Abstract
Cell polarity is essential for various cellular functions during both proliferative and developmental stages, and it displays dynamic alterations in response to intracellular and extracellular cues. However, the molecular mechanisms underlying spatiotemporal control of polarity transition are poorly understood. Here, we show that fission yeast Cki3 (a casein kinase 1γ homolog) is a critical regulator to ensure persistent monopolar growth during S phase. Unlike the wild type, cki3 mutant cells undergo bipolar growth when S phase is blocked, a condition known to delay transition from monopolar to bipolar growth (termed NETO [new end takeoff]). Consistent with this role, Cki3 kinase activity is substantially increased, and cells lose their viability in the absence of Cki3 upon an S-phase block. Cki3 acts downstream of the checkpoint kinase Cds1/Chk2 and calcineurin, and the latter physically interacts with Cki3. Autophosphorylation in the C terminus is inhibitory toward Cki3 kinase activity, and calcineurin is responsible for its dephosphorylation. Cki3 localizes to the plasma membrane, and this localization requires the palmitoyltransferase complex Erf2-Erf4. Membrane localization is needed not only for proper NETO timing but also for Cki3 kinase activity. We propose that Cki3 acts as a critical inhibitor of cell polarity transition under S-phase arrest.
INTRODUCTION
Cell polarity is a fundamental requirement for tissue modeling and a variety of cellular functions. Each cell, derived from various tissues, displays a specific shape, which further undergoes dynamic alterations in the context of cell cycle progression and differentiation processes. These include asymmetric cell division, cell migration, and epithelial-mesenchymal transition (EMT), which eventually lead to multicellular-body morphogenesis (Citation1Citation–Citation3). In principle, the basic rules of tissue morphogenesis lie in the level of polarity within a single cell that displays active cytoskeletal remodeling. However, the molecular mechanisms underlying alterations of cell polarity in response to intracellular cues remain elusive.
The fission yeast Schizosaccharomyces pombe provides an ideal system with which to study the control of cell polarity (Citation4). During the vegetative cycle, rod-like fission yeast cells undergo polarized growth only at the cell tips, with a constant cell width (∼7 to 14 μm and ∼3.8 μm in length and width, respectively). When cells reach a critical size during late G2 phase, mitosis is initiated; cell elongation then ceases and is followed by cell division in the medial region, producing two equal-size daughter cells (Citation5). These newly born cells initially grow exclusively from the old end, which already existed in the previous cycle. The cells maintain this monopolar growth pattern until a certain point during the G2 phase (0.34 of the way through the cell cycle), when they have a length of ∼9.5 μm (Citation6). At this point, the new end, newly emerged through cell division, is somehow activated, resulting in the initiation of growth from this end. Thus, the growth polarity changes from monopolar to bipolar, referred to as NETO (new end takeoff) (Citation6). Several cell morphogenesis mutants have been isolated in the hope of understanding the regulatory mechanisms of cell polarity, and some of them display constitutive monopolar growth patterns, i.e., they are defective in the onset of NETO. Characterization of these NETO mutants is instrumental for determining how NETO physically takes place. A set of mutants deficient in actin and microtubule cytoskeletons, intracellular trafficking, and nutrient-sensing signal transduction pathways fails to progress into NETO (Citation7, Citation8). Consistent with these findings, it has been shown that transient treatment of fission yeast cells with latrunculin A, an actin polymerase inhibitor, induces NETO in cells that grow in a monopolar manner (Citation9). Also, it is known that a fusion protein formed between the microtubule-associated protein (MAP) Tea1 and the actin polymerase formin For3 is capable of triggering NETO in monopolar cells (Citation10).
Despite these previous studies, the regulatory mechanism by which the timing of NETO is set at a specific cell cycle stage remains unexplored. A pioneering work by Mitchison and Nurse (Citation6) proposed that there are two requirements for NETO to take place: DNA replication and a critical cell length. We previously showed that calcineurin, the Ca2+/calmodulin-regulated type 2B protein phosphatase, plays a crucial role in NETO delay before DNA replication is completed (Citation11). We found that when DNA replication is halted by hydroxyurea (HU) treatment or a mutation in DNA polymerase α, the checkpoint kinase Cds1 (a Chk2 homolog) delays the transition of growth polarity from monopolar to bipolar, as well as mitotic entry. Cds1 phosphorylates calcineurin, which in turn dephosphorylates the microtubule-plus-tip-associated protein Tip1 (a CLIP170 homolog) (Citation12). Tip1 subsequently modulates microtubule dynamics at the two cell tips in an asymmetrical manner, which is crucial for the NETO delay (Citation11). Despite this work, the mechanism of signal transduction from the two cell tips to the growth polarity transition remains to be established.
Casein kinase 1 (CK1) is a ubiquitous protein kinase involved in numerous cellular processes (Citation13, Citation14). This family of kinases is composed of seven isoforms in mammals (Citation13, Citation14). The kinase domains are highly conserved among these isoforms; however, the regulatory mechanisms of kinase activity and cellular localization vary among the isoforms (Citation13, Citation14). CK1γ is a unique member of the CK1 family in that, unlike other members, it becomes localized to the plasma membrane. This membrane localization relies on the C-terminal double-cysteine motif (C-C), which is posttranslationally modified by palmitoylation (Citation15). In higher eukaryotes, CK1γ plays essential roles in developmental processes, which are mainly performed via spatial regulation of the Wnt signaling pathway and phospholipid-mediated asymmetrical cell division (Citation15, Citation16). In budding yeast, Yck1 and Yck2 also are localized to the plasma membrane in a manner mediated by the palmitoyltransferase Akr1 (Citation17Citation–Citation19), and this localization is essential for their functions (Citation19, Citation20).
Here, we show that Cki3, a fission yeast homolog of CK1γ, is required for delaying NETO when DNA replication is inhibited. Cki3 acts downstream of Cds1 and calcineurin but in parallel with Tip1. We describe the multiple regulatory mechanisms modulating Cki3 activities, which include palmitoylation-mediated membrane localization, inhibitory autophosphorylation, and calcineurin-dependent dephosphorylation. Also, we discuss the roles of Cki3 as a safeguard in retaining cell polarity when the DNA replication checkpoint is activated.
MATERIALS AND METHODS
Yeast general methods.
Standard media and methods for S. pombe were used (Citation21, Citation22). The fission yeast strains used in this study are listed in Table S1 in the supplemental material. Gene deletion and tagging were carried out by a PCR-based method using homologous recombination at the corresponding genomic loci (Citation22). Site-directed mutagenesis was performed with a PrimeStar mutagenesis basal kit (TaKaRa). The mutated cki3 gene, including its own promoter and terminator sequences, was subcloned into the integrating plasmid pJK148 carrying the leu1+ gene, and the resulting plasmid was linearized by digestion with NdeI within the leu1+ gene and integrated into the leu1-32 locus. In C-terminal tagging for cki3SS, cki34A, or cki34E, mutated forward primers were used (CTAAGCCTGCGCCCTCGAAAGAGAAATCACGAAAGAAGTTCCATCTTCGCTTACTGTCTTCCTCCATATCGAAACAAGAAcggatccccgggttaattaa, CTAAGCCTGCGCCCGCGAAAGAGAAAGCACGAAAGAAGTTCCATCTTCGCTTACTGTCTTGCTGCATATCGAAACAAGAAcggatccccgggttaattaa, and CTAAGCCTGCGCCCGATAAAGAGAAAGATCGAAAGAAGTTCCATCTTCGCTTACTGTCTTGCTGCATATCGAAACAAGAAcggatccccgggttaattaa). Nucleotides that were mutated are underlined; lowercase letters indicate sequences common in all pFA6a-MX6 plasmids.
Microscopy and image analysis.
Cell morphology and chromosomes were observed by staining with calcofluor white and 4′,6-diamidino-2-phenylindole (DAPI), respectively, and were imaged with an Olympus epifluorescence microscope equipped with a video micrometer (Olympus), Olympus UVFL 40× (numerical aperture, 1.3) and oil immersion objectives, and a charge-coupled-device (CCD) camera (Ikegami). For protein localization, the DeltaVision RT system (Applied Precision) consisting of an Olympus IX70 wide-field inverted fluorescence microscope, Olympus PlanApo 100× (numerical aperture, 1.4) and oil immersion objectives, and a CoolSnap HQ camera (Roper Scientific) was used. For CRIB-GFP (Cdc42/Rac interactive binding-green fluorescent protein) localization, cells grown at 27°C were transferred to 36°C for 3 h, subsequently mounted on a 2% agar pad, left at 36°C for another 30 min, and then observed under the microscope at 36°C. The cells were imaged in 15 Z sections, each 0.3 μm thick. The images were captured and processed by iterative constrained deconvolution using SoftWoRx (Applied Precision).
Screening for bipolar mutants under conditions of DNA replication arrest.
A panel of kinase-deleted mutants (Citation23) was crossed with pol1-1546, and each double mutant was constructed. The growth patterns of single-deletion mutants were previously reported (Citation24). Individual strains were then grown at 25°C until early log phase and shifted to 36°C. After a 4-h incubation at the restrictive temperature, the cells were fixed with formaldehyde and then stained with calcofluor white, after which the percentages of monopolar, bipolar, and septated cells were determined (n > 200). In total, 83 kinase gene deletions were analyzed (see Table S2 in the supplemental material).
Immunochemistry.
Preparation of cell extracts and immunoprecipitation were performed as follows. Cells (4 × 108) were collected by centrifugation and washed once with STOP buffer (150 mM NaCl, 50 mM NaF, 10 mM EDTA, and 1 mM NaN3). All subsequent manipulations were carried out at 4°C or on ice. Cells were resuspended in POM buffer (25 mM HEPES, pH 7.4, containing 0.1% Triton X-100, 10% glycerol, 50 mM potassium acetate, 50 mM NaF, 60 mM b-glycerophosphate, 2 mM EDTA, 1 mM dithiothreitol, 0.1 mM sodium vanadate, 15 mM p-nitrophenylphosphate, 40 μg ml−1 aprotinin, 20 μg ml−1 leupeptin, 1 μg ml−1 pepstatin, and 1 mM phenylmethylsulfonyl fluoride). They were then broken by vigorous vortexing with glass beads (GMB-40; Nippon Rikagaku Kikai) for 20 min, and the cell lysates were cleared by centrifugation at 7,000 rpm (4,400 × g) for 2 min. Protein concentrations were determined by using the Bradford protein assay system (Bio-Rad). The lysates (2 mg of protein) were pretreated with magnetizable beads conjugated to protein G (Dynabeads; Dynal) by incubation at 4°C with rotation for 60 min. The pretreated lysates were then separated from the beads and incubated with fresh protein G and a monoclonal anti-GFP or anti-FLAG antibody (1:200 dilution) at 4°C for 2 h. The beads were then washed in POM buffer, and cell extracts or immunocomplexes bound to them were separated by SDS-PAGE and analyzed by immunoblotting. For the detection of phosphorylated proteins, phosphate affinity SDS-PAGE was carried out by using Phos-tag acrylamide (NARD Institute). λ-Phosphatase (λ-PPase) (NEB) was used for phosphatase treatment. Cell extracts were incubated at 30°C for 1 h in the presence or absence of λ-phosphatase (400 units). The antibody probes used for immunoblotting were antihemagglutinin (anti-HA) (HA.11; Covance), anti-GFP (Roche), and anti-FLAG (M2; Sigma) antibodies. The band intensity was quantified with Image J.
In vitro assay of Cki3 protein kinase activity.
Protein extracts prepared from cells expressing Cki3-GFP or Cki3-HA were pretreated with magnetizable beads conjugated to protein G (Dynabeads; Dynal) by incubation at 4°C with rotation for 60 min. The pretreated extracts were separated from the beads with a micropipette and incubated with monoclonal anti-GFP (Roche) or anti-HA (Covance) antibodies for 1 h, followed by treatment with fresh protein G-conjugated beads at 4°C for 1.5 h with rotation. The beads were then washed three times with wash buffer (50 mM Tris-HCl, pH 7.4, containing 150 mM NaCl, 2 mM EDTA, and 0.1% Triton X-100) and once in kinase buffer (50 mM Tris-HCl, pH 7.4, containing 150 mM NaCl, 10 mM MgCl2, and 1 mM MnCl2). The precipitants (20 μl) were then incubated with 15 μl of kinase reaction buffer (kinase buffer plus 1 mM dithiothreitol, 5 μCi of [γ-32P]ATP, 1 μM ATP, 3 mg/ml casein) at 30°C for 20 min. The reaction was stopped by the addition of 15 μl of 2× SDS-PAGE sample buffer. Samples were then separated by SDS-PAGE.
RESULTS
The cki3 deletion mutant undergoes bipolar growth when DNA replication is incomplete.
Several protein kinases are known to be required for NETO execution in fission yeast (Citation7, Citation8, Citation24), so we reasoned that protein phosphorylation events might also be involved in NETO delay under conditions of DNA replication arrest. In order to identify such kinases, we performed systematic screening using a deletion library of protein kinase genes (Citation23). Each deletion strain was crossed with the temperature-sensitive pol1-1546 mutant, in which the S phase is arrested in monopolar cells (Citation11), and double mutants were constructed (83 strains). The growth polarity of each mutant was examined by calcofluor white staining (Citation6) in cultures incubated at 36°C for 4 h (see Fig. S1A and Table S2 in the supplemental material). Three mutants (cki3, gsk3, and ppk26) displayed a larger population of bipolar cells in the pol1-1546 background. Subsequent reevaluation by independent experiments showed that the cki3 mutant exhibited a consistent result (53.5% bipolar cells), whereas neither the gsk3 nor the ppk26 mutant displayed reproducibly higher bipolarity (20% and 27%, respectively) (see Fig. S1B in the supplemental material). Therefore, we selected Cki3 for further analysis. Cki3 belongs to the casein kinase superfamily (Citation13, Citation14, Citation25) and, in particular, is a member of the CK1γ family. The fission yeast genome contains two other CK1γ family members, Cki1 and Cki2 (Citation25). In order to examine the functional redundancy between Cki1 and Cki3. The pol1 cki1Δ cki3Δ triple mutant was constructed; however, these cells displayed the multiseptated phenotype at both 25°C and 36°C (see Fig. S1C in the supplemental material). In the case of Cki2, this subtype, as well as its budding yeast homolog, Yck3, is localized to the vacuole membrane and is likely to play a role distinct from those of Cki1-Cki3 and Yck1-Yck2 (Citation26, Citation27). Hence, subsequently, we focused our investigation on Cki3.
To assess growth polarity more precisely, we exploited the CRIB-GFP reporter, which selectively interacts with GTP-bound active Cdc42 and therefore marks growing tips (Citation28). Consistent with previous results (Citation11), CRIB-GFP was localized to the only one-cell tip in the pol1 mutant at the restrictive temperature (). In contrast, pol1 cki3Δ double-mutant cells exhibited mostly bipolar CRIB localization under the same conditions (). Interestingly, the bipolar-growth phenotype of the pol1 cki3Δ mutant was eliminated when genes coding for polarity factors, such as Tea1 and Tea4 (Citation10, Citation29), were deleted (see Fig. S2 in the supplemental material); either of these deletion mutants is known to undergo monopolar growth under unperturbed conditions. Furthermore, the cki3 deletion also could not induce bipolar growth in these mutants even at the permissive temperature. This indicates that in the absence of Cki3, Tea1 and Tea4 are required for bipolar growth not only upon an S-phase block but also during the cell cycle.
FIG 1 Cki3 is required for NETO inhibition when DNA replication is blocked. (A) Distribution of CRIB-GFP signals in pol1 and pol1 cki3Δ mutants. Cells were grown at 27°C, shifted up to 36°C, and kept at that temperature for 3.5 h. Representative patterns of CRIB-GFP (top) and the percentages of monopolar and bipolar cells at 27°C and 36°C (n > 100) (bottom) are shown. One cell undergoing monopolar growth is outlined (top right). (B) Increase in Cki3 kinase activity upon S-phase arrest. Cells expressing Cki3-GFP (the wild type [WT] and the pol1 mutant [lanes 2 and 3, respectively]) or nontagged wild-type cells (lane 1) were grown at 25°C and then transferred to 36°C and kept at that temperature for 1 h. Cki3-GFP was immunoprecipitated (IP) with an anti-GFP antibody (using 4 mg of protein extracts). Half of each precipitate was used for immunoblotting, while the other half was assayed for the phosphorylation reaction using casein as a substrate. The kinase activity (KA) was quantified with Image J (bottom). WCE, whole-cell extracts; RI, relative intensity.
![FIG 1 Cki3 is required for NETO inhibition when DNA replication is blocked. (A) Distribution of CRIB-GFP signals in pol1 and pol1 cki3Δ mutants. Cells were grown at 27°C, shifted up to 36°C, and kept at that temperature for 3.5 h. Representative patterns of CRIB-GFP (top) and the percentages of monopolar and bipolar cells at 27°C and 36°C (n > 100) (bottom) are shown. One cell undergoing monopolar growth is outlined (top right). (B) Increase in Cki3 kinase activity upon S-phase arrest. Cells expressing Cki3-GFP (the wild type [WT] and the pol1 mutant [lanes 2 and 3, respectively]) or nontagged wild-type cells (lane 1) were grown at 25°C and then transferred to 36°C and kept at that temperature for 1 h. Cki3-GFP was immunoprecipitated (IP) with an anti-GFP antibody (using 4 mg of protein extracts). Half of each precipitate was used for immunoblotting, while the other half was assayed for the phosphorylation reaction using casein as a substrate. The kinase activity (KA) was quantified with Image J (bottom). WCE, whole-cell extracts; RI, relative intensity.](/cms/asset/287c3e39-e149-4b25-a2df-30c0f7ba8e98/tmcb_a_12275595_f0001_oc.jpg)
Consistent with the role of Cki3 under a DNA replication block, an in vitro protein kinase assay using casein as a substrate showed that Cki3 kinase activity was substantially increased in the pol1 mutant incubated at 36°C (). Together, these results suggest that Cki3 acts as a critical regulator for growth polarity, thereby delaying NETO in the pol1 mutant.
Cki3 acts downstream of Cds1 and calcineurin.
We previously showed that the DNA replication checkpoint kinase Cds1 delays NETO onset when overproduced during G2 independently of an S-phase block, in which calcineurin acts downstream of Cds1 (Citation11). To examine whether Cki3 overproduction would also inhibit NETO, we overexpressed the cki3+ gene under the thiamine-repressible nmt1 promoter (Citation30) on episomal plasmids and used them to transform wild-type cells. As shown in , cki3+ overexpression induced accumulation of monopolar cells, like cds1+. Interestingly, the increase in monopolar patterns was still observed in the absence of Cds1, calcineurin (Ppb1), or Tip1, which acts downstream of calcineurin (Citation11).
FIG 2 Cki3 acts downstream of Cds1 and calcineurin when the DNA replication checkpoint is activated. (A) Growth patterns upon overexpression of various genes in individual deletion strains. Cells containing an empty vector (pREP1) or plasmid pREP1-cki3+, pREP1-cds1+, or pREP1-ppb1ΔC were cultured in the absence of thiamine (derepressed) at 28°C for 16 h, and growth polarity was examined by calcofluor white staining. The percentages of monopolar cells are shown. P values were obtained by chi-square tests. **, P < 0.01. (B) Percentages of monopolar, bipolar, and septated cells. Individual strains were grown at 25°C and shifted to 36°C for 4 h. The growth patterns were examined by calcofluor white staining (n > 200). (C) HU sensitivity. Tenfold serial dilutions of the indicated strains were spotted on Edinburgh minimal medium (EMM) plates containing appropriate supplements (leucine, uracil, histidine, adenine, and lysine) in the presence or absence of HU (5 and 7.5 mM) and incubated at 27°C for 4 days.
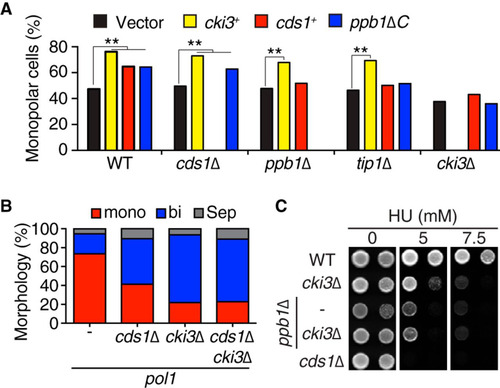
On the other hand, the growth patterns of the cki3 deletion mutant were insensitive to overexpression of Cds1 or a C-terminally truncated constitutively active form of calcineurin (Ppb1ΔC) (Citation11) (), indicating that these two proteins were upstream elements of Cki3. Consistently, the percentage of bipolar growth was not further increased in the pol1 cds1Δ cki3Δ triple mutant compared to each double mutant, pol1 cds1Δ or pol1 cki3Δ (). Interestingly, cki3Δ cells were hypersensitive to HU, like ppb1Δ cells, and in line with a linear relationship between the two proteins, the double mutant displayed no additive phenotypes in terms of HU sensitivity (). Collectively, our data suggest that Cki3 acts downstream of Cds1 and calcineurin.
Cki3, in concert with Tip1, is critical to retaining cell viability when DNA replication is blocked.
What is the biological significance of NETO inhibition while DNA replication is being halted? We found that pol1 cki3Δ double mutants could not form colonies at the semirestrictive temperature (34°C), while pol1 single-mutant cells could (). In contrast, pol1 ppb1Δ double mutants showed an even stronger impact, which was not exaggerated in the pol1 cki3Δ ppb1Δ triple mutant (). This result is consistent with the data for HU hypersensitivity presented above (see ), further substantiating the proposition that Cki3 acts downstream of Ppb1.
FIG 3 Cki3 acts in concert with Tip1 to retain cell viability under an S-phase block. (A) cki3Δ and tip1I87AI137A render the pol1 mutant more sensitive to high temperatures. Tenfold serial dilutions of the indicated strains were spotted on rich YE5S (yeast extract with 5 supplements) plates and incubated at various temperatures; the plates were incubated for 2 days. (B) Cell viability. (Left) The indicated strains were incubated at 36°C, and the viable colonies were counted by plating aliquots from cell cultures at each time point. (Right) Percentages of lethal “cut” cells (n > 200). (C) Functional hierarchy among individual factors required for NETO delay under conditions of perturbed DNA replication. Cki3 and Tip1 act downstream of Cds1 and calcineurin, but it is possible that the two proteins functionally interact (depicted as a two-headed arrow).
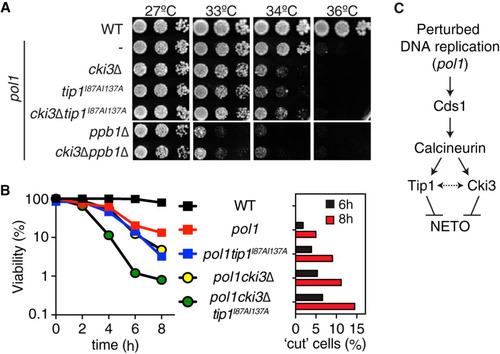
We then examined further the functional hierarchy between Cki3 and Tip1. The tip1 mutant allele (pol1 tip1I87AI137A), which fails to interact with calcineurin and as a result triggers NETO (Citation11), lowered the restrictive temperature of the pol1 mutant like pol1 cki3Δ (). Deletion of Cki3 in this strain (pol1 cki3Δ tip1I87AI137A) apparently caused behavior similar to that of each double mutant, pol1 tip1I87AI137A or pol1 cki3Δ (). Viability tests with liquid cultures showed that cell viability progressively decreased in pol1 cki3Δ double mutants incubated at 36°C, as in pol1 tip1I87AI137A double mutants (, left). Interestingly, pol1 cki3Δ tip1I87AI137A mutants displayed a sharper drop in cell viability than individual double mutants (). Consistent with the reduced viability, microscope observation indicated the emergence of lethal “cut” cells (septum formation across the undivided nucleus) within the population (, right) (Citation11, Citation31). Taking these results together, we envision that Cki3 and Tip1 acted in a parallel manner downstream of the Cds1-calcineurin pathway, though as-yet-unknown functional interactions between the two proteins may operate (). In summary, Cki3 is essential for maintaining monopolar growth when DNA replication is blocked and is also important for keeping cells alive under these conditions.
Autophosphorylation of Cki3 in its C-terminal region is inhibitory.
Previous reports indicated that CK1γ is autophosphorylated and that this phosphorylation negatively regulates CK1γ activity (Citation32). Accordingly, we next addressed whether Cki3 would also be regulated by autophosphorylation. By implementing a high-sensitivity phosphorylation detection system (Phos-tag) (Citation33), we showed that Cki3 is a highly phosphorylated protein with multiple more slowly migrating bands, which mostly disappeared upon treatment with λ-PPase (). In line with the notion of autophosphorylation, the slowly migrating phosphorylated bands were abolished in cells containing kinase-dead Cki3K44R ().
FIG 4 Cki3 is negatively regulated by autophosphorylation. (A) Phosphatase treatment of Cki3. Individual cells were grown at 25°C, and whole-cell extracts were prepared from cells expressing Cki3-HA and treated with λ-PPase in the presence or absence of the phosphatase inhibitor. Proteins were separated by SDS-PAGE in the presence (top) or absence (bottom) of 50 μM Phos-tag. Immunoblotting was performed with an anti-HA antibody. (B) The C terminus of Cki3 is autophosphorylated. Individual cells were grown at 25°C. Whole-cell extracts were prepared from strains containing the indicated Cki3 mutants tagged with HA, treated with λ-PPase, and separated by SDS-PAGE as for panel A. (C) Schematic presentation of the Cki3 structure and the amino acid sequence of the C-terminal region. The 4 amino acid residues shown in red (S and T) were mutated to either alanines (Cki34A) or glutamates (Cki34E). (D) Phosphomimetic Cki3 behaves like cki3 deletion. Cells containing CRIB-GFP were grown at 27°C, shifted up to 36°C, and kept at that temperature for 4 h. Growth polarity was examined by CRIB-GFP distribution (n > 100). Representative images (top) and quantification (bottom) are shown.
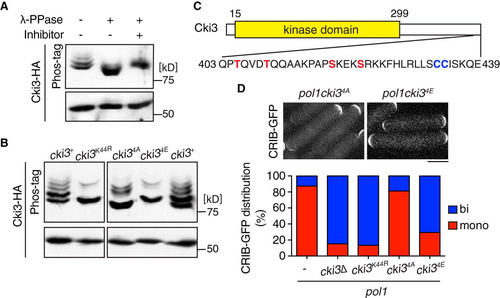
Genome-wide phosphoproteome analysis has indicated that S418, close to the C terminus of Cki3, is phosphorylated in vivo (Citation34). Intriguingly, this serine lies in the consensus phosphorylation sequence catalyzed by CK1, pS/TX1–3S/T, in which the first S/T is phosphorylated by another priming kinase (418SKEKS422; putative phosphosites are underlined) (Citation13). In addition, there is a second consensus phosphorylation site in this region (405TQVDT409) (). Therefore, we mutated these four residues to alanines (nonphosphorylatable) or glutamates (phosphomimetic) and designated the mutants Cki34A and Cki34E, respectively. Phos-tag analysis showed that the phosphorylated bands disappeared or decreased in density in cells containing Cki34A or Cki34E (). However, we noted that although the phosphorylated bands almost disappeared in Cki34E, as in Cki3K44R, some residual phosphorylated bands were still retained in Cki34A, suggesting that Cki34A or kinases other than Cki3 might have phosphorylated additional sites within Cki3 when the four residues were converted to the nonphosphorylatable form. Interestingly, while the growth polarity of Cki34A was indistinguishable from that of wild-type Cki3 in the pol1 mutant background, Cki34E behaved like the cki3 deletion; NETO was induced in the pol1 cki34E mutant (). It is worth pointing out that the protein kinase activity of Cki3 is necessary for monopolar growth, as a kinase-dead mutant of Cki3 (Cki3K44R) behaved similarly to the cki3 deletion mutant (), suggesting the existence of critical substrates for regulating growth polarity other than autophosphorylation. These results establish that Cki3 is indeed negatively regulated by its autophosphorylation at the C terminus.
Calcineurin is required for dephosphorylation of Cki3.
We then addressed how Cki3 is regulated by its upstream factor calcineurin. First, we examined the physical interaction between Cki3 and Ppb1. We found that the two proteins coimmunoprecipitated and that this interaction was not enhanced in the pol1 mutant incubated at 36°C (; see Fig. S3A and B in the supplemental material). Next, we examined Cki3 mobility by using the Phos-tag system and deletion mutants of the catalytic or regulatory subunit of calcineurin (Ppb1 and Cnb1, respectively) (Citation35, Citation36). In either mutant, we observed an increased density of phosphorylated bands (). This increase was specific to calcineurin, as the PP1 dis2Δ mutant (Citation37, Citation38) did not show this profile. In line with increased levels of inhibitory autophosphorylation in the absence of calcineurin, in vitro Cki3 kinase activity was reduced in ppb1Δ cells (). Taking the data together, we envisage that calcineurin may have directly dephosphorylated autophosphorylated Cki3, thereby activating Cki3 kinase activity.
FIG 5 Calcineurin is responsible for Cki3 dephosphorylation and promotes its kinase activity. (A) Calcineurin binds Cki3. Individual cells were grown at 27°C, and whole-cell extracts were prepared from cells containing Ppb1-FLAG and Cki3-GFP or Ppb1-FLAG or Cki3-GFP only. Immunoprecipitation was performed with an anti-GFP antibody, followed by immunoblotting with anti-FLAG and anti-GFP antibodies. (B) Calcineurin is responsible for dephosphorylation of Cki3. Immunoprecipitates prepared from the indicated strains containing Cki3-HA were immunoblotted with an anti-HA antibody in a Phos-tag (top) or normal (bottom) gel. As in , Cki3 was resolved into four major bands (1 to 4). Density scan patterns are shown on the right; black, wild type; gray, dis2Δ; orange, cnb1Δ; red, ppb1Δ. A.U., arbitrary units. (C) Cki3 kinase activity is reduced in the absence of calcineurin. The in vitro kinase activity of a ppb1Δ strain containing Cki3-GFP was measured as for by using casein as a substrate (far right). Strains containing nontagged Cki3, Cki3-GFP, or Cki3K44R-GFP were also used. The kinase activity (KA) was quantified with Image J (bottom).
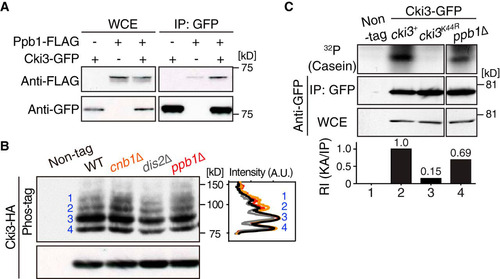
Erf2 palmitoyltransferase is essential for Cki3 localization to the plasma membrane.
One of the features of the CK1γ subgroup is its membrane-binding ability (Citation13, Citation14). Consistently, C-terminally fluorescence-tagged Cki3 produced under its own promoter (Cki3-GFP) localized to the whole plasma membrane, though regions corresponding to cell tips and the septum appeared more enriched in GFP signals (). This accumulation at the cell tips did not depend on either growth polarity or cell cycle stages (). It is known that plasma membrane localization of CK1γ is regulated by S-palmitoylation (Citation14, Citation20). Accordingly, we sought to identify the palmitoyltransferase that is responsible for the observed Cki3 localization. As recently reported (Citation39, Citation40), the fission yeast genome contains five open reading frames (ORFs) that encode proteins containing the DHHC (Asp-His-His-Cys) motif, which is characteristic of palmitoyltransferase (Citation18, Citation41) (). We checked the localization of Cki3-GFP in each deletion mutant except that of Swf1, which is essential for cell viability (). This analysis clearly showed that Erf2 was responsible for Cki3 plasma membrane localization; in erf2Δ cells, Cki3 was dispersed across the cytoplasm (). Erf2 requires the coactivator Erf4 for its full function (Citation39, Citation40), and indeed, Cki3-GFP was also delocalized from the plasma membrane in the erf4Δ mutant (D. Hirata and T. Koyano, unpublished observation). Cki3 localization was not distinctively altered under Cds1 overproduction (see Fig. S4 in the supplemental material), suggesting that factors other than the cellular localization potentiate Cki3 kinase activity when DNA replication is activated (see ). Collectively, these results established that Erf2, together with Erf4, is a palmitoyltransferase responsible for Cki3 localization to the plasma membrane.
FIG 6 Erf2-mediated palmitoylation is essential for plasma membrane localization of Cki3. (A) Cki3 localization in wild-type cells. Cells exponentially growing at 27°C that produced Cki3-GFP and Crn1-tdTomato (coronin, an actin-binding protein) (Citation53) were mounted on 2% agar pads containing minimal medium. Representative images corresponding to various cell cycle stages are shown. Maximum-intensity projection images are presented. Bar, 5 μm. (B) Summary of putative palmitoyltransferases and Cki3 localization in their deletion mutants. N.D., not determined; PM, plasma membrane. (C) Cki3 localization in erf2Δ cells. Images corresponding to a single section in the middle (left) and maximum-intensity projections (right) are shown for wild-type (WT) and erf2Δ cells. Bar, 5 μm.
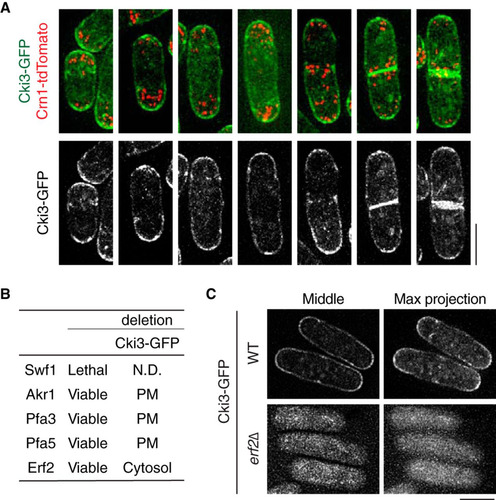
Two C-terminal cysteines are required for both membrane localization and kinase activity of Cki3.
Consistent with Erf2-dependent palmitoylation, Cki3 contains two cysteines in its C-terminal region (). To confirm the role of palmitoylation, we mutated these cysteines to serines (Cki3SS). As shown in , Cki3SS-GFP was no longer localized to the plasma membrane, just as in erf2Δ cells (see ). Intriguingly, the in vitro kinase activity of Cki3SS using casein was also substantially decreased, though compared with that of kinase-dead Cki3 (Cki3K44R), residual activity was still retained (14% versus 4%) (). The kinase activity of Cki3 was not required for its membrane localization, as the kinase-dead Cki3 mutant (Cki3K44R) was capable of being localized to the plasma membrane ( and ).
FIG 7 The C-terminal Cys-Cys sequences of Cki3 are required for not only membrane localization but also NETO delay. (A) C-terminal amino acid sequences of various CK1γ family members: S. pombe (S.p), Saccharomyces cerevisiae (S.c), C. elegans (C.e), Xenopus laevis (X.l), Drosophila melanogaster (D.m), and Homo sapiens (H.s). (B) Localization of Cki3 mutants defective in palmitoylation (Cki3SS) and kinase activity (Cki3K44R). Two cysteines were replaced with serines in Cki3SS, whereas K44, a crucial lysine residue for protein kinase activity (Citation25), was replaced with arginine in Cki3K44R. The top row shows maximum-intensity projection images, while the bottom row displays single-section (middle) images. Bar, 10 μm. (C) Reduced in vitro kinase activity in palmitoylation-deficient Cki3SS. Cell extracts (4 mg) were prepared from the indicated strains exponentially growing at 25°C, and immunoprecipitation was performed with anti-HA antibodies. Half of each precipitate was immunoblotted with an anti-GFP antibody, while the other half was assayed for the phosphorylation reaction using casein as a substrate. The kinase activity (KA) was quantified with Image J (bottom). (D) CRIB-GFP distribution. The indicated strains were grown at 27°C, shifted up to 36°C, and kept at that temperature for 3.5 h. Quantification of CRIB-GFP localization patterns is shown at the bottom (n > 100). Bar, 10 μm.
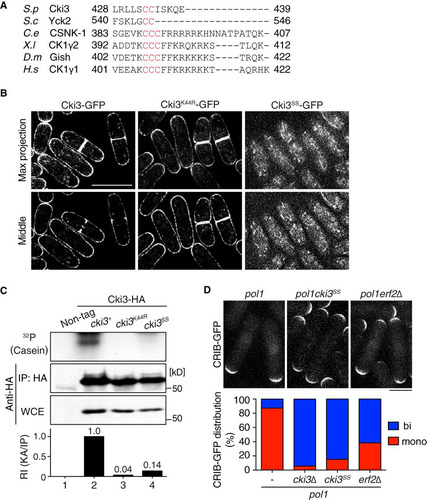
We next investigated the impact of palmitoylation-mediated Cki3 plasma membrane localization on NETO delay. Cells containing Cki3SS essentially behaved like cells with cki3 deleted on the pol1 mutant background; the pol1 cki3SS cells underwent bipolar growth at the restrictive temperature (). In addition, the percentage of monopolar cells in the absence of Erf2 was substantially reduced. These data indicate that C-terminal palmitoylation is required not only for membrane localization but also for in vivo kinase activity of Cki3, which plays an inhibitory role in the execution of NETO under the DNA replication block.
DISCUSSION
How cell polarity changes in response to intracellular and/or extracellular cues is one of the critical questions in a variety of biological systems. In this study, we show that Cki3, the fission yeast homolog of CK1γ, plays a crucial role in delaying the growth polarity transition when DNA replication is blocked (). It was previously shown that calcineurin (Ppb1) acts directly downstream of Cds1 for NETO delay. The phosphatase dephosphorylates Tip1/CLIP170, thereby restraining NETO via modulating microtubule dynamics (Citation11). We show that Tip1 and Cki3 act in a parallel rather than a linear manner downstream of Cds1 and calcineurin ( and ). Cki3 is autophosphorylated and dephosphorylated by calcineurin, leading to Cki3 activation ( and ). We have also shown that Cki3 is localized to the plasma membrane, and this localization was mediated by the Erf2-Erf4 palmitoyltransferase complex (). Membrane localization of Cki3 is essential for its kinase activity and NETO delay; however, Cki3 kinase activity is not required for membrane localization (). Therefore, it appears that the Cds1-calcineurin pathway bifurcates, forming two branches, i.e., the Tip1-microtubule branch and the Cki3-membrane branch (depicted in ).
FIG 8 Schematic model of NETO controlled by Cki3. Upon a DNA replication block, the Cds1/Chk2 checkpoint kinase is activated, resulting in two inhibitory outcomes: one is cell cycle arrest, and the other is inhibition of NETO (transition from monopolar to bipolar growth). It was previously shown that calcineurin (Ppb1) acts downstream of Cds1, thereby dephosphorylating Tip1/CLIP170 and in turn restraining NETO (Citation11). In the current study, we show that, in addition to Tip1, membrane-bound Cki3 is also dephosphorylated, mediated by calcineurin. Cki3 is autophosphorylated, which inactivates Cki3 kinase activity. Therefore, calcineurin activates Cki3. Note that Cki3 and Tip1 may functionally interact, instead of acting independently. The plasma membrane localization of Cki3 is achieved by the Erf2-Erf4 palmitoyltransferase, which is also essential for Cki3 kinase activity. Dephosphorylated Cki3 inhibits NETO, the molecular mechanism of which is currently unknown. It is possible that membrane-bound substrate proteins required for NETO are inactivated through Cki3-mediated phosphorylation. Alternatively, substrate proteins that are inhibitory for NETO onset are activated instead.
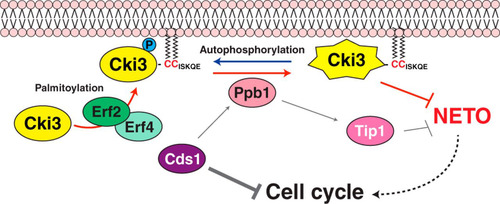
Plasma membrane localization of Cki3 and asymmetric monopolar growth.
While Cki3 ensures asymmetrical growth patterns under a DNA replication block, it becomes localized symmetrically to both cell tips, in addition to the cell cortex. One possibility to account for this apparent paradox is that Cki3 may be activated in an asymmetric manner; activation and inactivation occur only at the nongrowing and growing ends, respectively. We previously showed that microtubule dynamics are asymmetrically regulated at the two cell tips; microtubules reaching the nongrowing end are more stable than those at the growing end. Furthermore, calcineurin is localized along the microtubule cytoskeleton when DNA replication is defective (Citation11). It is therefore tempting to speculate that calcineurin is delivered to the nongrowing end via the microtubule, thereby promoting dephosphorylation of Cki3, leading to monopolar patterns by inhibiting growth from that end. A phosphospecific antibody against Cki3 autophosphorylation sites would be a useful tool with which to scrutinize this possibility.
The roles of palmitoylation in Cki3 functions.
Like other posttranslational modifications, such as phosphorylation and acetylation, the palmitoylation reaction is also reversible (Citation42), suggesting that this is another step by which Cki3 functions are regulated. Humans have 23 or 24 DHHC-containing proteins (Citation42, Citation43), and global proteomic analyses suggest that a plethora of proteins are palmitoylated in vivo (Citation41, Citation44, Citation45). In budding and fission yeasts, the Erf2-Erf4 complex catalyzes modification of Ras and Rho family proteins (Citation39, Citation40, Citation43, Citation44, Citation46, Citation47). Interestingly, however, in budding yeast, it is the Akr1 palmitoyltransferase, not Erf2-Erf4, that is responsible for Yck2 palmitoylation (Citation17, Citation44). It is worth noting that only Yck2 contains double cysteines at its extreme C terminus, whereas other CK1γ family members, including the fission yeast Cki3, contain amino acid extensions rich in basic amino acids after the double cysteines (). These diversifications may contribute to the substrate specificity of Erf2-Erf4 and Akr1.
We have shown that palmitoylation-deficient Cki3 (Cki3SS) not only becomes delocalized from the plasma membrane but also loses its kinase activity (). A similar phenotype was also reported for the analogous Yck2 mutant (Citation19, Citation20). This implies that palmitoylation of Cki3 comprises a regulatory mechanism by which cytoplasmic Cki3 remains inactive. We surmise that the palmitoyl moiety is somehow involved in structural hindrance of the C-terminal autophosphorylation, which is reversed by calcineurin-mediated dephosphorylation, thus leading to Cki3 activation.
The inhibitory role of Cki3 in NETO onset.
Although Tip1 plays a negative role in NETO only when DNA replication is blocked (Citation11), we envision that Cki3 could regulate NETO even under unperturbed conditions. This proposition arises because pol1 cki3Δ cells, unlike pol1 tip1Δ cells, exhibit mostly bipolar growth even when they divide at the permissive temperature (), and furthermore, calcineurin-mediated dephosphorylation of Cki3 takes place during the normal cell cycle (). Cki3 is likely to be a key molecule in the signaling pathway regulating NETO under both unperturbed and perturbed conditions. A number of molecules required for NETO onset become localized to cell tips or the plasma membrane, and some of these factors, such as Tea1 and Tea4, are known to be phosphorylated (Citation7, Citation8, Citation48).
The biological significance of NETO remains elusive. However, deletion of cki3 renders cells hypersensitive to hydroxyurea on plates and the pol1 cki3Δ double mutant rapidly loses viability in liquid cultures ( and , respectively), indicating that Cki3 makes an important contribution to the high viability of fission yeast cells under an S-phase block. In human cells, when DNA replication is blocked, CK1γ phosphorylates claspin, which in turn activates Chk1 (Citation49). Considering the role of CK1γ during S phase, the timing of NETO might be closely coupled to the completion of S phase, as previously pointed out (Citation6, Citation11). This raises the intriguing possibility that cell cycle progression regulates not only chromosome behavior (in S and M phases) but also the cell polarity transition (in mid-G2 phase), both of which are under strict Cdc2/Cdk1-dependent control of cell size (Citation50). Further analysis will help address this proposition.
CK1γ in asymmetric cell polarity.
One of the conserved functions among CK1γ family members lies in the creation of asymmetry in the cell. For instance, during Caenorhabditis elegans embryogenesis, CSNK-1 is localized to the plasma membrane and accumulates at the anterior aspect of the embryo, where it excludes the PtdIns(4,5)P2 synthesis enzyme PPK-1. This biased localization of PPK-1 leads to posterior spindle displacement and subsequent asymmetrical cell division (Citation16). In budding yeast, Yck2 accumulates in the polarized region and plays essential roles in septin organization and morphogenesis (Citation51, Citation52). The results obtained from our present study are consistent with the idea that CK1γ is a critical regulator of asymmetric properties of the cell.
tmcb_a_12275595_sm0001.pdf
Download PDF (2.1 MB)ACKNOWLEDGMENTS
We thank Mohan Balasubramanian for his generous gift of the set of kinase deletions and Eiji Kinoshita for Phos-tag.
T.K. was the recipient of a JSPS fellowship (DC1; PD) and was partly supported by the Strategic Young Researcher Overseas Visits Program for Accelerating Brain Circulation from JSPS. This work was supported by Cancer Research UK (T.T.) and the Ministry of Education, Culture, Sports, Science and Technology (D.H. and K.K.).
The experiments were designed by T.K., K.K., D.H., and T.T. T.K. performed the majority of the experiments and data analysis. K.K. and M.K. performed mutant screening. S.G.M. and Y.O. provided materials and made critical suggestions during the course of this work. T.K. and T.T. wrote the paper with suggestions from the other authors.
Supplemental material for this article may be found at http://dx.doi.org/10.1128/MCB.01465-14.
REFERENCES
- Etienne-Manneville S. 2013. Microtubules in cell migration. Annu Rev Cell Dev Biol 29:471–499. http://dx.doi.org/10.1146/annurev-cellbio-101011-155711.
- Lancaster OM, Baum B. 2014. Shaping up to divide: coordinating actin and microtubule cytoskeletal remodelling during mitosis. Semin Cell Dev Biol 34:109–115. http://dx.doi.org/10.1016/j.semcdb.2014.02.015.
- Gonzalez DM, Medici D. 2014. Signaling mechanisms of the epithelial-mesenchymal transition. Sci Signal 7:re8. http://dx.doi.org/10.1126/scisignal.2005189.
- Hayles J, Nurse P. 2001. A journey into space. Nat Rev Mol Cell Biol 2:647–656. http://dx.doi.org/10.1038/35089520.
- Nurse P. 1990. Universal control mechanism regulating onset of M-phase. Nature 344:503–508. http://dx.doi.org/10.1038/344503a0.
- Mitchison JM, Nurse P. 1985. Growth in cell length in the fission yeast Schizosaccharomyces pombe. J Cell Sci 75:357–376.
- Huisman SM, Brunner D. 2011. Cell polarity in fission yeast: a matter of confining, positioning, and switching growth zones. Semin Cell Dev Biol 22:799–805. http://dx.doi.org/10.1016/j.semcdb.2011.07.013.
- Martin SG, Chang F. 2005. New end take off: regulating cell polarity during the fission yeast cell cycle. Cell Cycle 4:1046–1049. http://dx.doi.org/10.4161/cc.4.8.1853.
- Rupes I, Jia Z, Young PG. 1999. SspI promotes actin depolymerization and is involved in stress response and new end take-off control in fission yeast. Mol Biol Cell 10:1495–1510. http://dx.doi.org/10.1091/mbc.10.5.1495.
- Martin SG, McDonald WH, Yates JR, III, Chang F. 2005. Tea4p links microtubule plus ends with the formin for3p in the establishment of cell polarity. Dev Cell 8:479–491. http://dx.doi.org/10.1016/j.devcel.2005.02.008.
- Kume K, Koyano T, Kanai M, Toda T, Hirata D. 2011. Calcineurin ensures a link between the DNA replication checkpoint and microtubule-dependent polarized growth. Nat Cell Biol 13:234–242. http://dx.doi.org/10.1038/ncb2166.
- Brunner D, Nurse P. 2000. CLIP170-like tip1p spatially organizes microtubular dynamics in fission yeast. Cell 102:695–704. http://dx.doi.org/10.1016/S0092-8674(00)00091-X.
- Knippschild U, Gocht A, Wolff S, Huber N, Lohler J, Stoter M. 2005. The casein kinase 1 family: participation in multiple cellular processes in eukaryotes. Cell Signal 17:675–689. http://dx.doi.org/10.1016/j.cellsig.2004.12.011.
- Knippschild U, Kruger M, Richter J, Xu P, Garcia-Reyes B, Peifer C, Halekotte J, Bakulev V, Bischof J. 2014. The CK1 Family: contribution to cellular stress response and its role in carcinogenesis. Front Oncol 4:96. http://dx.doi.org/10.3389/fonc.2014.00096.
- Davidson G, Wu W, Shen J, Bilic J, Fenger U, Stannek P, Glinka A, Niehrs C. 2005. Casein kinase 1 gamma couples Wnt receptor activation to cytoplasmic signal transduction. Nature 438:867–872. http://dx.doi.org/10.1038/nature04170.
- Panbianco C, Weinkove D, Zanin E, Jones D, Divecha N, Gotta M, Ahringer J. 2008. A casein kinase 1 and PAR proteins regulate asymmetry of a PIP(2) synthesis enzyme for asymmetric spindle positioning. Dev Cell 15:198–208. http://dx.doi.org/10.1016/j.devcel.2008.06.002.
- Babu P, Deschenes RJ, Robinson LC. 2004. Akr1p-dependent palmitoylation of Yck2p yeast casein kinase 1 is necessary and sufficient for plasma membrane targeting. J Biol Chem 279:27138–27147. http://dx.doi.org/10.1074/jbc.M403071200.
- Roth AF, Feng Y, Chen L, Davis NG. 2002. The yeast DHHC cysteine-rich domain protein Akr1p is a palmitoyl transferase. J Cell Biol 159:23–28. http://dx.doi.org/10.1083/jcb.200206120.
- Roth AF, Papanayotou I, Davis NG. 2011. The yeast kinase Yck2 has a tripartite palmitoylation signal. Mol Biol Cell 22:2702–2715. http://dx.doi.org/10.1091/mbc.E11-02-0115.
- Babu P, Bryan JD, Panek HR, Jordan SL, Forbrich BM, Kelley SC, Colvin RT, Robinson LC. 2002. Plasma membrane localization of the Yck2p yeast casein kinase 1 isoform requires the C-terminal extension and secretory pathway function. J Cell Sci 115:4957–4968. http://dx.doi.org/10.1242/jcs.00203.
- Moreno S, Klar A, Nurse P. 1991. Molecular genetic analysis of fission yeast Schizosaccharomyces pombe. Methods Enzymol 194:795–823. http://dx.doi.org/10.1016/0076-6879(91)94059-L.
- Bahler J, Wu JQ, Longtine MS, Shah NG, McKenzie A, III, Steever AB, Wach A, Philippsen P, Pringle JR. 1998. Heterologous modules for efficient and versatile PCR-based gene targeting in Schizosaccharomyces pombe. Yeast 14:943–951.
- Bimbo A, Jia Y, Poh SL, Karuturi RK, den Elzen N, Peng X, Zheng L, O'Connell M, Liu ET, Balasubramanian MK, Liu J. 2005. Systematic deletion analysis of fission yeast protein kinases. Eukaryot Cell 4:799–813. http://dx.doi.org/10.1128/EC.4.4.799-813.2005.
- Koyano T, Kume K, Konishi M, Toda T, Hirata D. 2010. Search for kinases related to transition of growth polarity in fission yeast. Biosci Biotechnol Biochem 74:1129–1133. http://dx.doi.org/10.1271/bbb.100223.
- Kitamura K, Yamashita I. 1998. Identification of a novel casein kinase-1 homolog in fission yeast Schizosaccharomyces pombe. Gene 214:131–137. http://dx.doi.org/10.1016/S0378-1119(98)00203-0.
- Matsuyama A, Arai R, Yashiroda Y, Shirai A, Kamata A, Sekido S, Kobayashi Y, Hashimoto A, Hamamoto M, Hiraoka Y, Horinouchi S, Yoshida M. 2006. ORFeome cloning and global analysis of protein localization in the fission yeast Schizosaccharomyces pombe. Nat Biotechnol 24:841–847. http://dx.doi.org/10.1038/nbt1222.
- Sun B, Chen L, Cao W, Roth AF, Davis NG. 2004. The yeast casein kinase Yck3p is palmitoylated, then sorted to the vacuolar membrane with AP-3-dependent recognition of a YXXPhi adaptin sorting signal. Mol Biol Cell 15:1397–1406. http://dx.doi.org/10.1091/mbc.E03-09-0682.
- Tatebe H, Nakano K, Maximo R, Shiozaki K. 2008. Pom1 DYRK regulates localization of the Rga4 GAP to ensure bipolar activation of Cdc42 in fission yeast. Curr Biol 18:322–330. http://dx.doi.org/10.1016/j.cub.2008.02.005.
- Mata J, Nurse P. 1997. tea1 and the microtubular cytoskeleton are important for generating global spatial order within the fission yeast cell. Cell 89:939–949. http://dx.doi.org/10.1016/S0092-8674(00)80279-2.
- Maundrell K. 1990. nmt1 of fission yeast. A highly transcribed gene completely repressed by thiamine. J Biol Chem 265:10857–10864.
- Hirano T, Funahashi S, Uemura T, Yanagida M. 1986. Isolation and characterization of Schizosaccharomyces pombe cut mutants that block nuclear division but not cytokinesis. EMBO J 5:2973–2979.
- Zhai L, Graves PR, Robinson LC, Italiano M, Culbertson MR, Rowles J, Cobb MH, DePaoli-Roach AA, Roach PJ. 1995. Casein kinase I gamma subfamily. Molecular cloning, expression, and characterization of three mammalian isoforms and complementation of defects in the Saccharomyces cerevisiae YCK genes. J Biol Chem 270:12717–12724.
- Kinoshita E, Kinoshita-Kikuta E, Takiyama K, Koike T. 2006. Phosphate-binding tag, a new tool to visualize phosphorylated proteins. Mol Cell Proteomics 5:749–757. http://dx.doi.org/10.1074/mcp.T500024-MCP200.
- Wilson-Grady JT, Villen J, Gygi SP. 2008. Phosphoproteome analysis of fission yeast. J Proteome Res 7:1088–1097. http://dx.doi.org/10.1021/pr7006335.
- Yoshida T, Toda T, Yanagida M. 1994. A calcineurin-like gene ppb1+ in fission yeast: mutant defects in cytokinesis, cell polarity, mating and spindle pole body positioning. J Cell Sci 107:1725–1735.
- Sio SO, Suehiro T, Sugiura R, Takeuchi M, Mukai H, Kuno T. 2005. The role of the regulatory subunit of fission yeast calcineurin for in vivo activity and its relevance to FK506 sensitivity. J Biol Chem 280:12231–12238. http://dx.doi.org/10.1074/jbc.M414234200.
- Ohkura H, Kinoshita N, Miyatani S, Toda T, Yanagida M. 1989. The fission yeast dis2+ gene required for chromosome disjoining encodes one of two putative type 1 protein phosphatases. Cell 57:997–1007. http://dx.doi.org/10.1016/0092-8674(89)90338-3.
- Alvarez-Tabares I, Grallert A, Ortiz JM, Hagan IM. 2007. Schizosaccharomyces pombe protein phosphatase 1 in mitosis, endocytosis and a partnership with Wsh3/Tea4 to control polarised growth. J Cell Sci 120:3589–3601. http://dx.doi.org/10.1242/jcs.007567.
- Zhang MM, Wu PY, Kelly FD, Nurse P, Hang HC. 2013. Quantitative control of protein s-palmitoylation regulates meiotic entry in fission yeast. PLoS Biol 11:e1001597. http://dx.doi.org/10.1371/journal.pbio.1001597.
- Sanchez-Mir L, Franco A, Martin-Garcia R, Madrid M, Vicente-Soler J, Soto T, Gacto M, Perez P, Cansado J. 2014. Rho2 palmitoylation is required for plasma membrane localization and proper signaling to the fission yeast cell integrity MAPK pathway. Mol Cell Biol 34:2745–2759. http://dx.doi.org/10.1128/MCB.01515-13.
- Greaves J, Chamberlain LH. 2011. DHHC palmitoyl transferases: substrate interactions and (patho)physiology. Trends Biochem Sci 36:245–253. http://dx.doi.org/10.1016/j.tibs.2011.01.003.
- Fukata Y, Fukata M. 2010. Protein palmitoylation in neuronal development and synaptic plasticity. Nat Rev Neurosci 11:161–175. http://dx.doi.org/10.1038/nrn2788.
- Young E, Zheng ZY, Wilkins AD, Jeong HT, Li M, Lichtarge O, Chang EC. 2014. Regulation of Ras localization and cell transformation by evolutionarily conserved palmitoyltransferases. Mol Cell Biol 34:374–385. http://dx.doi.org/10.1128/MCB.01248-13.
- Roth AF, Wan J, Bailey AO, Sun B, Kuchar JA, Green WN, Phinney BS, Yates JR, III, Davis NG. 2006. Global analysis of protein palmitoylation in yeast. Cell 125:1003–1013. http://dx.doi.org/10.1016/j.cell.2006.03.042.
- Kang R, Wan J, Arstikaitis P, Takahashi H, Huang K, Bailey AO, Thompson JX, Roth AF, Drisdel RC, Mastro R, Green WN, Yates JR, III, Davis NG, El-Husseini A. 2008. Neural palmitoyl-proteomics reveals dynamic synaptic palmitoylation. Nature 456:904–909. http://dx.doi.org/10.1038/nature07605.
- Zhao L, Lobo S, Dong X, Ault AD, Deschenes RJ. 2002. Erf4p and Erf2p form an endoplasmic reticulum-associated complex involved in the plasma membrane localization of yeast Ras proteins. J Biol Chem 277:49352–49359. http://dx.doi.org/10.1074/jbc.M209760200.
- Bartels DJ, Mitchell DA, Dong X, Deschenes RJ. 1999. Erf2, a novel gene product that affects the localization and palmitoylation of Ras2 in Saccharomyces cerevisiae. Mol Cell Biol 19:6775–6787.
- Martin SG. 2009. Microtubule-dependent cell morphogenesis in the fission yeast. Trends Cell Biol 19:447–454. http://dx.doi.org/10.1016/j.tcb.2009.06.003.
- Meng Z, Capalbo L, Glover DM, Dunphy WG. 2011. Role for casein kinase 1 in the phosphorylation of Claspin on critical residues necessary for the activation of Chk1. Mol Biol Cell 22:2834–2847. http://dx.doi.org/10.1091/mbc.E11-01-0048.
- Grallert A, Patel A, Tallada VA, Chan KY, Bagley S, Krapp A, Simanis V, Hagan IM. 2013. Centrosomal MPF triggers the mitotic and morphogenetic switches of fission yeast. Nat Cell Biol 15:88–95. http://dx.doi.org/10.1038/ncb2633.
- Robinson LC, Menold MM, Garrett S, Culbertson MR. 1993. Casein kinase I-like protein kinases encoded by YCK1 and YCK2 are required for yeast morphogenesis. Mol Cell Biol 13:2870–2881.
- Robinson LC, Bradley C, Bryan JD, Jerome A, Kweon Y, Panek HR. 1999. The Yck2 yeast casein kinase 1 isoform shows cell cycle-specific localization to sites of polarized growth and is required for proper septin organization. Mol Biol Cell 10:1077–1092. http://dx.doi.org/10.1091/mbc.10.4.1077.
- Pelham RJ, Jr, Chang F. 2001. Role of actin polymerization and actin cables in actin-patch movement in Schizosaccharomyces pombe. Nat Cell Biol 3:235–244. http://dx.doi.org/10.1038/35060020.