Abstract
The kinetic and mechanistic aspects of the visible-light-mediated photodegradation of the phenolic antioxidants (PA), propyl gallate (PG), and t-butylhydroquinone (TBHQ), employing riboflavin (Rf) as photosensitizer, have been studied by time-resolved and stationary techniques. The photosensitizer Rose Bengal (RB) was used for auxiliary experiments. Results show the occurrence of chemical transformations on PA with the participation of electronically excited states of Rf and different reactive oxygen species (ROS) generated from these states. With 0.02 mM Rf and 1.0 mM PA, the electronically excited triplet state of Rf is quenched by PA, in a competitive manner with the dissolved oxygen. As a consequence, a cascade of photoprocesses produces singlet oxygen (O2(1Δg)) and H2O2 in the case of PG and, O2(1Δg), H2O2 and HO• in the case of TBHQ. The participation of these species is supported by experiments of oxygen consumption carried out in the presence of specific ROS scavengers. TBHQ has a relatively high capacity for O2(1Δg) physical deactivation and a low photodegradation efficiency by the oxidative species. Comparatively, it can be asserted that TBHQ has a higher antioxidant capacity than PG.
Introduction
Lipid oxidation in food is one of the main reasons for quality degradation during processing and storage. Deterioration in flavor, color, texture, nutritive value, and the production of toxic compounds are some of the negative consequences of lipid oxidation.Citation1–Citation3 There have been numerous methods developed to control the rate and extend of lipid oxidation in foods, with one the most effective being the addition of antioxidants.Citation2,Citation3 Butylated hydroxyanisole, butylated hydroxytoluene, propyl gallate (PG), and t-butylhydroquinone (TBHQ) are the most widely used antioxidants in the food industry, especially for oils and fatty foods.Citation4,Citation5 These synthetic antioxidants are exposed to daylight during the manufacture and storage of foods. Nevertheless, the antioxidants are transparent to visible light and their photodegradation depends on the presence in the protected medium of an adequate daylight-absorbing substance, named photosensitizer. Most of the commercial compounds are colored and/or possess different dyes and pigments that can act as photosensitizers and start an unpredictable series of photoreactions, many of them driven through the action of the so-called reactive oxygen species (ROS).Citation4 These photoreactions can involve antioxidant preservatives and affect their specific capacity, being this aspect of enormous importance in the commercial, health, and safety fields. The most frequent ROS generated by photosensitized processes are singlet oxygen (O2(1Δg)), superoxide radical anion (O2•−), hydrogen peroxide (H2O2), hydroxyl radical (HO•), and different free radicals, generally produced as primary reaction products.Citation6
We have recently studied kinetic and mechanistic aspects of the photosensitized reactions involving butylated hydroxyanisole and butylated hydroxytoluene. Both O2•− and O2(1Δg) produce the photodegradation of butylated hydroxytoluene. Butylated hydroxyanisole mainly interacts with O2(1Δg) and exhibits a desirable property as antioxidant: a relatively high capacity for O2(1Δg) physical deactivation and a low photodegradation efficiency by oxidative species.Citation7
The main aim of this work is to investigate kinetic and mechanistic aspects of the sensitized photoreactions involving the phenolic antioxidants (PA), PG, and TBHQ (generically named PA in the following). The structural formulae of both are shown in Scheme . For this purpose riboflavin (Rf; vitamin B2) was used as dye-photosensitizer. It can act as a prooxidant for food components under visible light. Rf-photosensitization causes production of free radicals and ROS such as O2(1Δg), O2•−, H2O2, and HO•. These species could produce not only the destruction of lipids, proteins, carbohydrates, and vitamins, but also the formation of off-flavor and loss of nutrients in foods.Citation8–Citation11
As PA and Rf can occupy common locations in foods, kinetic, and mechanistic information about the visible-light-promoted interactions between the antioxidants and the vitamins can contribute to the understanding of important aspects connecting the photoprotection activity and the degradability of these antioxidants.
Materials and methods
Materials
PG, TBHQ, Rf, Rose Bengal (RB), sodium azide (NaN3), catalase (CAT) from bovine liver, and superoxide dismutase (SOD) from bovine erythrocytes were purchased from Sigma Chem. Co. (St Louis, USA). Benzoic acid sodium salt (NaBz), 9,10-dimethylanthracene (DMA), d-mannitol and deuterated methanol (MeOD) was provided by Aldrich (St Louis, USA); whereas methanol (MeOH), HPLC quality, by Sintorgan and H2O2, by Tensol (Argentine).
Methods
Stationary photolysis
Stationary aerobic photolysis of MeOH solutions containing 0.04–1.0 mM PA and 0.02 mM Rf was carried out in a home-made photolyser. This is provided with quartz-halogen lamp (OSRAM XENOPHOT® HLX 64640, 150 W–24 V–G6.35–6000lm, OSRAM Augsburg, Germany) which generates a continuous spectrum of light that ranges from the central ultraviolet through the visible and into the infrared wavelength regions (ca. 300–2000 nm). A cut-off filter of λ >350 nm was used in order to ensure that the light was only absorbed by the photosensitizer. The light was passed through a water filter and focused on the reaction vessel (either a hermetically sealed reaction cell, with an oxygen electrode or a 1 × 1-cm spectrophotometric cuvette) containing the continuously stirred solutions.
The experiments of oxygen uptake by 1.0 mM PA were determined with the specific oxygen electrode Orion 97-08.
The Rf-photosensitized rates of oxygen consumption were determined by evaluation of the initial slopes of oxygen uptake vs. irradiation time. In order to investigate the eventual involvement of ROS, experiments of oxygen uptake in the absence and in the presence of different additives with ROS-scavenging capacity were carried out. The following compounds were employed as ROS scavengers: 10.0 mM NaN3 for O2(1Δg); 1 mg/100 ml SOD for O2•−; 1 mg/100 ml CAT for H2O2 and 10.0 mM NaBz for HO• (or 5.0 mM d-mannitol).
The rate constant of the chemical reaction of PA with O2(1Δg) (reaction [Citation11], Scheme ) was determined in MeOH by means of the method introduced by Foote and Ching.Citation12 The synthetic dye-photosensitizer RB was used as O2(1Δg)-generator.Citation13 Assuming that the reaction of O2(1Δg) with the substrate is the only way of oxygen consumption, the ratio of the slope of the first-order plot for substrate consumption, measured by the initial decrease in the respective absorption spectra of the PA and a reference compound (with a krRef value known) upon photosensitization, both at identical concentrations, is equal to the ratio kr/krRef.
Scheme 2. Possible reaction pathways in the sensitized photoirradiation of propyl gallate and t-butylhydroquinone, and reactions of ROS with specific auxiliary quenchers. S represents the photosensitizer and PA the phenolic antioxidants.
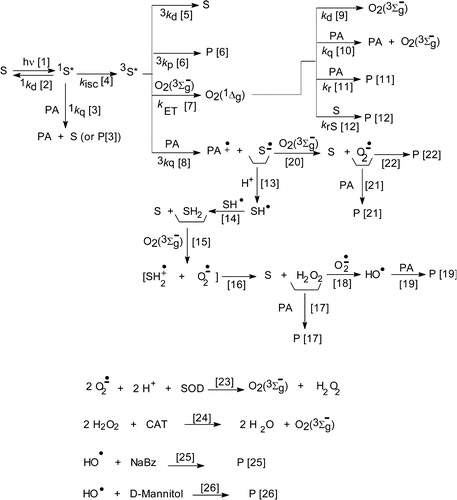
Ground state absorption spectra were registered in a Hewlett Packard 8452A diode array spectrophotometer. In all cases, quartz cells of 1.0-cm path-length were used.
All rate constant values were determined by triplicate. The reported values in are the mean value and the error bars correspond to the extreme values obtained.
Table 1. Relative rates of oxygen photoconsumption by propyl gallate and t-butylhydroquinone sensitized by riboflavin (V−ox(rel); rate constants for the overall (kt) and reactive (kr) quenching of singlet oxygen; kr/kt ratios; rate constants for the quenching of electronically excited singlet state of riboflavin (1kq); rate constants for the quenching of electronically excited triplet state of riboflavin (3kq); slope of oxygen uptake sensitized by riboflavin in the absence (slope0) and in the presence (slopex) of different scavengers (X = NaN3, SOD, CAT, or NaBz). Solvent: methanol.
Time-resolved O2(1Δg) phosphorescence detection
The laser-kinetic spectrophotometer for time resolved O2(1Δg) phosphorescence detection (TRPD) has been previously described.Citation14 In brief, it consisted of a Nd:YAG laser (Spectron Laser System Ltd, Rugby, UK) as the excitation source. The output at 532 nm was used to excite the phtosensitiser RB. The emitted radiation (mainly 1270 nm) was detected at right angles using an amplified Judson J16/8Sp germanium detector after passing through appropriate filters. The output of the detector was coupled to a digital oscilloscope and to a personal computer for processing the signal. Sixteen shots were usually averaged in order to get a good signal-to-noise ratio, from which the decay times were determined. Solution of RB with absorbance at the laser wavelength of ca. 0.2 was used. The decay kinetics was first order in all cases. The experiments were made in MeOD instead of MeOH, due to the enlargement of the O2(1Δg) lifetime in this solvent, as already discussed elsewhere.Citation15 O2(1Δg) lifetime was evaluated in the absence (τ0) and in the presence (τ) of PA and the ratio τ0/τ was plotted as a function of the PA concentration, according to a simple Stern-Volmer treatment, using equation (1):1 where kt (addition kq + kr, processes [Citation10] and [Citation11], respectively, in Scheme ) is the overall rate constant for O2(1Δg) deactivation.
Stationary and time-resolved fluorescence experiments
For the stationary Rf fluorescence experiments, a Spex Fluoromax spectrofluorometer was used.
Fluorescence lifetimes were measured using a time-correlated single photon counting technique (SPC) on an Edinburgh FL-9000CD instrument (Edinburgh Instruments Ltd, Edinburgh, UK).
The excitation and emission wavelengths were 445 and 518 nm, respectively. All the measures were made at 25 ± 1°C in air equilibrated solutions of MeOH. Quartz cells of 1.0-cm path-length were used.
In order to determine the values of Citation1kq (process [Citation3] in Scheme ), as before, a classical Stern–Volmer treatment of the data was applied through equation (2) where, Citation1τ0 and Citation1τ are the fluorescence lifetimes in the absence and in the presence of different concentrations of PA, respectively.2
Laser flash photolysis experiments
Nitrogen-saturated Rf MeOH solutions were irradiated using a laser flash photolysis apparatus with the frequency-doubled output of a Nd:YAG laser (Spectron) at 355 nm as excitation wavelength, using a 150-W xenon lamp as analyzing light.
The detection system comprised a PTI monochromator and a red-extended photomultiplier (Hamamatsu R666, Hamamatsu Photonics, Hamamatsu, Japan). The signal, acquired and averaged by a digital oscilloscope (Hewlett-Packard 54504A, Agilent Technologies, Santa Clara, USA), was transferred to a PC via a Hewlett-Packard Interface Bus (HPIB) parallel interface, where it was analyzed and stored.
Solutions of Rf with absorbance ca. 0.2 at excitation wavelength were used.
A Stern–Volmer treatment was applied (equation (3)) in order to determine the values of Citation3kq (process [Citation8], in Scheme ).3 where Citation3τ0 and Citation3τ are the respective lifetimes of electronically excited triplet state of Rf in the absence and in the presence of PA.
Test of H2O2-mediated oxidation
The thermal reaction between PA and 20.0 mM H2O2 was evaluated monitoring the respective fluorescence spectra of the antioxidants in MeOH, after and before addition of the peroxide. The respective excitation and emission wavelengths were 280 and 370 nm for 0.05 mM PG, and 290 nm and 325 nm for 0.03 TBHQ.
Results
Photodegradation kinetics
The photoirradiation of the system 0.05–0.2 mM PA/0.02 mM Rf in air saturated MeOH solutions, produces changes in the PA absorption spectra and also in the absorption bands of the photosensitizer (, main and inset A). In parallel, oxygen consumption by PA was observed, but not in the dark or in the absence of PA within of the irradiation times used in these experiments.
Figure 1. Spectral changes of ca. 0.2 mM t-butylhydroquinone + 0.02 mM riboflavin vs. 0.02 mM riboflavin upon visible-light photoirradiation. Inset A: Spectral changes of 0.1 mM propyl gallate + 0.02 mM riboflavin vs. 0.02 mM riboflavin upon visible-light photoirradiation. Numbers on the spectra represent irradiation time, in minutes. Inset B: Spectral changes in a N2-saturated solution of 0.02 mM riboflavin upon visible-light photoirradiation; a, without additives and non-irradiated; b, without additives, 25 minutes irradiation; c, in the presence of 0.15 mM t-butylhydroquinone, 25 minutes irradiation. Solvent: MeOH.
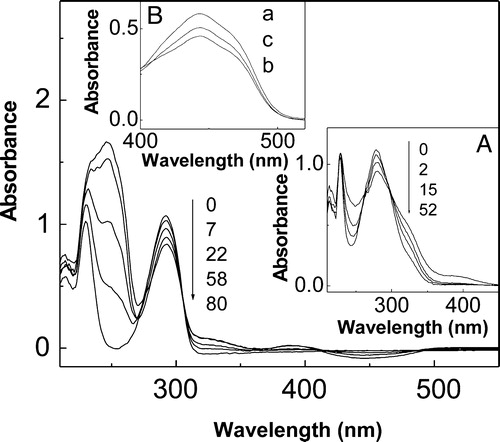
It is known that the anaerobic photodegradation of Rf under visible light irradiation predominantly proceeds through the electronically excited triplet state,Citation8,Citation16 and the rate of the process can be estimated by absorption spectroscopy. Comparative irradiations of N2-saturated MeOH solutions of 0.02 mM Rf in the absence and in the presence of PA demonstrated that this rate decreases in the presence of PA (ca. 0.15 mM) (, inset B).
Results strongly suggest that under visible light irradiation the interaction of Rf–PA could include the participation of electronically excited states of the dye and/or ROS formed from these states. In order to elucidate kinetic and mechanistic aspects of the sensitized-photooxidation of PA a systematic study was developed.
Participation of ROS in the sensitized-photodegradation of PA
Singlet oxygen
The rate of oxygen consumption of the individual PA/Rf-photomediated systems diminished in the presence of 10.0 mM NaN3 (). This salt is an efficient quencher of O2(1Δg) with reportedCitation17 kt values in water in the range (2–5) × 108/M second. NaN3 competes with PA for the quenching of O2(1Δg), diminishing the rate of oxygen consumed by the reaction of the excited oxygen species with PA. Nevertheless, it is known that the ion quenches the Citation3Rf*Citation18 (no data for the quenching rate constants is available) and that its oxygen-consumption inhibitory effect must be carefully considered. The NaN3 test only suggests the possible presence of O2(1Δg) as an oxidative species.
In order to evaluate the eventual participation of the species O2(1Δg) and to achieve as much information as possible about pure potential interactions of O2(1Δg)–PA, the photosensitizer RB, a profusely used O2(1Δg)-generator,Citation13 was used. The overall and reactive interactions between O2(1Δg) and PA were quantified through the rate constants kt and kr, respectively.
The kt values (addition kq + kr, processes [Citation10] and [Citation11], Scheme ) were determined in MeOD, employing TRPD technique (see Experimental section). They do not depend either on the type of photosensitizer or on potential interactions of the substrate with electronically excited states of the photosensitizer involved in O2(1Δg)-generation. The O2(1Δg) lifetimes were evaluated in the absence and in the presence of PA, and the data plotted as a function of substrate concentration, according to equation (1) (, ).
Figure 2. Stern–Volmer plot for the quenching of O2(1Δg)-phosphorescence emission by PA (a) propyl gallate and (b) t-butylhydroquinone, in MeOD. Inset: first order plots for PA consumption in MeOH of (a) propyl gallate; (b) t-butylhydroquinone; (c) 9,10-dimethylanthracene (reference compound).
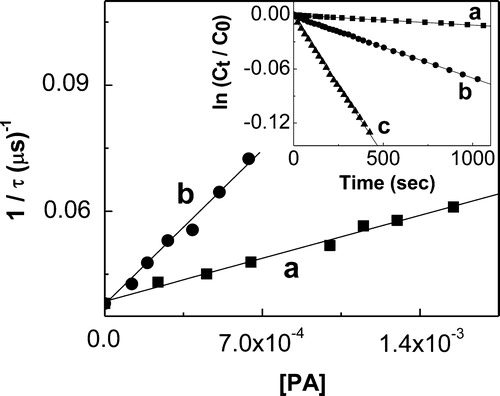
The kr values (process [Citation11]) were determined, in MeOH, from the initial decrease in absorption band at 275 nm for PG, 292 nm for TBHQ, and 355 nm for DMA, upon RB-sensitized photoirradiation. In all cases, conversions lower than 10% were used to avoid possible interference from photoproducts absorption. A first order kinetic treatment of the data of substrate consumption for PG, TBHQ, and DMA was employed to determine the kr values (, inset; ). The hydrocarbon was used as reference compound with krRef = 2.4 107/M second in MeOH.Citation17
Superoxide radical anion, hydrogen peroxide, and hydroxyl radical
Experiments of oxygen uptake upon Rf-photosensitized irradiation in the absence and in the presence of different additives with ROS-scavenging capacity SOD, CAT, and NaBz were carried out. These scavengers have been already used in similar concentrations to confirm/discard the participation of O2•−, H2O2, and HO•, respectively, in a given oxidative process.Citation9,Citation19–Citation26
The rate of oxygen consumption of the individual PA/Rf system was practically the same in the presence of SOD (1 mg/100 ml). The enzyme SOD dismutates the species O2•− (reaction [Citation23], Scheme ).
The participation of H2O2 species in the Rf-sensitized photooxidation of PA is supported by the oxygen-uptake inhibition observed in the presence of CAT ( main and inset A). This enzyme decomposesCitation9 H2O2 as shown in reaction [Citation24], Scheme .
Figure 3. Profiles of oxygen consumption, in MeOH solutions, upon riboflavin-sensitized photoirradiation (a) 0.02 mM Riboflavin + 1.0 mM propyl gallate; (b) 0.02 mM riboflavin + 1.0 mM propyl gallate + 1 mg/100 ml catalase. Inset A: Profiles of oxygen consumption, in MeOH, upon riboflavin-sensitized photoirradiation (a) 0.02 mM riboflavin + 1.0 mM t-butylhydroquinone; (b) 0.02 mM Riboflavin + 1.0 mM t-butylhydroquinone + 1 mg/100 ml catalase. Inset B: Profile of 0.03 mM t-butylhydroquinone consumption, monitored through the decay of stationary fluorescence intensity at 325 nm in the presence of 20.0 mM H2O2 as a function of time, in MeOH.
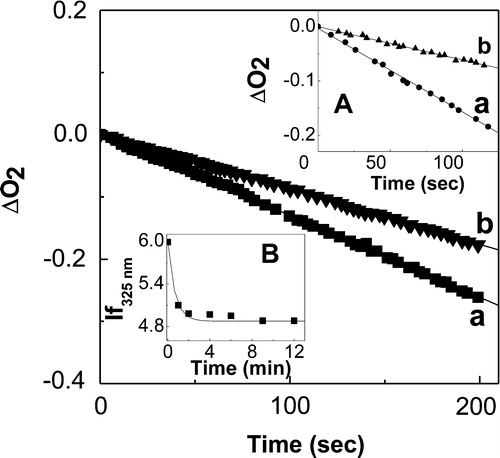
We independently tested the thermal reaction between 0.05 mM PG, 0.03 mM TBHQ, and 20.0 mM H2O2, in MeOH solution. A decrease in the PA fluorescence intensity upon addition of the peroxide indicates the occurrence of chemical transformations in the antioxidants (, inset B).
The behavior of the profile for oxygen uptake by PG and TBHQ upon Rf-sensitized in the presence of NaBz is somewhat different. In the case of PG, the addition of 10.0 mM salt did not produce any change in the rate of oxygen consumption. Nevertheless, for TBHQ the rate of oxygen uptake in the presence of the salt was decreased. A similar concentration of NaBz as used in these experiments has been used by other authorsCitation22 for the specific quenching of the species HO•. The results with NaBz were confirmed using d-mannitol as additional scavenger of HO•. d-Mannitol reacts with the species HO• with a second order rate constant of 1.9 109/M second in waterCitation20 (reaction [Citation26]). Similar concentrations of d-mannitol have been previously used as an efficient trappers in HO•-mediated photooxidations.Citation22,Citation24,Citation27
As additional information, and in order to eliminate possible interference of the quenchers in the oxygen uptake experiments, SOD and NaBz were checked to control that they not produced a noticeable oxygen uptake when exposed to light in the presence of 0.02 mM Rf. Besides, both scavengers did not interact with the electronically excited states of the vitamin.Citation27 The last asseveration is also valid for CAT, whereas the enzyme, in the described conditions, produced an oxygen consumption of ca. 10% as compared with the corresponding values for PA.
The values of the relative rates of oxygen uptake in the presence and in the absence of specific quenchers of ROS are shown in . These rates constitute the mean values of three independent runs which do not differ over 3% from each other.
Participation of electronically excited states of Rf in the photodegradation of PA
The former experimental evidence clearly indicates that at least a ROS-mediated mechanism operates in the aerobic sensitized photodegradation of PA. Consequently, the possibility of an additional photodegradation mechanism based on the interaction of PA with electronically excited states of the Rf was investigated.
Quenching of electronically excited singlet state of Rf
The fluorescence properties of Rf are well known.Citation8 In air-equilibrated MeOH solution, Rf shows a fluorescence emission band centered at 518 nm. In the presence of ≥5.0 mM PA, the intensity of the stationary emission of Citation1Rf* (1S*, in Scheme ) decreased, but the shape of the emission spectrum did not change. In parallel, the fluorescence decay of Rf was evaluated in the absence and in the presence of PA by means of time-resolved methods (SPC technique). From the classical Stern–Volmer treatment (Eq. (2)) the rate constant Citation1kq (process [Citation3], Scheme ) was graphically determined (, ). In all cases, the fluorescence decay of Rf in MeOH was monoexponencial with a value Citation1τo of 5.4 ns, in excellent agreement with previous published data.Citation28
Quenching of electronically excited triplet state of Rf
The decay of Citation3Rf* (3S*, in Scheme ) in N2-saturated MeOH solution was determined at low Rf concentration and at laser energy low enough to avoid undesired effects such as self-quenching and triplet–triplet annihilation. The disappearance of Citation3Rf*, generated by a 355-nm laser pulse was monitored from the first-order decay of the absorbance at 670 nm, at wavelength where the interference from other possible species is negligible. Under these conditions, Citation3Rf* lifetime appreciably decreases in the presence of PA.
As before, a Stern–Volmer treatment of the triplet quenching (equation (3)) yielded the bimolecular rate constants Citation3kq, (process [Citation8]) (, inset; ).
Discussion
Scheme shows the set of reactions employed for interpretation and discussion of the results. It depicts a generic photosensitized process, in which the absorption of visible light promotes the dye-photosensitizer (S) to the electronically excited singlet (1S*) and triplet (3S*) states (processes [Citation1] and [Citation4]). Citation3S* can transfer energy to ground state oxygen (O2(3Σg−)) in the aerated solution, generating O2(1Δg) (process [Citation7]). These species can decay by collision with solvent molecules (process [Citation9]), and can interact physically (rate constant kq) and/or chemically (rate constant kr) with the substrate PA (processes [Citation10] and [Citation11], respectively), with overall rate constant kt = kq + kr. Typical electron donors, as phenols,Citation29 can also transfer an electron to Citation3S*, giving rise to the respective semireduced (S•−) and semioxidised (PA•+) forms (process [Citation8]).
The interaction of S•− with O2(3Σg−) could generate the reactive species O2•− through process [Citation20]. In the case of Rf, the neutral radical (SH•) would be formed after the protonation of the species S•− (process [Citation13]). The bimolecular decay of SH• is knownCitation30,Citation31 to proceed through disproportion reaction to yield equimolar S and fully reduced S (SH2) (process [Citation14]), which in the presence of O2(3Σg−) is reoxidised to give initially SH2•+ and O2•− (process [Citation15]) and finally S and H2O2 (process [Citation16]). Under these reaction conditions, H2O2 together with O2•− could give rise to HO• (process [Citation18]). The processes [Citation16] and [Citation20] regenerate ground state S. These processes represent crucial steps in living organisms, for which it is well established that ROS are a key intermediate in the oxygen redox chemistry.Citation32
According to the results herein shown, PA interact with electronically excited singlet and triplet states of Rf, as well as with ROS generated from these excited states.
Although the interaction of PA with electronically excited singlet state of Rf (process [Citation3], Scheme ) occurs with a rate constant close to the diffusion limit, relatively high concentrations of PA, much higher than those employed in the kinetic experiments, are necessary to partially hinder electronically excited triplet state generation. As a consequence, it can be assumed that the population of Citation3Rf* is practically not affected by any quenching effect on Citation1Rf* under our experimental conditions (ca. 1.0 mM PA).
It is currently acceptedCitation33 that, in general, the quenching of Citation3S* by O2(3Σg−) to produce O2(1Δg) occurs with a rate constant kET of 1/9 of the diffusional value. A kinetic analysis of laser flash photolysis data for Rf, considering a rate constant kET ca. 1.2 × 109/M second in MeOHCitation34 and a mean value for Citation3kq of 3.6 × 109/M/second, indicates that the quenching of Citation3Rf* by PA (process [Citation8]) could compete with the generation of O2(1Δg) (process [Citation7]) at concentrations typically employed in this work (ca. 1.0 mM PA and 2.0 mM dissolved O2(3Σg−) in MeOH).Citation35 In other words, both O2(1Δg) and Rf•− are formed from Citation3Rf*. The last species is generated by an electron transfer process from PA toward Citation3Rf*, with the concomitant production of Rf•− and PA•+ (process [Citation8]). From Rf•−, the species RfH• may be formed in the presence of proton-donating substratesCitation29,Citation36,Citation37 (process [Citation13]) and triggers a cascade of photoprocesses that may produce the oxidative species O2•−, H2O2, and/or HO• that in a further step can react with PA or with Rf (Scheme ).
According to Scheme , both RfH• and O2•− are straightforwardly generated from Rf•− (processes [Citation13] and [Citation20], respectively) and could act as precursors of the species H2O2 and HO•. Results show that different ROS participate in the Rf-sensitized photooxidation of PG and TBHQ.
In the case of PG, the observed decrease in the rate of oxygen consumption in the presence of CAT strongly suggests that H2O2 species is formed during the oxidative process and may eventually react with PG. The result obtained from the thermal reaction between PG and H2O2 demonstrates the viability of a H2O2-mediated oxidation of PG. In parallel, the lack of any effects on the oxygen consumption in the presence of SOD and NaBz leads us to discard the participation of O2•− and HO• as oxidative agents under work conditions. However, the species O2•− should be generated because the formation of H2O2 occurs via O2•− (processes [Citation15] and [Citation16]). In this way, the case of TBHQ is somewhat different. The decrease in the rate of oxygen consumption in the presence of CAT or NaBz, accounting for the respective presence of H2O2 and HO•, suggests that both oxidative species may be formed and could react with TBHQ. The presence in the photoreaction of HO• is supported by additional experiments of oxygen consumption carried out in the presence of d-mannitol. Although other radicals such as alkyl-peroxy could also react with HO•, their presence in our system is not evident. Hence, on the basis of the available experimental evidence, the interaction of TBHQ with HO• remains a possibility. The generation of HO• depends on the previous H2O2 and O2•− generation (processes [Citation15] and [Citation16], respectively). The scavenging of either of the two species reduces the rate of oxygen uptake in a HO•-mediated TBHQ oxidation. Following this line, HO• is a final product, so that it is the only species that may be independently and selectively detected by a specific inhibitor like NaBz or d-mannitol. The CAT test effectively recognizes the presence of H2O2 as an initial oxidative species, but does not demonstrate the direct participation of this ROS in the oxidative process. Already described experiments support the occurrence of thermal reaction between TBHQ and H2O2, as shown in , inset B.
In contrast, the observed inhibition of the oxygen uptake runs in the presence of NaN3 and the results mentioned above for the flash photolysis experiments, suggest the participation of O2(1Δg) in the Rf-sensitized photoreaction of both PA. The quenching of O2(1Δg) can occur through collisions with surrounding solvent molecules (process [Citation9]), by interaction with Rf (process [Citation12]) for which a rate constant krRf of 6 × 107/M second has been reported in MeOHCitation38 or by interaction with PA (processes [Citation10] and [Citation11]). The value for overall rate constant (kt = kq + kr) obtained by time-resolved methods and the reactive rate constant (kr) suggest that the photooxidation of PA by O2(1Δg) is possible. According to these rate constant values, we can said that the quenching ability of both PA towards O2(1Δg) is low-to-moderate, in accordance with values reported for different alkyl-substituted phenols.Citation39
The distinction between physical and chemical contributions (kq, process [Citation10] and kr, process [Citation11], respectively) is highly significant because the physical contribution usually is interpreted, in practical terms, as a form of self-protection against O2(1Δg)-mediated photooxidations. Nevertheless, no relevant information about these photoreactions can be obtained from the straightforward analysis of isolated kt, kq, and kr values. A simple and useful approach is the evaluation of the kr/kt ratio, which can be envisaged as the fraction of the overall interaction O2(1Δg)-substrate that leads to effective chemical transformation. Results show that TBHQ has a high component of O2 (1Δg)-physical deactivation and low photodegradation efficiency by the oxidative species. In comparative terms, TBHQ has a higher antioxidant capacity than PG.
With regard to the photooxidation products O2(1Δg) mediated for phenols and polyhydroxybenzenes,Citation39,Citation40 the accepted mechanism of the quenching is the initial formation of an encounter complex [O2(1Δg)-substrate] with partial charge-transfer character, from which an irreversible electron transfer process would yield the products. This primary encounter complex can also give, through a (2 + 4) cycloaddition of O2(1Δg), an unstable endoperoxide and, later, a quinonic compound.Citation39,Citation41,Citation42
Acknowledgements
Financial support from Consejo Nacional de Investigaciones Científicas y Técnicas (CONICET) and Secretaría de Ciencia y Técnica de la Universidad Nacional de Río Cuarto (SECyT-UNRC).
References
- Fasseas MK, Mountzouris KC, Tarantilis PA, Polissou M, Zervas G. Antioxidant activity in meat treated with oregano and sage essential oils. Food Chem 2007;106:1188–94.
- Alamed J, Chaiyasit W, McClements DJ, Decker EA. Relationships between free radical scavenging and antioxidant activity in foods. J Agric Food Chem 2009;57:2969–76.
- Pazos M, Alonso A, Sánchez I, Medina I. Hydroxytyrosol prevents oxidative deterioration in foodstuffs rich in fish lipids. J Agric Food Chem 2008;56:3334–40.
- IARC Monographs on the Evaluation of Carcinogenic Risks to Humans. Some naturally occurring and synthetic food components. Vol. 40 Lyon: IARC Press; 1986. p. 123–206.
- Guan Y, Chu Q, Fu L, Wu T, Ye J. Determination of phenolic antioxidants by micellar electrokinetic capillary chromatography with electrochemical detection. Food Chem 2005;94:157–62.
- Albini A, Fasani E. Drugs. Photochemistry and photostability. Cambridge, UK: The Royal Society of Chemistry; 1998.
- Criado S, Allevi C, Ceballos C, García NA. Visible-light-promoted degradation of the comercial antioxidants butylated hydroxyanisole (BHA) and butylated hydroxytoluene (BHT). A kinetic study. Redox Rep 2007;12:283–8.
- Heelis PF. The photophysical and photochemical properties of flavin (isoalloxazines). Chem Soc Rev 1982;11:15–39.
- Silva E, Ugarte R, Andrade A, Edwards AM. Riboflavin-sensitized photoprocesses of tryptophan. J Photochem Photobiol B: Biol 1994;23:43–8.
- Silva E, Ruckert V, Lissi E, Abuin E. Effects of pH and ionic micelles on the riboflavin-sensitized photoprocesses of tryptophan. J Photochem Photobiol B: Biol 1991;11:57–68.
- Choe E, Huang R, Min DB. Chemical reactions and stability of riboflavin in foods. J Food Sci 2005;70:28–36.
- Foote CS, Ching TY. Chemistry of singlet oxygen XXI. Kinetics of bilirubin photooxygenation. J Am Chem Soc 1975;97:6209–14.
- Neckers DC. Rose Bengal. J Photochem Photobiol A: Chem 1989;47:1–29.
- Criado S, Bertolotti SG, García NA. Kinetic aspects of the Rose Bengal sensitized photooxygenation of tryptophan alkyl esters. Ground state and photopromoted dye-tryptophan derivative interactions. J Photochem Photobiol B: Biol 1996;34:79–86.
- Nonell S, Moncayo L, Trull F, et al. Solvent influence on the photodynamic degradation of Trolox, a water-soluble model compound for vitamin E. J Photochem Photobiol B: Biol 1995;29:157–62.
- Fritz BJ, Matsui K, Kasai S, Yoshimura A. Triplet lifetime of some flavins. Photochem Photobiol 1987;45:539–41.
- Wilkinson F, Helman W, Ross AB. Rate constants for the decay of the lowest electronically excited singlet state molecular oxygen in solution. An expanded and revised compilation. J Phys Chem Ref Data 1995;24:663–1021.
- Lu C, Lin W, Wang W, Han Z, Yao S, Lin N. Riboflavin (VB2) photosensitized oxidation of 2′-deoxyguanosine-5′-monophosphate (dGMP) in aqueous solution: a transient intermediates study. Phys Chem Chem Phys 2000;2:329–34.
- Baxter RM, Carey JH. Evidence for photochemical generation of superoxide ion in humic waters. Nature 1983;306:575–6.
- Zang LY, Misra HP. Superoxide radical production during the autoxidation of 1-methyl-4-phenyl-2,3-dihydroxypyridinium perchlorate. J Biol Chem 1992;267:17547–52.
- Iuliano L, Pratico D, Ghiselli A, Bonavita MS, Violi F. Reaction of dipyridamole with hydroxyl radical. Lipids 1992;27:349–53.
- Tratniek PG, Hoignè J. Oxidation of substituted phenols in the environment: a QSAR analysis of rate constants for reaction with singlet oxygen. Environ Sci Technol 1991;25:1596–604.
- Silva E, Herrera L, Edwards AM, De la Fuente J, Lissi E. Enhancement of riboflavin-mediated photo-oxidation of glucose 6-phosphate dehydrogenase by uronic acid. Photochem Photobiol 2005;81:206–11.
- Silva E, Edwards AM, Pacheco D. Visible light-induced photooxidation of glucose sensitized by riboflavin. J Nutr Biochem 1999;10:181–5.
- Cohen G, Heikkila RE. The generation of hydrogen peroxide, superoxide radical and hydroxyl radical by 6-hydroxydopamine, dialuric acid and related cytotoxic agents. J Biol Chem 1974;249:2447–53.
- Frati E, Khatib AM, Front P, Panasyuk A, Aprile F, Mitrovic RD. Degradation of hyaluronic acid by photosensitized riboflavin in vitro. Modulation of the effect by transition metals, radicals quenchers and metal chelators. Free Radical Bio Med 1997;22:1139–44.
- Criado S, García NA. A Comparative kinetic and mechanistic study between Tetrahydrozoline and Naphazoline toward photogenerated reactive oxygen species. Photochem Photobiol 2010;86:23–30.
- Bertolotti SG, Previtali CM, Rufs AM, Encinas MV. Riboflavin/triethanolamine as photoinitiator system of vinyl polymerization. A mechanistic study by laser flash photolysis. Macromolecules 1999;32:2920–4.
- Haggi E, Bertolotti S, Miskoski S, Amat-Guerri F, García NA. Environmental photodegradation of pyrimidine fungicides. Kinetics of the visible-light-promoted interactions between riboflavin and 2-amino-4-hydroxy-6-methylpyrimidine. Can J Chem 2002;80:62–7.
- Lu C, Bucher G, Sander W. Photoinduced interaction between oxidized and reduced lipoic acid and riboflavin (vitamin B2). Phys Chem Phys 2004;5:47–56.
- Lu CY, Wang WF, Lin WZ, Han ZH, Yao SD, Lin NY. Generation and photosensitization properties of the oxidized radicals of riboflavin: a laser flash photolysis study. J Photochem Photobiol B: Biol 1999;52:111–6.
- Kanofsky JR. Singlet oxygen production from the reaction of superoxide ion in aprotic solvents: implications for hydrophobic biochemistry. Free Rad Res Commun 1991;87:12–3.
- Koizumi M, Kato S, Mataga N, Matsuura T, Isui I. Photosensitized reactions. Kyoto: Kagakudogin; 1978.
- Calvert J, Pitts J. Photochemistry. New York: John Wiley & Sons; 1966.
- Murov SL. Handbook of photochemistry. New York: M. Decker; 1973. p. 89.
- Land EJ, Swallow AJ. One-electron reactions in biochemical systems as studied by pulse radiolysis. II Riboflavin. Biochemistry 1969;8:2117–25.
- Pajares A, Gianotti J, Stettler G, Bertolotti SG, Criado S, Posadaz A, et al. Modelling the natural photodegradation of water contaminants: a kinetic study on the light-induced interactions between riboflavin and 4-hydroxypyridine. J Photochem Photobiol A: Chem 2001;139:199–204.
- Chacon JN, McLearie J, Sinclair RS. Singlet oxygen yields and radical contributions in the dye-sensitised photooxidation in methanol of esters of polyunsaturated fatty acids (oleic, linoleic, linolenic and arachidonic). Photochem Photobiol 1988;47:647–56.
- García NA. Singlet molecular oxygen mediated photodegradation of aquatic phenolic pollutants. A kinetic and mechanistic overview. J. Photochem Photobiol B: Biol 1994;22:185–96.
- Miskoski S, Soltermann AT, Molina PG, Günther GL, Zanocco A, García NA. Sensitized photooxidation of thyroidal hormones. Evidence for heavy atom effect on singlet molecular oxygen [O2(1Δg)]-mediated photoreactions. Photochem Photobiol 2005;81:325–32.
- Thomas MJ, Foote CS. Chemistry of singlet oxygen XXVI. Photooxygenations of phenols. Photochem Photobiol 1978;27:683–93.
- Wright A, Bubb WA, Hawkins CL, Davies M. Singlet oxygen-mediated protein oxidation: evidence for the formation of reactive side chain peroxides on tyrosine residues. Photochem Photobiol 2002;76:35–46.