Abstract
In this study, we applied D, L‐threo‐1‐phenyl‐2‐decanoylamino‐3‐morpholino‐1‐propanol (PDMP) hydrochloride as a chemical inhibitor for glucosylceramide synthase (GCS) and tetrandrine (Tet) for P‐glycoprotein (P‐gp) to reverse daunorubicin (DNR) resistance of human leukemia cell line K562/A02. Cytotoxicity assays showed that either PDMP or Tet enhanced cytotoxic effect of DNR on K562/A02 cells, while cotreatment of these two drugs had a more significant effect on chemosensitization. Using flow cytometric analysis, we confirmed that the enhancement effect was accompanied by elevated cellular DNR accumulation and DNR‐induced apoptosis. According to reverse transcription‐polymerase chain reaction and western blot, the reversal effect of that composite might owe to the significant downregulation of mdr1 and GCS gene expressions. Importantly, PDMP diminished mdr1 gene expression and Tet also downregulated GCS gene expression. Moreover, a positive correlation was observed between GCS and P‐gp. Thus, our results suggest that a potential clinical application of PDMP in combination with Tet may enhance chemosensitivity in leukemia.
Introduction
Development of multidrug resistance (MDR) is a major concern in cancer patients. Resistance to chemotherapy is associated with many mechanisms including, for instance, decreased concentration of intracellular drug, enhanced ability of DNA repair and altered expressions of genes and proteins involved in the control of apoptosis.Citation1 In a classic model of MDR, P‐glycoprotein (P‐gp), a transmembrane protein, effluxes different chemical substances across plasma membranes, thus preventing anticancer drugs from reaching their sites of action. As more have been studied about P‐gp, its function has expanded to a flippase for glucosylceramide which is a metabolic product of ceramide and a precursor for glycosphingolipid biosynthesis.Citation2
Known as a pivotal enzyme in ceramide metabolism, glucosylceramide synthase (GCS) transfers a glucose residue from uridine diphosphate‐glucose to ceramide, blocking apoptosis signal‐pathway mediated by ceramide.Citation3 Since GCS removes ceramide by a constant conversion into glucosylceramide, many chemotherapeutic agents are unable to induce apoptosis through accumulating of ceramide. It has been established that overexpression of GCS is a common phenomenon in a variety of MDR cancer cell lines and its related metabolite, glucosylceramide, contributes to multidrug‐resistant phenotype.Citation4Citation4,5 Surpression of GCS by RNA interference has been shown to reverse MDR in breast cancer cells, decrease human neuroepithelioma cell growth,Citation6 and inhibit melanoma formation in mice,Citation7 suggesting that blocking GCS gene expression has the potential to suppress ceramide glycosylation and to induce apoptosis and to enhance drug uptake/or cell growth arrest. Interestingly, the expression of GCS gene increased in parallel with that of mdr1 gene in terms of mRNA and protein, indicating that there is a possible connection between GCS and P‐gp.Citation5 Furthermore, RNA interference that blockades GCS also has the ability to downregulate the expression of P‐gp.Citation8
Traditional strategies toward MDR cases concentrate on enhancement of drug delivery efficiency. Tetrandrine (Tet), a bisbenzylisoquinoline albaloid isolated from the Chinese herb ‘Hanfangji’ (Stephania tetrandra Radix), is often applied to sensitize P‐gp‐mediated MDR cancer cells.Citation9Citation9,10 In addition to cause a notable downregulation of mdr1 mRNA and P‐gp, Tet could prevent doxorubicin‐induced mdr1 mRNA/P‐gp expression and P‐gp functions, thus preventing or delaying the occurrence of leukemia MDR.Citation11 Tet has been now reported to have an encouraging effect on reversing MDR in patients with poor risk forms and to be relatively well tolerated in these patients.Citation12
Recently, GCS inhibitors have been introduced as chemosensitizer to elevate the ceramide‐related apoptotic rate. D, L‐threo‐1‐phenyl‐2‐decanoylamino‐3‐morpholino‐1‐propanol (PDMP) family is the representative of GCS enzyme inhibitors, exhibiting significant similarity to endogenous ceramide and glycolipids,Citation13 which has been shown to sensitize MDR cancer cells as a result of promoting ceramide accumulation and enhancing anticancer drug concentration in cells.Citation14 Moreover, a derivative of PDMP has been used to inhibit tumor formation in miceCitation15 and to increase the cytotoxicity of anticancer drugs in tumor cells.Citation16 In Lewis lung carcinoma cells, treatment with PDMP reduced the lung colonizing capacity of cells in inoculated mice by 70%. After removal of PDMP, cancer cell capacity to metastasize was completely restored.Citation17
In our study, these two therapeutic strategies were combined to sensitize MDR cells to anticancer agents. We showed an effective combination of PDMP and Tet to enhance DNR cytotoxicity, possibly as a result of a significant increase in DNR accumulation and enhancement of DNR‐induced apoptosis. Importantly, by measuring the expressions of mdr1 and GCS genes, we found that the significant reversal effect may owe to the regulation of gene products and a positive correlation was indicated between the expressions of GCS and mdr1 genes. Taking into account that PDMP and Tet are included in many present‐day researches of possible solutions of MDR, their combined application with recombinant DNR opens broad perspectives for therapy of anti‐MDR.
Materials and Methods
Cell lines and culture conditions
Human leukemia cell line K562 and its adriamycin‐selected P‐gp‐overexpressing subline K562/A02 were obtained from the Institute of Hematology, Chinese Academy of Medical Sciences (Tianjin, China). Both cells were cultured in RPMI‐1640 medium (Gibco‐BRL, Carlsbad, CA, USA) containing 10% (v/v) heat‐inactivated new‐born calf serum (Sijiqing, Hangzhou, China), 100 U/ml penicillin and 100 μg/ml streptomycin at 37°C in a humidified 5% CO2 incubator. For maintaining MDR phenotype, K562/A02 was cultured in the medium containing 1 μg/ml adriamycin (Hisun Phamaceutical Co., Taizhou, China) and maintained in drug‐free medium for at least 7 days before use.
Cytotoxicity assays
K562 and K562/A02 cells were plated in 96‐well plates at 8×103 cells/well in complete growth medium at 37°C for 2 hours. Various concentrations of DNR (Main Luck Phamaceuticals Inc., Shenzheng, China) were added in the presence and absence of PDMP (Aliex, Lausen, Switzerland) and/or Tet (Kanghong, Chengdu, China). All these drugs were added in serum‐free 1640 medium (0·1 ml). After incubation for 48 hours, 50 μl of 5 mg/ml MTT solution (Sigma Co. Ltd, St Louis, MO, USA) was added to each well and incubated for another 4 hours, followed by reading the optical density value (absorbance) at 540 nm using a plate reader (model 550; Bio‐Rad, Tokyo, Japan) to measure cell proliferation and/or inhibition. The cytotoxicity of each treatment was expressed as a percentage of inhibition ratio compared to control cells (percentage of control) and was defined as: [1−(A540 nm treated cells)/A540 nm control cells)]×100%.
Apoptosis assay
Flow cytometry was used to measure the cell apoptosis. Briefly, K562/A02 cells (2×105/ml) were incubated with DNR (0·5 μg/ml) in the presence and absence of PDMP (20 μmol/l) and/or Tet (1 μmol/l) for 48 hours. K562/A02 cells without any drugs were regarded as a blank control. After 48 hours’ incubation in the medium containing different drugs at 37°C, cell suspensions were washed twice with phosphate‐buffered saline (PBS) and centrifugated at 1000 revolutions/minute for 5 minutes. Then cells were resuspended in 500 μl binding buffer (KenGen, Nanjing, China) and 5 μl Annexin V‐FITC (KenGen), incubated at room temperature for 15 minutes in the dark. The samples were measured on a flow cytometry with CellQuest software (Becton Dickinson, San Jose, CA, USA).
Cellular accumulation of DNR
The amount of DNR accumulated in K562/A02 or K562 cells was determined by measuring the cell‐associated fluorescence emission (488 nm) by flow cytometry. The average intracellular fluorescence intensity was proportional to the accumulation of fluorescent DNR. Briefly, K562/A02 cells (2×105/ml) were incubated with DNR (0·5 μg/ml) in the presence and absence of PDMP (20 μmol/l) and/or Tet (1 μmol/l) at 37°C. K562/A02 cells (2×105/ml) without any drugs was regarded as a blank control and K562 cells (2×105/ml) treated with DNR (0·5 μg/ml) alone was regarded as a positive control. After 48 hours, cells (2×105/ml) were washed with PBS twice and centrifugated at 1000 revolutions/minute for 5 minutes. Then samples were resuspended in 500 μl PBS and measured by flow cytometry.
RNA analysis
After treatment with PDMP and/or Tet for 48 hours, total RNA of K562 and K562/A02 cells (2×105/ml) were isolated using Trizol reagent (Gibco‐BRL). Reverse transcription‐polymerase chain reaction (RT‐PCR) was conducted according to TaKaRa LA PCR™ KitVer.2·1 (TaKaRa Biotechnology Co., Dalian, China). PCR primers (synthesized by Shanghai Sangon Co., Shanghai, China) were designed to amplify products sequences [GCS (440 bp) upstream 5′‐CTGGAAACATTCTTTGAATTGG AT‐3′ and downstream 5′‐CTCATTAAACAAGACATTCCTGTC‐3′; mdr1 (322 bp) upstream 5′‐AATGCGACAGGAGATAGG‐3′ and downstream 5′‐TCTAATTGCTGCCAAGAC‐3′; beta‐actin (155 bp) upstream 5′‐TATGACTTAGTTGCGTTACACC‐3′ and downstream 5′‐CCTT CACCGTTCCAGTTT ‐3′]. Reverse transcription was performed for 30 minutes at 42°C, 5 minutes at 99°C, and then 5 minutes at 5°C. DNA was amplified in a 30‐cycle PCR reaction, using the following conditions: denaturation at 94°C for 30 seconds, annealing at 50°C for 30 seconds (at 55·7°C, 30 seconds for GCS), and elongation at 72°C for 1 minute. RT‐PCR products were analyzed by 2% agarose gel electrophoresis stained with ethidium bromide.
Western blot
After treatment with PDMP and/or Tet for 48 hours, 1×106 cells were collected and washed in PBS. Cells lysates were prepared in buffer [30 mM Tris‐HCl (pH 8·0), 150 mmol/l NaCl, 1% Triton X‐100, 100 μg/ml phenylmethylsulfonyl fluoride] and protein concentration was determined using Modified Lowry Protein Assay Kit (Pierce, Rockford, IL, USA). Proteins were then subjected to 4–10% gradient sodium dodecyl sulfate–polyacrylamide gel electrophoresis and transferred to a polyvinylidene difuoride membrane. The transferred nitrocellulose blot was blocked with 5% fat‐free milk powder in PBS containing 0·1% Tween 20 at room temperature for 1 hour. The blots were stained with rabbit monoclonal antibodies against human P‐gp and GCS (diluted 1∶1500; Beijing Biosynthesis Biotechnology Co., Beijing, China) overnight at 4°C, and then with horseradish peroxidase conjugated goat anti‐rabbit antibody (1∶5000; Pierce) for 1 hour at room temperature. The protein bands were visualized by enhanced chemiluminescence regents (Santa Cruz Biotechnology, Inc., Santa Cruz, CA, USA).
Statistical analysis
All experiments were repeated at least three times; data were expressed as mean±SD and analyzed with the Statistical Package for Social Science (SPSS Release 11·5; SPSS Inc., Chicago, IL, USA). Statistical significance was determined by one‐way ANOVA followed by Bivariate Correlation test to adjust for multiple comparisons. P<0·05 was considered to be statistically significant.
Results
Cytotoxicity of PDMP or Tet
The cytotoxic activity of PDMP was analyzed by MTT assay. It was demonstrated that PDMP (20 μmol/l or less) had no obvious influence on the proliferation of K562 and K562/A02 cells (the inhibition ratio were below 10%) (). Our previous data showed that Tet hardly had cytotixic effect on K562/A02 and K562 cells when its dose was less than 1·5 μmol/l.Citation18 Considering low cytotoxicity, 20 μmol/l PDMP and 1 μmol/l Tet were chosen as reversal agents in our study.
Table 1. The inhibitory effects of PDMP on the proliferation of K562 and K562/A02 cells (48 hours) (
±SD, n = 3)
Sensitization of K562/A02 cells to DNR
The ability of PDMP and Tet to enhance the cytotoxic effect of DNR was evaluated by exposing K562 and K562/A02 cells to PDMP and/or Tet. After 48 hours, the IC50 (the half maximal inhibitory concentration) for DNR in K562/A02 and K562 cell lines were 7·06±0·53 and 0·23±0·02 μg/ml, respectively (P<0·001). In the presence of 20 μmol/l PDMP, the IC50 for DNR was 2·92±0·24 μg/ml and in the presence of 1 μmol/l Tet, the IC50 was 1·53±0·17 μg/ml (P<0·001). While K562/A02 cells were cotreated by PDMP and Tet, the value was 0·69±0·03 μg/ml, which was significantly different when compared with Tet or PDMP alone (P<0·05), suggesting that the two reversal agents had an enhancement effect on chemosensitization of K562/A02 cells. However, the reversal effects were not observed in K562 cells treated by PDMP, Tet solitarily and jointly (The IC50 were 0·25±0·02, 0·22±0.01 and 0·21±0·01 μg/ml respectively) (P>0·05).
Enhancement of DNR‐induced apoptosis in K562/A02 cells
PDMP and Tet were also observed to have a positive effect on DNR‐induced apoptosis. The apoptotic percentage of K562/A02 cells induced by 0·5 μg/ml DNR with 20 μmol/l PDMP or 1 μmol/l Tet were 18·06±1·46% and 21·93±1·98% respectively. While in the presence of both PDMP and Tet, the value was notably increased to 42·77±2·32%, compared with the control group (5·43±0·70%, P<0·001).The results suggested that PDMP and Tet could significantly promote the action of DNR‐induced apoptosis ().
Figure 1. Effect of PDMP and Tet on DNR‐induced apoptosis of K562/A02 cells. After incubated with DNR (0·5 μg/ml) in the presence and absence of PDMP (20 μmol/l) and/or Tet (1 μmol/l) for 48 hours, the apoptosis rate of K562/A02 cells was assessed by flow cytometry. Results are representative of at least three experiments.
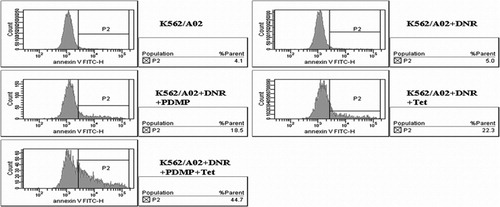
Alterations of intracellular DNR concentration
When K562/A02 cells were incubated with 0·5 μg/ml DNR alone, the average fluorescence intensity of DNR was 1554·67±91·09, which was significantly lower than their drug sensitive counterparts (4772·67±193·90) (P<0·001). Similar to Tet, the presence of PDMP also affected cellular DNR accumulation of K562/A02 cells. The mean increase was 2069·67±118·29 at 1 μmol/l Tet and 1975·33±111·54 at 20 μmol/l PDMP. However, PDMP and Tet cotreatment had even higher DNR accumulation (4435·33±151·13) than PDMP or Tet alone (P<0·001) (). These data indicated that the PDMP and Tet induced chemosensitization possibly by enhancing the intracellular DNR concentration.
Figure 2. Effect of PDMP and Tet on intracellular accumulation of DNR in K562/A02 cells. After incubated with DNR (0·5 μg/ml) in the presence and absence of PDMP (20 μmol/l) and/or Tet (1 μmol/l) for 48 hours, the average fluorescence intensity of DNR in K562/A02 cells was assessed by flow cytometry. Results are representative of at least three experiments.
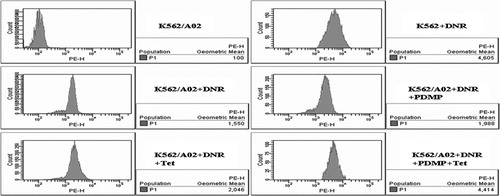
Effects of PDMP and/or Tet on the expressions of GCS and P‐gp
The expressions of GCS and mdr1 genes, measured by mRNA and protein, were dramatically higher in K562/A02 cells than those in their drug‐sensitive counterparts. Incubation of K562/A02 cells with 20 μmol/l PDMP and 1 μmol/l Tet resulted in remarkably downregulating the expressions of GCS and P‐gp, compared with single drug treatment (P<0·001). Moreover, besides the chemical lowering of their target gene expression, PDMP also diminished the transcription of mdr1 and downregulated the expression of P‐gp and Tet obviously downregulated GCS gene expression. There was a positive correlation between the levels of GCS and mdr1 mRNA (r = 0·97, P<0·05) () and such a correlation was also observed in the expression of P‐gp and GCS protein (r = 0·96, P<0·05) ().
Figure 3. Effect of PDMP and Tet on the transcriptions of (A) GCS and (B) mdr1 in K562/A02 cells. After incubated with and without PDMP (20 μmol/l) and/or Tet (1 μmol/l), RNA was extracted from K562 and K562/A02 cells and detected by RT‐PCR as detailed in the ‘Materials and methods’ section. The reverse PCR products were 440 bp for GCS, 322 bp for mdr1 and 155 bp for beta‐actin. Results shown are typical of three independent experiments.
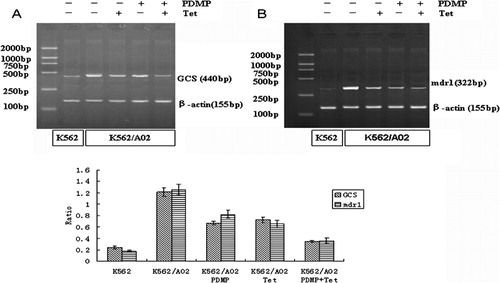
Figure 4. Effect of PDMP and Tet on expressions of GCS and P‐gp in K562/A02 cells. After K562 and K562/A02 cells were incubated in the presence and absence of PDMP (20 μmol/l) and/or Tet (1 μmol/l), protein was extracted and used in western blot as detailed in the ‘Materials and methods’ section. Results shown are typical of three independent experiments.
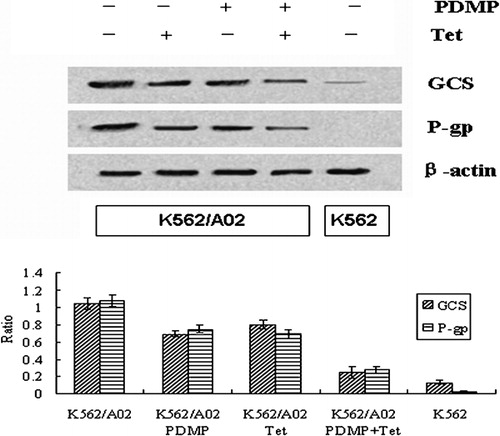
Discussion
MDR is defined as the cross‐resistance of tumor cells to a diverse range of structurally and functionally unrelated drugs. Treatment failure of leukemia is largely attributable to MDR. Among a variety of agents contributing to MDR, P‐gp is well known as a drug efflux pump reducing intracellular accumulation of chemotherapeutic drugs. GCS is another distinctive contributor to MDR which have the ability to remove ceramide metabolically. It has been reported that GCS blockade by either RNA interference or chemical inhibitors can induce sensitivity of MDR cells to anticancer drugs.Citation19Citation19,20 A few novel chemotherapeutic drugs according to these mechanisms emerge, such as Tet which is widely used to treat P‐gp‐mediated MDR, and PDMP, a chemical inhibitor for GCS. However, the therapeutic effects are not as good as what have been anticipated.
In order to find a more effective way to reverse drug resistance, we investigated whether PDMP and Tet cotreatment could exert enhancement effects on chemotherapeutic treatment of K562/A02 cells. In the cytotoxicity assay, PDMP or Tet, in nontoxic doses, could enhance DNR‐induced cytotoxicity. While they were combined, they showed a strong reduction in K562/A02 cell survival. However, the reversal effect was not observed in drug sensitive K562 cells.
Intracellular concentration of DNR in leukemia cells is a primary parameter indicating the response to therapy.Citation21Citation21,22 The inability of anticancer drug accumulation is a fundamental drug resistance mechanism. Flow cytometric assay indicated that the chemosensitizing effect of PDMP and Tet cotreatment resulted in more intracellular concentration of DNR. The ability of tumor cells to activate apoptotic response has also been an ingredient for chemosensitization. In our study, compared with either PDMP or Tet alone group, DNR‐induced apoptosis rate was obviously high when the two reverse agents were combined. These results suggested that the enhancement effect of PDMP and Tet on DNR cytotoxicity correlated with significantly increased DNR accumulation and enhanced cell apoptosis rate.
Because of pronounced difference of drug concentration and cell apoptosis, we explored the transcription of mdr1 and GCS mRNA by RT‐PCR and the expressions of P‐gp and GCS by western blot. It has been reported that GCS and mdr1 mRNA of clinic MDR samples exhibited higher levels than those of drug sensitive group.Citation23 In our present study, we also found overexpressions of GCS and mdr1 genes in K562/A02 cells. PDMP and Tet could limit their target gene expression respectively, while being together, they produced a more significant reduction of their target mRNA and protein products. Interestingly, a positive correlation between expressions of GCS and mdr1 genes was observed, indicating the relationship of ceramide metabolism and drug transporter in the context of chemosensitization.
Previous studies showed that PDMP treatment increased the retention of Rh123, a specific P‐gp substrate,Citation24 and decreased the efflux of both paclitaxel and vincristine,Citation14 similar to treatment with a P‐gp inhibitor. However, PDMP has been described to exert its modulating effect on P‐gp function through reduction of glycosylated forms of Cer. The inhibitory effect of PDMP on P‐gp function could rather be related to depletion of glycosylated Cer derivatives including monosialo ganglioside (GM3), which was a positive regulator of P‐gp through phosphorylation.Citation24 Thereby, PDMP modulates P‐gp through an indirect mechanism. Other studies reported that limiting GCS activity by either RNA interference or chemical inhibitor downregulated the expression of P‐gp and the decrease in GCS expression also had an important influence on cellular drug concentration.Citation8Citation8,14 In our study, PDMP treatment did result in a diminished expression of mdr1 genes and decreased efflux of anticancer drugs, similar to treatment with Tet, the P‐gp inhibitor. Moreover, in addition to a role of drug efflux pump, P‐gp has also been implicated in ceramide metabolism. It has been documented that P‐gp translocates glucosylceramide across Golgi membrane,Citation25 thus modulating GCS activity.Citation26 In this study, Tet did not only downregulate P‐gp but also GCS, which supported the proposal that P‐gp inhibitors influence GCS activity in intact P‐gp‐expressing cells.Citation27
Conclusion
In summary, we have demonstrated a chemosensitizing effect of PDMP and Tet on K562/A02 cells treated with DNR which may be associated with significantly enhanced DNR‐induced apoptosis and cellular DNR concentration. Furthermore, regulation of GCS and mdr1 genes products may also be an important contributor. It may be possible that a combination of PDMP and Tet becomes a more sufficient and less toxic anti‐MDR method.
BAC and LY designed the research, conducted experiments, analyzed results and wrote this paper. JC, JHD, CG, GZ, JW, YYS, WB and XMW designed the research. GHX contributed to the composition of the manuscript. This work was supported by National Natural Science Foundation of China (no. 30740062 and no. 39970832) and High School Doctor Subject Special‐Purpose Scientific Research Foundation (no. 20070286042).
References
- Volm M, Kästel M, Mattern J, Efferth T. Expression of resistance factors (P-glycoprotein, glutathione S-transferase-pi, and topoisomerase II) and their interrelationship to proto-oncogene products in renal cell carcinomas. Cancer 1993;71:3981–7.
- Lala P, Ito S, Lingwood CA. Retroviral transfection of Madin–Darby canine kidney cells with human MDR1 results in a major increase in globotriaosylceramide and 10(5)- to 10(6)-fold increased cell sensitivity to verocytotoxin. Role of P-glycoprotein in glycolipid synthesis. J Biol Chem 2000;275:6246–51.
- Reynolds CP, Maurer BJ, Kolesnick RN. Ceramide synthesis and metabolism as a target for cancer therapy. Cancer Lett 2004;206:169–80.
- Uchida Y, Itoh M, Taguchi Y, Yamaoka S, Umehara H, Ichikawa S, et al.. Ceramide reduction and transcriptional up-regulation of glucosylceramide synthase through doxorubicin-activated Sp1 in drug-resistant HL-60/ADR cells. Cancer Res 2004;64:6271–9.
- Gouazé V, Yu JY, Bleicher RJ, Han TY, Liu YY, Wang H, et al.. Overexpression of glucosylceramide synthase and P-glycoprotein in cancer cells selected for resistance to natural product chemotherapy. Mol Cancer Ther 2004;3:633–9.
- Di Sano F, Di Bartolomeo S, Fazi B, Fiorentini C, Matarrese P, Spinedi A, et al.. Antisense to glucosylceramide synthase in human neuroepithelioma affects cell growth but not apoptosis. Cell Death Differ 2002;9:693–5.
- Deng W, Li R, Guerrera M, Liu Y, Ladisch S. Transfection of glucosylceramide synthase antisense inhibits mouse melanoma formation. Glycobiology 2002;12:145–52.
- Gouazé V, Liu YY, Prickett CS, Yu JY, Giuliano AE, Cabot MC. Glucosylceramide synthase blockade down-regulates P-glycoprotein and resensitizes multidrug-resistant breast cancer cells to anticancer drugs. Cancer Res 2005;65:3861–7.
- Fu L, Liang Y, Deng L, Ding Y, Chen L, Ye Y, et al.. Characterization of tetrandrine, a potent inhibitor of P-glycoprotein-mediated multidrug resistance. Cancer Chemother Pharmacol 2004;53:349–56.
- Zhao QX, Chen BA, Cheng J, Ding JH, Gao F, Gao C, et al.. Effect of tetrandrine, Toremifene and their combination on the reversion of multidrug resistance of K562/A02 cell line. Zhongguo Shi Yan Xue Ye Xue Za Zhi 2008;16:61–4.
- Shen H, Xu W, Chen Q, Wu Z, Tang H, Wang F. Tetrandrine prevents acquired drug resistance of K562 cells through inhibition of mdr1 gene transcription. J Cancer Res Clin Oncol 2010;136:659–65.
- Xu WL, Shen HL, Ao ZF, Chen BA, Xia W, Gao F, et al.. Combination of tetrandrine as a potential-reversing agent with daunorubicin, etoposide and cytarabine for the treatment of refractory and relapsed acute myelogenous leukemia. Leuk Res 2006;30:407–13.
- Lee L, Abe A, Shayman JA. Improved inhibitors of glucosylceramide synthase. J Biol Chem 1999;274:14662–9.
- Sietsma H, Veldman RJ, Kolk D, Ausema B, Nijhof W, Kamps W, et al.. 1-phenyl-2-ecanoylamino-3-morpholino-1-propanol chemosensitizes neuroblastoma cells for taxol and vincristine. Clin Cancer Res 2000;6:942–8.
- Deng W, Li R, Ladisch S. Influence of cellular ganglioside depletion on tumor formation. J Natl Cancer Inst 2000;92:912–7.
- Senchenkov A, Litvak DA, Cabot MC. Targeting ceramide metabolism – a strategy for overcoming drug resistance. J Nat Cancer Inst 2001;93:347–57.
- Inokuchi J, Jimbo M, Momosaki K, Shimeno H, Nagamatsu A, Radin NS. Inhibition of experimental metastasis of murine Lewis lung carcinoma by an inhibitor of glucosylceramide synthase and its possible mechanism of action. Cancer Res 1990;50:6731–7.
- Wang JQ, Chen BA, Cheng J, Xu WL, Sun XC. Comparison of reversal effects of 5-bromotetrandrine and tetrandrine on P-glycoprotein-dependent resistance to adriamycin in human lukemia cell line K562/A02. Ai Zheng 2008;27:491–5.
- Liu YY, Han TY, Yu JY, Bitterman A, Le A, Giuliano AE, et al.. Oligonucleotides blocking glucosylceramide synthase expression selectively reverse drug resistance in cancer cells. J Lipid Res 2004;45:933–40.
- Weiss M, Hettmer S, Smith P, Ladisch S. Inhibition of melanoma tumor growth by a novel inhibitor of glucosylceramide synthase. Cancer Res 2003;63:3654–8.
- Marie JP, Faussat-Suberville AM, Zhou D, Zittoun R. Daunorubicin uptake by leukemic cells: correlations with treatment outcome and mdr1 expression. Leukemia 1993;7:825–31.
- Guerci A, Merlin JL, Missoum N, Feldmann L, Marchal S, Witz F, et al.. Predictive value for treatment outcome in acute myeloid leukemia of cellular daunorubicin accumulation and P-glycoprotein expression simultaneously determined by flow cytometry. Blood 1995;85:2147–53.
- Xie P, Shen YF, Shi YP, Ge SM, Gu ZH, Wang J, et al.. Overexpression of glucosylceramide synthase in associated with multidrug resistance of leukemia cells. Leuk Res 2008;32:475–80.
- Plo I, Lehne G, Beckstrøm KJ, Maestre N, Bettaïeb A, Laurent G, et al.. Influence of ceramide metabolism on P-glycoprotein function in immature acute myeloid leukemia KG1a cells. Mol Pharmacol 2002;62:304–12.
- Halter D, Neumann S, van Dijk SM, Wolthoorn J, de Mazière AM, Vieira OV, et al.. Pre- and post-Golgi translocation of glucosylceramide in glycosphingolipid synthesis. J Cell Biol 2007;179:101–15.
- Shabbits JA, Mayer LD. P-glycoprotein modulates ceramide-mediated sensitivity of human breast cancer cells to tubulin-binding anticancer drugs. Mol Cancer Ther 2002;1:205–13.
- Turzanski J, Grundy M, Shang S, Russell N, Pallis M. P-glycoprotein is implicated in the inhibition of ceramide-induced apoptosis in TF-1 acute myeloid leukemia cells by modulation of the glucosylceramide synthase pathway. Exp Hematol 2005;33:62–72.