Abstract
Laser cleaning of bronze artefacts is a very promising conservation approach and its wider distribution would benefit from further studies of its advantages and drawbacks. Some important references are available, yet laser cleaning cannot be considered a traditional method for bronze surfaces. In this paper, we report on the evaluation of cleaning tests performed on an important statue of Napoleon, depicted as Mars the Peacemaker, cast by Righetti in 1811 after a model by Antonio Canova. The sculpture is exposed to the outdoor environment in the main courtyard of the Brera Gallery, Milan, Italy. An analytical survey of corrosion products was carried out using X-ray diffraction, micro-Fourier transform infrared spectroscopy (μFTIR), and μRaman spectroscopy; and scanning electron microscopy with energy-dispersive X-ray spectroscopy analysis was applied to cross-sections. The results showed the ubiquitous presence of brochantite Cu4(SO4)(OH)6. Antlerite Cu3SO4(OH)4 is also occasionally present. Residues of an past conservation treatment (Incralac®) and of proteins were detected by μFTIR. Laser cleaning tests were carried out with an El.En. Combo laser, both in long Q-switched and short free-running regimes. Evaluation of the effects of cleaning was carried out with a portable microscope and a reflectance colorimeter. Moreover, a portable FTIR spectrometer was used in order to evaluate the presence of coating residues and corrosion products after cleaning. Electrochemical impedance spectroscopy was used in situ on a selection of the tested areas in order to characterize the effect of laser cleaning on the rate of corrosion. A specially designed contact probe was used for measurements in the frequency range 100 kHz–10 mHz both before and after cleaning.
Introduction: cleaning a bronze sculpture
Approaches to cleaning bronze surfaces vary greatly due to differing aesthetic decisions, available resources, and the degree of understanding of the relative stability of surface and corrosion patinas. The most common method of cleaning weathered bronze may entail washing with aqueous solutions, usually followed by scrubbing with bristle brushes to remove loose corrosion and degreasing by an organic solvent to remove embedded waxes and other organic coatings.
Laser cleaning of metals, which is a valid alternative to traditional methods, is associated mainly with the use of Nd:YAG lasers emitting at their fundamental frequency (1064 nm) with optimized pulse durations either in long Q-switch (LQS) or short free-running (SFR) regimes (Siano & Salimbeni, Citation2010). The SFR regime consisting of the emission of 20 μs pulses proved efficient in removal of calcareous and siliceous encrustation (Pini et al., Citation2000), while that emitting in 40–150 μs range allowed for a very gradual removal of black crusts and oleo-resinous surface coatings rich in silicates, calcite, gypsum, carbon black, and ochres, along with calcium and copper oxalates, from both gilded bronze and bronze surfaces (Siano et al., Citation2012). In fact, several authors stress the importance of the choice of suitable pulse duration and irradiation conditions for the cleaning of metal surfaces, where temperature increases on the surface are of critical concern. This generally increases with decreasing pulse duration but it lasts for a longer time with longer pulse durations. The optimized pulse duration can prevent high mechanical stress and at the same time minimize large instantaneous temperature gradients in the irradiated surfaces. (Garbacz et al., Citation2010; Siano & Salimbeni, Citation2010). Moreover, the application of water during laser cleaning leads not only to local cooling of the irradiated area but also to the reduction of the fraction of reflected laser light (Pini et al., Citation2000), and is often of great help in optimizing cleaning. Consequently, increased laser ablation efficiency can be achieved by more efficient coupling of laser radiation and the encrustation layer. Ultraviolet laser wavelengths have also been tested (Pini et al., Citation2000; Buccolieri et al., Citation2013) but their main disadvantage is their extremely low ablation efficiency. UV lasers can therefore be considered of relatively low practical interest.
Different techniques are employed for the evaluation of laser cleaning of metals. One of the most frequently reported is optical and scanning electron microscopy (SEM) with energy-dispersive X-ray spectroscopy (EDX) that allows for characterization of the surface morphology and for determination of elemental composition (Korenberg & Baldwin, Citation2006; Garbacz et al., Citation2010; Garbacz et al., Citation2011; Lee et al., Citation2013), atomic force microscopy (Lee et al., Citation2013), X-ray photo-electron spectroscopy (Lee et al., Citation2013), and ion chromatography (Matteini et al., Citation2003). Laboratory characterization may be of great importance for systematic fundamental investigation or when objects of small dimensions are cleaned. However, there is significant need for portable instrumentation for the evaluation of the cleaning progress on historical monuments where sampling is limited. Such non-invasive evaluation can be based on elemental analysis by energy-dispersive X-ray fluorescence, EDXRF (Korenberg & Baldwin, Citation2006; Buccolieri et al., Citation2013), Raman spectroscopy (Garbacz et al., Citation2011; Siano et al., Citation2012), and laser-induced plasma or breakdown spectroscopy (Siano et al., Citation2012).
The procedure presented in this paper makes use of a multi-analytical protocol, based on the use of portable instruments. Portable FTIR spectrometry and reflectance colorimetry have been compared with optical microscopy. These techniques proved to be useful to address most of the problems concerning cleaning evaluation. Many are discussed in the literature, even if a bench FTIR instrument is usually used to provide transmission or absorption spectra. The spectra in our study were acquired in reflectance mode. Moreover, as an original contribution we report, for the first time, on the use of electrochemical impedance spectroscopy (EIS) used during laser cleaning of a bronze sculpture. EIS measurements represent powerful analytical tools for metal characterization and they are becoming more and more frequently used in the analysis of metal objects in conservation. Laboratory measurements have been more widely applied, while very few in situ measurements have been reported (Letardi, Citation2013). A special contact probe has been developed for EIS measurements on a bronze statue (Letardi, Citation2004) and for the first time we used this technique to characterize the effect of laser cleaning on the rate of corrosion.
The important figure of Napoleon, depicted as Mars the Peacemaker (), was cast by Righetti in 1809 after a model by Antonio Canova. The sculpture is exposed to the outdoor environment in the main courtyard of the Brera Gallery, Milan, Italy. An analytical survey of the corrosion products was carried out by means of XRD, μFTIR, and μRaman; while SEM-EDX was applied to cross-sections. The XRD, μFTIR, and μRaman results showed the ubiquitous presence of brochantite Cu4(SO4)(OH)6. Antlerite Cu3SO4(OH)4 is also present, but on some areas only. Optical microscopy observations and SEM-EDX applied to cross-sections highlighted the condition of the alloy/patina interface and rendered visible the direct contact between blue/green corrosion product and the alloy; black encrustations were locally present before cleaning in areas sheltered from rain wash; their thickness was in the range 50–200 μm and they were mainly composed of silicates and carbon particles. Residues of an applied acrylic coating (Incralac®) coupled with microcrystalline waxes, and of proteins were highlighted by μFTIR.
Figure 1. Detail of the head of the bronze sculpture of Napoleon. Different cleaning problems are visible in the cheek (grayish/greenish in colour as Area 1), in the neck under the chin (black and shiny as Area 2) and in the shoulder (brilliant green colour as Area 3).
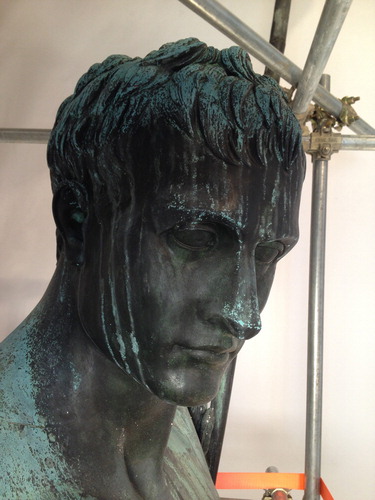
Three areas were chosen for cleaning tests, representing three different cleaning problems. Area 1 at the base of the statue is greyish/greenish in colour and it is characterized by a mix of wax and Incralac® residues, and corrosion products (-A1); Area 2 is protected by water washings by the presence of the chlamys; it is black and shiny, Incralac® residues being the main component of the outer surface with traces of microcrystalline wax (-A2); Area 3 is located on the shoulder and it shows a brilliant green colour. It is totally exposed to water washing; in this case no acrylics or wax are present (-A3).
Instrumentation
Compositional information of powder micro-samples from the corrosion layers was obtained with μFTIR spectroscopy with a Nicolet Nexus equipped with a continuum microscope (MCT detector; analysis range 4000–650 cm−1; a diamond anvil cell was used with the aim to record the spectra of micro-particles of corrosion products); identification of crystalline phases of samples was achieved with a Panalytical X'Pert PRO diffractometer (goniometer geometry Theta–Theta; diffractograms recorded between 3° and 75° 2-Theta using Cu K-alpha radiation). The study on corrosion products was also conducted with a Senterra dispersive micro-Raman spectrometer (Bruker) using two excitation lines (785 and 532 nm). Observations on cross-sections were carried out by means of a Leitz Ortholux optical microscope in reflectance mode, equipped with a Nikon camera. SEM-EDX was carried out on cross-sections using a JEOL 5910 LV equipped with an IXRF-2000 energy-dispersive X-ray spectrometer.
A Scalar DG2A portable microscope with an optical zoom ×25–200 was used. Images were recorded at ×25 magnification (area 13 × 8 mm), at ×50 (area 6.5 × 4 mm), and at ×100 (area 3.2 × 2 mm). A Minolta CM-700d spectrophotometer with D65 illuminant and an 8-mm diameter measurement area was used; 25 scans were obtained for each test area, scanning homogeneously a surface of 25 cm2.
EIS measurements were acquired with a specially designed contact probe with a nominal area of 1.77 cm2 (Letardi, Citation2004) and a Gamry Ref600 Potentiostat in the frequency range 100 KHz–10 mHz. The low frequency impedance modulus |Z|lf was used to characterize the influence of laser cleaning: its increase corresponds to a lowering of the corrosion rate. The wet footprint of the EIS contact probe was photographed with graph paper as a background immediately after measurement in order to normalize data for the active measurement area, which is influenced by surface wettability. Two measurements over each test area were averaged.
In situ FTIR spectra were collected with a portable Bruker Optics ALPHA FT-IR spectrometer equipped with SiC Globar source and a DTGS detector. The instrument was equipped with a video camera. All spectra were acquired in total reflection mode, collecting 256 scans, with a resolution of 4 cm−1 in the 7500–375 cm−1 range and a measuring spot of 6 mm in diameter. The collected IR spectra were processed using OPUS software.
Laser cleaning parameters
Preliminary laser cleaning tests were carried out with the El.En. Combo laser, used in both LQS and SFR regimes. With respect to the LQS tests, the energy ranged between 150 and 450 mJ; repetition rate ranged from 1 to 5 Hz. For FR tests energy ranged between 300 and 900 mJ. The repetition rate ranged between 1 and 20 Hz. The laser spot measured about 0.9 cm. Preliminary tests were carried out both in dry and wet conditions. The results of preliminary tests, evaluated by visual and microscopic inspection, indicated the SFR pulse duration as more suitable as it allowed a more gradual removal of material. For this purpose, three different areas A1, A2, and A3 () have been chosen to test the performance of the SFR regime in-depth (El.En. EOS 1000 Nd:YAG 1064 nm), in solving three types of cleaning problems. Specifically, laser cleaning parameters were optimized with the aim of thinning brochantite layers and of removing Incralac® residues. Each area was divided into three sections labelled (a)–(b)–(c) (see ), cleaned with laser parameters set so as to assess gradually the effects of laser cleaning and determine a safe fluence working range and a possible damage threshold. A total of nine laser tests (1a,b,c; 2a,b,c; and 3a,b,c) were carried out with the following laser cleaning parameters adopted in each section:
a. | 600 mJ and 10 Hz, F = 0.94 J/cm2; | ||||
b. | 900 mJ and 10 Hz, F = 1.42 J/cm2; | ||||
c. | 900 mJ and 10 Hz wetted by brushing. |
Evaluation of laser cleaning effects
Observations using a portable microscope
In Area 1, general thinning of the blackish layer, composed of the mixed acrylic/wax, was achieved. By increasing the fluence, more complete removal of the organic materials is obtained ( and ). Green surfaces become more evident because they are freed from the black formation in the upper layer. Only a small fraction of tiny particulate matter (white, grey, or reddish in colour) has been removed; and most of the green layer is undisturbed after cleaning, even at 1.42 J/cm2 in wet conditions (). The same effect has been observed in Area 2, even if less evident; morphological observations using the portable microscope did not provide meaningful images. This lack of information is probably due to the very flat and dark surface; however it was possible to observe that after cleaning the amount of surface green in colour is greater respect to the conditions before cleaning. In Area 3 the laser cleaning has conserved the general morphology of the corrosion products and slight thinning has been observed only in some areas (red arrow in ).
Colorimetric measurements
Colorimetric measurements before laser cleaning confirm the more homogeneous colour in Area 2, while a much larger standard deviation (st.dev.) affects the average colour values in Areas 1 and 3 due to the spotted green/black texture of the surface. In general, the colour variable most affected by laser cleaning is L*, even though the variation of the three colour coordinates is generally lower than the corresponding st.dev., with the exception of conditions (a) and (b) on Area 2. On Area 3 the laser cleaning (c) has the greatest effect on colour with a decrease in lightness and a shift toward less green and blue. L*a*b* variation in Area 1 is negligible for all the three laser cleaning conditions ().
EIS
Values obtained on surfaces before cleaning are 0.16 ± 0.06 MΩcm2 for Area 1, 0.22 ± 0.05 MΩcm2 for Area 2 and 0.11 ± 0.06 MΩcm2 for Area 3: as expected lower values are obtained in Area 3 and higher ones in Area 2.
Laser cleaning with different conditions (a), (b), and (c) had different effects on the three types of surfaces tested. Area 2 has a lower corrosion rate; on Area 3 the corrosion rate is almost unaffected by laser cleaning using (a) and (b); on Area 1 a higher sensitivity to laser cleaning parameters is observed ().
Evaluation of laser cleaning with portable FTIR
The spectral patterns achieved on all the areas were all consistent and only the most meaningful ones are reported here. In particular the removal of the double-layer coating applied in past restoration interventions, composed by acrylic resin (Incralac®) and microcrystalline wax and particularly evident in Area 2, was monitored through the typical peaks of ν(C = O) at 1723 cm−1 and δ(C–H) at 1464–1475 cm−1. After cleaning, a clear loss in the intensity of these peaks was observed on surfaces treated with parameters (a) and (b), confirming that the laser cleaning effectively reduced the thickness of the coating, and further supporting observations with the video camera mounted on the head of the instrument (). Instead, copper hydroxysulphates (antlerite and brochantite) were found in all the investigated areas both before and after cleaning, without any evident change in the spectral pattern (data not reported).
Figure 8. FTIR reflectance spectra of (I) reference double-layer coating acrylic resin (Incralac®)/microcrystalline wax laid on a bronze coupon; area 2a (II) before and (III) after laser cleaning. The removal of the coatings was evaluated through their typical peaks at 1465–1474 cm−1 (dotted-line square, microcrystalline wax) and 1723 cm−1 (dashed-line square, acrylic resin). Antlerite (*) and brochantite (°) were also found before and after cleaning. Insets show the investigated areas.
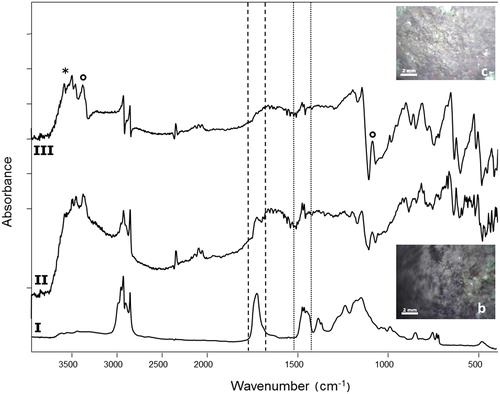
Conclusions
Laser tests resulted in a very mild and gradual degree of cleaning, avoiding important modifications of the copper-based corrosion patina and its thickness as enlightened by the different in situ measurement techniques adopted. Laser cleaning on Area 2, with Incralac® and wax remnants from previous restoration, performed with conditions (a) and (b) gave the better results. Laser cleaning in wet conditions (c) appeared less mild than desirable and less controllable; on Area 3 both colour measurements and microscopic observations corroborate this hypothesis. Taking into account these results, the whole statue was cleaned with the parameters (a) and (b), maintaining fluence in the range 0.94 J/cm2 < F < 1.42 J/cm2, and avoiding wet conditions. Only when strictly needed a piezo-electric tool was used as a first step on the most resistant encrustations. The simultaneous application of colour, FTIR, and EIS measurements proved to be useful in the evaluation of laser cleaning effectiveness on bronze artefacts.
Acknowledgements
Conservation was carried out in the framework of Bank of America–Merrill Lynch Programme for Patronage of the Arts. The authors are grateful to Amici di Brera for their support during the project.
References
- Buccolieri, G., Nassisi, V., Buccolieri, A., Vona, F. & Castellano, A. 2013. Laser Cleaning of a Bronze Bell. Applied Surface Science, 272: 55–58.
- Garbacz, H. Koss, A., Marczak, J., Mróz, J., Onyszczuk, T., Rycyk, A., Sarzyski, A., Skrzeczanowski, W., Strzelec, M. & Zatorska, A. 2010. Optimized Laser Cleaning of Metal Artworks – Evaluation of Determinants. Physics Procedia, 5: 457–66.
- Garbacz, H., Fortuna-Zalesna, E., Marczak, J., Koss, A., Zatorska, A., Zukowska, G.Z., Onyszczuk, T. & Kurzydlowski, K.J. 2011. Effect of Laser Treatment on the Surface of Copper Alloys. Applied Surface Science, 257: 7369–74.
- Korenberg, C. & Baldwin, A. 2006. Laser Cleaning Tests on Archaeological Copper Alloys Using an Nd:YAG laser. Laser Chemistry, 2006: Article ID 75831.
- Lee, H., Chob, N. & Lee, J. 2013. Study on Surface Properties of Gilt-Bronze Artifacts, after Nd:YAG Laser Cleaning. Applied Surface Science, 284: 235–41.
- Letardi, P. 2004. Laboratory and Field Test on Patinas and Protective Coating Systems for Outdoor Bronze Monuments. In: Ashton, J. & Hallam, D., eds. Metal2004 – Proceedings of the International Conference on Metals Conservation. National Museum of Australia, Canberra, pp. 379–87.
- Letardi, P. 2013. Electrochemical Measurements in the Conservation of Metallic Heritage Artefacts: An Overview. In: Dillmann, P., Watkinson, D., Angelini, E. & Adriens, A., eds, Corrosion and Conservation of Cultural Heritage Metallic Artefacts. EFC Publications Number 65, Cambridge, pp. 126–48.
- Matteini, M., Lalli, C., Tosini, I., Giusti, A. & Siano, S. 2003. Laser and Chemical Cleaning Tests for the Conservation of the Porta del Paradiso by Lorenzo Ghiberti. Journal of Cultural Heritage, 4: S147–51.
- Pini, R., Siano, S., Salimbeni, R., Pasquinucci, M. & Miccio, M. 2000. Tests of Laser Cleaning on Archeological Metal Artefacts. Journal of Cultural Heritage, 1: S129–37.
- Siano, S. & Salimbeni, R. 2010. Advances in Laser Cleaning of Artwork and Objects of Historical Interest: The Optimized Pulse Duration Approach. Accounts of Chemical Research, 43(6): 739–50.
- Siano, S., Agresti, J., Cacciari, I., Ciofini, D., Mascalchi, M., Osticioli, I. & Mencaglia, A.A. 2012. Laser Cleaning in Conservation of Stone, Metal, and Painted Artifacts: State of the Art and New Insights on the Use of the Nd:YAG Lasers. Applied Physics A, 106: 419–46.