , 45 : 36 – 43 .
- Roesti , D , Gaur , R Johri , BN . 2006 . Plant growth stage, fertiliser management and bio-inoculation of arbuscular mycorrhizal fungi and plant growth promoting rhizobacteria affect the rhizobacterial community structure in rain-fed wheat fields . Soil Biol. Biochem. , 38 : 1111 – 1120 .
- Romero , AM , Correa , OS , Moccia , S and Rivas , JG . 2003 . Effect of Azospirillum-mediated plant growth promotion on the development of bacterial diseases on fresh-market and cherry tomato . J. Appl. Microbiol. , 95 : 832 – 838 .
- Rudrappa , T , Czymmek , KJ , Pare , PW and Bais , HP . 2008 . Root-secreted malic acid recruits beneficial soil bacteria . Plant Physiol. , 148 : 1547 – 1556 .
- Saito , A , Ikeda , S , Ezura , H and Minamisawa , K . 2007 . Microbial community analysis of the phytosphere using culture-independent methodologies . Microbes Environ. , 22 : 93 – 105 .
- Saito , A , Kawahara , M , Ikeda , S , Ishimine , M , Akao , S and Minamisawa , K . 2008 . Broad distribution and phylogeny of anaerobic endophytes of cluster XlVa clostridia in plant species including crops . Microbes Environ. , 23 : 73 – 80 .
- Spaepen , S , Dobbelaere , S , Croonenborghs , A and Vanderleyden , J . 2008 . Effects of Azospirillum brasilenseindole-3-acetic acid production on inoculated wheat plants . Plant Soil , 312 : 15 – 23 .
- Suzuki , K , Okazaki , K , Tawaraya , K , Osaki , M and Shinano , T . 2009 . GC-MS associated global analysis of rice root exudates under aseptical condition . Soil Sci. Plant Nutr. , 55 : 505 – 513 .
- Tsunoda , S . 1987 . Distribution, properties and phylogenetic relations of Asian upland rice varieties . Southeast Asian Stud. , 25 : 39 – 50 . In Japanese with English summary
- Uga , Y , Ebana , K , Abe , J , Morita , S , Okuno , K and Yano , M . 2009 . Variation in root morphology and anatomy among accessions of cultivated rice (Oryza sativaL.) with different genetic backgrounds . Breed. Sci. , 59 : 87 – 93 .
- Watanabe , I and Lin , C . 1984 . Response of wetland rice to inoculation with Azospirillum lipoferumand Pseudomonassp . Soil Sci. Plant Nutr. , 30 : 117 – 124 .
- Xie , CH and Yokota , A . 2005 . Azospirillum oryzaesp. nov., a nitrogen-fixing bacterium isolated from the roots of the rice plant Oryza sativa . Int. J. Syst. Evol. Microbiol. , 55 : 1435 – 1438 .
- Yasuda , M , Isawa , T , Minamisawa , K , Shinozaki , S and Nakashita , H . 2009 . Effects of colonization of a bacterial endophyte, Azospirillumsp. B510a on disease resistance in rice . Biosci. Biotechnol. Biochem. , 12 : 2595 – 2599 .