Abstract
Brain and acute leukemia, cytoplasmic (BAALC) is a novel molecular marker indicating an inferior outcome in acute myeloid leukemia (AML) with normal cytogenetics. The biological function of BAALC is largely unknown. In this study, BAALC gene expression in an acute myeloid leukemia cell line KG1a was knocked down by a small hairpin RNA (shRNA). The expression of BAALC mRNA and protein in the knockdown cells was significantly inhibited. The proliferation and apoptosis status in the knockdown cells were investigated. The growth curves and FACS analysis demonstrated that BAALC gene knockdown resulted in decreased proliferation and enhanced apoptosis in KG1a cells. These results indicated that BAALC may act as an adverse prognostic factor through prompting proliferation and inhibiting apoptosis in leukemia cells.
Keywords:
Introduction
Acute myeloid leukemia (AML) is a heterogeneous diseases characterized by a block in differentiation of hematopoiesis, resulting in growth of a clonal population of neoplastic cells or blasts.Citation1,Citation2 The clinical outcomes of AML are very unpredictable, from survival of a few days to complete cure.Citation3,Citation4 The cytogenetic aberrations have recently been identified as important prognostic factors. The cytogenetic risk systems classify AML patients into three groups, i.e. favourable, intermediate and adverse.Citation5,Citation6 AML patients with normal cytogenetics, who are classified in the intermediate prognostic category, constitute the largest cytogenetic subset of AML, approximately 45%. Only 20–42% of karyotypically normal patients are long-term survivors.Citation7–Citation9 Therefore, identifying prognostic markers that can more precisely predict patient outcome is vital for development of molecular risk-adapted treatment strategies that may improve the clinical outcome of AML.
The influence of altered gene expression on transcription factor activity and signal transduction pathways has demonstrated a critical role in leukemogenesis. The characterization of these genes and their underlying pathways could potentially aid distinguishing prognostically different subsets of AML patients, especially those with normal karyotype and may lead to development of new targeted therapies.Citation10–Citation12
Human brain and acute leukemia, cytoplasmic (BAALC) gene, located on human chromosome 8q22·3 and encoding a protein with no homology to known proteins or functional domains was identified.Citation13,Citation14 The expression of BAALC was clearly related to inferior outcomes and may be an important risk factor in AML with normal cytogenetics.Citation15–Citation17 In comparison to low BAALC-expressing patients, the high BAALC-expressing patients have a higher rate of primary resistant leukemia as well as a higher cumulative incidence of relapse, resulting in significantly inferior overall survival.Citation18 Multivariable analysis identified BAALC expression as an independent prognostic factor. Although the prognostic value of BAALC-expression has been shown in cytogenetically normal acute myeloid leukemia (CN-AML), the function of BAALC remains largely unknown. Functional studies investigating the impact of BAALC expression in leukemia cell lines could not demonstrate a mechanistic role of BAALC on proliferation and apoptosis.
In the current study, we investigated the effect of short hairpin RNA (shRNA)-mediated BAALC gene knockdown on proliferation and apoptosis in KG1a cells. Results showed that BAALC gene knockdown can inhibit proliferation and induce apoptosis in KG1a cells. Our results indicate that specifically targeting BAALC may improve the therapeutic outcome of AML.
Materials and methods
Materials
Bbs I, BamH I, Pst I restriction enzymes (NEB, USA), T4 DNA ligase, reverse transcriptase (RT) kit (Takara Co. Ltd, Shenyang, China), polymerase chain reaction (PCR) master mix (Toyobo, Japan), pGPU6/GFP/Neo siRNA plasmid vector (Genepharma Biotec, Shanghai, China), Nucleofector system (Lonza, Germany), fetal bovine serum (Gibco, USA), plasmid extraction kit (Promega, Madison, USA), RPMI-1640, G418 (Sigma, USA), Trizol (Invitrogen Biotec, Shanghai, China), ECL kit (Keygen Biotec, Nanjing, China), Cell Counting Kit-8 (CCK-8) assay kit (Beyotime Biotec, Jiangsu, China), 12-O-tetradecanoyl-phorbol-13-acetate (Sigma, USA) and nitroblue tetrazolium (NBT; Sigma, USA), Annexin V-FITC and Tunel apoptosis detection kit (Keygen Biotec, Nanjing, China).
Cell lines and culture
Human acute myeloid leukemia cell line KG1a, purchased from the German Collection of Microorganisms and Cell Cultures (Braunschweig, Germany), were cultured in RPMI-1640 supplemented with 10% FBS and 2 mM l-glutamine in a humidified atmosphere with 5% CO2 at 37°C.
Generation of BAALC short hairpin RNA expression plasmids
Basic Local Alignment Search Tool (BLAST 2) homology searching was used to identify conserved cDNA fragments for RNA interference (RNAi) within the coding region of the BAALC gene. Four 19-nt siRNAs targeting different nucleotide sites of BAALC mRNA were designed, and four pairs of 57-nt oligonucleotides coding shRNAs containing 19-nt reverted repeat, 9-nt spacer sequence, Bbs I and BamH I restriction enzyme sites were synthesized. Oligonucleotides were annealed and inserted into the shRNA expression vector pGPU6/GFP/Neo to form pGPU6/GFP/Neo-BAALC-shRNA1-4. pGPU6/GFP/Neo-shRNA-NC (Shanghai GenePharma Co., Shanghai, China) containing a scrambled sequence with no significant similarity to mouse, rat, or human gene sequences was used as a negative control vector. The ‘housekeeping’ gene glyceraldehyde phosphodehydrogenase (GAPDH) was used as positive control and pGPU6/GFP/Neo-shRNA-GAPDH was also constructed. Oligonucleotide sequences are shown in .
Table 1. Oligonucleotide sequences of shRNAs
Stable transfection of KG1a cells
BAALC short hairpin RNA expression plasmids, pGPU6/GFP/Neo-shRNA-NC and pGPU6/GFP/Neo-shRNA-GAPDH with a neomycin resistance gene were transfected into KG1a cells at a concentration of 40 nM using the Nucleofector system (Lonza kit, Germany) according to the manufacturer’s protocol. Two days after transfection, green fluorescent protein (GFP) expression was observed. For all studies, pooled populations of G418-resistant cells were used to eliminate clone variations. The cells were continuously cultured in G418 (400 µg/mL) containing medium.
Reverse transcriptase polymerase chain reaction
Total RNA was extracted from 2×106 cells using Trizol followed by phenol–chloroform extraction and isopropanol precipitation. Total RNA (1 μg) was incubated at 37°C for 100 minutes, then heated at 87°C for 5 minutes and used as a template for first-strand complementary DNA (cDNA) synthesis, using a RT kit. GAPDH was used as an internal standard yielding a polymerase chain reaction (PCR) product of 224 bp. The primers and probes were used as follows: BAALC, forward(F) 5′-CCATCGAGCCCCGCTACT-3′, reverse(R) 5′-TGTAGGTGAGCCAGGTGGATT-3′, yielded a PCR product of 67 bp; GAPDH, F 5′-GAAGGTGAAGGTCGGAGTC-3′, R 5′-GAAGATGGTGATGGGATTTC-3′. Standard RT–PCR was performed on an MJ 9700 (Applied Biosystems). The PCR protocol consisted of 35 cycles of 94°C for 30 seconds, 56°C for 45 seconds, and 72°C for 1 minute. In all experiments, two negative controls were carried through all steps. The PCR amplification products were separated in 1% agarose and visualized by ethidium bromide (EB) staining.
Western blot analysis
The cytoplasmic protein (50 μg/lane) from each sample was resolved in 10% SDS–polyacrylamide gel electrophoresis (PAGE), transferred to a PVDF membrane (Millipore, UK) and blotted with the various antibodies. Non-specific binding was avoided by blocking the nitrocellulose membrane with 5% skimmed milk in TBS-T for 1 hour. The 5% skimmed milk in TBS-T was also used to dilute primary (BAALC, mouse monoclonal antibody, 1∶100, CST) and HRP-conjugated monoclonal secondary (1∶5000; Amersham Pharmacia Biotech, NJ, USA) antibodies. The membranes were incubated with the primary antibodies overnight at 4°C and in the secondary antibody for 1 hour at room temperature. The quantity of protein loaded was verified by staining the same membranes with anti-beta actin antibody (1∶2000, Sigma-Aldrich, Dorset, UK). The signals were detected on X-ray films using an ECL Western blotting detection kit (Amersham, Pharmacia Biotech).
Proliferation assay
The CCK-8 assay was performed to assess the effect on cell proliferation. Stably transfected blast cells were plated in 96-well plates at a density of 3·0×103 cells/well, then for 4 days, every 24 hours a batch of cells were stained with 10 μL sterile CCK-8 dye (3 mg/mL) then incubated in medium at 37°C for 2 hours. Spectrometric absorbencies at 450 and 630 nm were measured using a microplate reader. All groups were measured in triplicate. Results were plotted as mean±SD of these separate experiments having six determinations per experiment for each experimental condition.
Statistical analysis
All statistical analysis was conducted with SPSS 13·0 software. Means were compared using an adjusted Student’s t-test. Values were considered significantly different when P<0·05.
Results
Identification of the recombinant plasmid vector
To validate the BAALC-targeting shRNA expression vector, recombinant plasmid DNA was extracted and agarose gel electrophoresis was conducted. A single electrophoretic band smaller than 100 bp by 1% agarose gel electrophoresis indicated the success of Oligo single strand annealing (). The shRNA expression vectors targeting BAALC were digested by BamH I and Pst I restriction endonuclease, and the successfully constructed plasmid could be digested by BamH I and Pst I ().
BAALC shRNA reduced BAALC mRNA and protein expression
The BAALC shRNA-expressing plasmid was constructed using the pGPU6/GFP/Neo vector to target human BAALC gene; shRNA-NC and shRNA-GAPDH plasmids were also constructed to act as a negative and positive control, respectively. To examine the efficacy of plasmids in silencing BAALC mRNA expression, they were stably transfected into leukemia cells. Total RNA was isolated from the transfected cells and the mRNA levels of BAALC and GAPDH were measured using RT–PCR. As shown in , BAALC mRNA expression was markedly decreased in the pGPU6/GFP/Neo-BAALC-shRNA1-4 transfected cells. Consistently, the GAPDH mRNA levels in KG1a cells were also more poorly transfected with the positive control plasmid of shRNA-GAPDH, whereas no change was observed in the pGPU6/GFP/Neo-shRNA-NC mock transfected cells. The protein levels of the BAALC gene were also analysed. In line with the mRNA expression changes, the levels of BAALC protein were also remarkably inhibited in different pools of cells transfected with BAALC shRNA-expressing plasmid compared with those transfected with shRNA-NC-expressing plasmid.
Figure 2. Effects of BAALC shRNA on mRNA and protein expression. (A) Results of 1% agarose gel electrophoresis showed that BAALC mRNA expression was decreased in cells transfected with pGPU6-BAALC-shRNA1-4. However, no change was observed in cells transfected with pGPU6-shRNA-NC. Lane 1: control; Lane 2: GAPDH-shRNA; Lane 3: GAPHD-shRNA-NC; BAALC: Lane 3: BAALC-shRNA-NC; Lanes 4–7: BAALC-shRNA-1-4. (B) Results of western blot showed that BAALC protein levels were markedly inhibited in pGPU6-BAALC-shRNA1-4 transfected leukaemia cells, in contrast to the negative control plasmid-transfected cells. Lane 1: BAALC-shRNA-NC, Lanes 2–5: BAALC-shRNA-1-4.
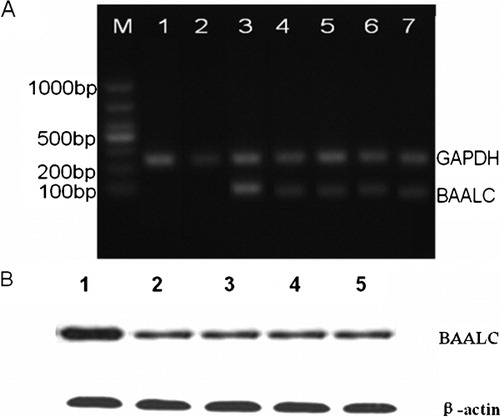
Decreased cell proliferation caused by knocking down BAALC
CCK-8 assays were conducted to determine whether silencing of BAALC affected the growth of leukemia cells. As shown in , proliferation of KG1a-BAALC shRNA cells was significantly suppressed at each different time point (48, 72 and 96 hours), compared with the control groups during the time course (P<0·05). These growth curves indicated that BAALC shRNA could inhibit the growth of cells.
BAALC knockdown induced apoptosis in leukemia cells
To explore the effect of BAALC knock down on leukemia cell apoptosis, BAALC shRNA-expressing plasmid was transfected into KG1a cells. First, the apoptotic nuclear changes (i.e. punctuated or granular and bright nuclei) were determined after DAPI staining and visualized by fluorescence microscopy. The apoptotic cellular nuclear changes () were observed predominantly in the pGPU6/GFP/Neo-BAALC-shRNAs transfected but not pGPU6/GFP/Neo -shRNA-NC transfected cells. In addition, the proportion of apoptotic cells was also determined by FACS analysis. As shown in able 2, Annexin V-FITC results showed that BAALC shRNA induced apoptosis relative to cells transfected with pGPU6/GFP/Neo-shRNA-NC (12·67% vs 85·33%, P<0·05).
Figure 4. Effects of BAALC shRNA on apoptosis in KG1a cells. (A) KG1a cells were stained with DAPI and observed under fluorescence microscopy (punctuated, granular, and brighter nuclei). (B) Results of Annexin V-FITC (measured by FACS).
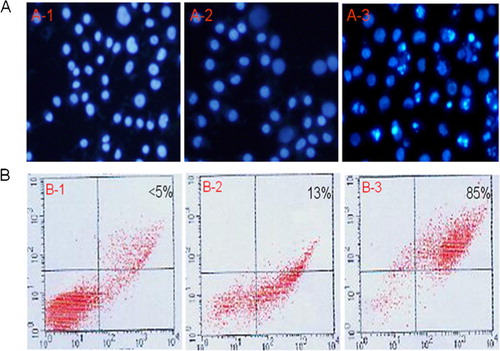
Table 2. Apoptosis of KG1a cells after different treatments
Discussion
RNA interference (RNAi) describes a conserved biological response to double-stranded RNA (dsRNA) resulting in the degradation of homologous messenger RNA. In the last few years, this process of sequence-specific, post-transcriptional gene silencing has become a key technique for rapidly assessing gene function in species ranging from plants to mammals. Many reports have shown promising therapeutic potential of small hairpin RNA (shRNA) in modulating gene function.Citation19,Citation20 In order to investigate the effect of BAALC gene on proliferation and apoptosis in acute myeloid leukemia cells, we stably knocked down BAALC expression in a human acute myeloid leukemia cell line KG1a, which highly expresses BAALC gene. Results demonstrated that knockdown of BAALC gene by BAALC shRNA down-regulated both mRNA and protein expression of BAALC in KG1a cells. This result indicates that BAALC shRNA expression plasmids were successfully constructed and transfected into KG1a cells.
BAALC expression was commonly detected in haematopoietic precursors and neuroectoderm-derived tissues. It encodes a protein with no homology to currently known proteins or functional domains.Citation13,Citation14 High expression of BAALC mRNA in circulating blasts was reported in patients with de novo CN-AML and was an indicating factor for failure to achieve complete remission (CR), associated with primary resistant disease and significantly shorter overall survival (OS) as well as a higher cumulative incidence of relapse (CIR).Citation18,Citation21,Citation22 However, there has not been a report describing the function of BAALC in the literature. To investigate the role of BAALC with the apoptosis and proliferation of KG1a cells, we transfected the BAALC shRNA expression vector into KG1a cells, then determined any potential effects on the proliferation and apoptosis of KG1a cells. Knockdown of BAALC expression in KG1a cells resulted in significant inhibition of cell proliferation when compared with the control cells. Enhanced apoptosis was also observed in the BAALC knockdown cells. The results indicate that BAALC expression contributed to the development of KG1a cells by a possible pathway of enhancing cell proliferation, inhibiting apoptosis. Our study provides in vitro evidence supporting the role of BAALC as an oncogene. The results are in accordance with our previous report and other research, showing that high levels of BAALC mRNA are related to higher proliferation of bone marrow blasts leading to higher peripheral white blood cell counts and an inferior clinical outcome.
In conclusion, our results showed that shRNA-mediated silencing of BAALC inhibited proliferation and induced apoptosis of KG1a cells. Targeting BAALC may be a novel and effective approach for leukaemia treatment.
This work was supported by grant no. 2007B030704010 from the Scientific and Technology Program Foundation of Guangdong Province.
References
- Estey E, Döhner H. Acute myeloid leukaemia.Lancet. 2006;368:1894–907.
- Smith M, Barnett M, Bassan R, Gatta G, Tondini C, Kern W. Adult acute myeloid leukaemia. Crit Rev Oncol Hematol. 2004;50:197–222.
- Ferrara F. Unanswered questions in acute myeloid leukaemia. Lancet Oncol. 2004;5:443–50.
- Avivi I, Rowe JM. Prognostic factors in acute myeloid leukemia. Curr Opin Hematol. 2005;12:62–7.
- Marcucci G, Haferlach T, Döhner H. Molecular genetics of adult acute myeloid leukemia: prognostic and therapeutic implications. J Clin Oncol. 2011 Feb 10;29(5):475–86.
- Marchesi F, Annibali O, Cerchiara E, Tirindelli MC, Avvisati G. Cytogenetic abnormalities in adult non-promyelocytic acute myeloid leukemia: A concise review. Crit Rev Oncol Hematol. 2011;80(3):331–46.
- Mrózek K, Heerema NA, Bloomfield CD. Cytogenetics in acute leukemia. Blood Rev. 2004;18:115–36.
- Zheng J, Wang X, Hu Y, Yang J, Liu J, He Y, et al.. A correlation study of immunophenotypic, cytogenetic, and clinical features of 180 AML patients in China. Cytometry B Clin Cytom. 2008;74:25–9.
- Farag SS, Ruppert AS, Mrózek K, Mayer RJ, Stone RM, Carroll AJ, et al.. Outcome of induction and postremission therapy in younger adults with acute myeloid leukemia with normal karyotype: a cancer and leukemia group B study. J Clin Oncol. 2005;23:482–93.
- Dunna NR, Rajappa S, Digumarti R, Vure S, Kagita S, Damineni S, et al.. Fms Like Tyrosine Kinase (FLT3) and Nucleophosmin 1 (NPM1) mutations in de novo normal karyotype acute myeloid leukemia (AML). Asian Pac J Cancer Prev. 2010;11:1811–6.
- Damm F, Oberacker T, Thol F, Surdziel E, Wagner K, Chaturvedi A, et al.. Prognostic importance of histone methyltransferase MLL5 expression in acute myeloid leukemia. J Clin Oncol. 2011;29:682–9.
- Taskesen E, Bullinger L, Corbacioglu A, Sanders MA, Erpelinck CA, Wouters BJ, et al.. Prognostic impact, concurrent genetic mutations, and gene expression features of AML with CEBPA mutations in a cohort of 1182 cytogenetically normal AML patients: further evidence for CEBPA double mutant AML as a distinctive disease entity. Blood. 2011;117:2469–75.
- Baldus CD, Tanner SM, Kusewitt DF, Chapelle A. BAALC, a novel marker of human hematopoietic progenitor cells. Exp Hematol. 2003;31(11):1051–6.
- Tanner SM, Austin JL, Leone G, Rush LJ, Plass C, Heinonen K, et al.. BAALC, the human member of a novel mammalian neuroectoderm gene lineage, is implicated in hematopoiesis and acute leukemia. Proc Natl Acad Sci U S A. 2001,98(24):13901–6.
- Langer C, Radmacher MD, Ruppert AS, Whitman SP, Paschka P, Mrózek K, et al.. High BAALC expression associates with other molecular prognostic markers, poor outcome, and a distinct gene-expression signature in cytogenetically normal patients younger than 60 years with acute myeloid leukemia: a Cancer and Leukemia Group B (CALGB) study. Blood. 2008;111(11):5371–9.
- Eid MA, Attia M, Abdou S, El-Shazly SF, Elahwal L, Farrag W, et al.. BAALC and ERG expression in acute myeloid leukemia with normal karyotype: impact on prognosis. Int J Lab Hematol. 2010;32(2):197–205.
- Schwind S, Marcucci G, Maharry K, Radmacher MD, Mrózek K, Holland KB, et al.. BAALC and ERG expression levels are associated with outcome and distinct gene and microRNA expression profiles in older patients with de novo cytogenetically normal acute myeloid leukemia: a Cancer and Leukemia Group B study. Blood. 2010;116(25):5660–9.
- Santamaría C, Chillón MC, García-Sanz R, Pérez C, Caballero MD, Mateos MV, et al.. BAALC is an important predictor of refractoriness to chemotherapy and poor survival in intermediate-risk acute myeloid leukemia (AML). Ann Hematol. 2010;89(5):453–8.
- Kurreck J. RNA interference: From basic research to therapeutic applications. Angew Chem Int Ed Engl. 2009;48(8):1378–98.
- Angaji SA, Hedayati SS, Poor RH, Madani S, Poor SS, Panahi S. Application of RNA interference in treating human diseases. J Genet. 2010;89:527–37.
- Heesch S, Schlee C, Neumann M, Stroux A, Kühnl A, Schwartz S, et al.. BAALC-associated gene expression profiles define IGFBP7 as a novel molecular marker in acute leukemia. Leukemia. 2010;24:1429–36.
- Zaidi SZ, Owaidah T, Al Sharif F, Ahmed SY, Chaudhri N, Aljurf M. The challenge of risk stratification in acute myeloid leukemia with normal karyotype. Hematol Oncol Stem Cell Ther. 2008;1:141–58.