Abstract
Andrographis paniculata (hempedu bumi) is a plant that possesses many medicinal values in treating several diseases and for health care maintenance. However, its hepatoprotective activity and mechanism of action have not been fully investigated. Therefore, this study aimed to evaluate the hepatoprotective effects of A. paniculata and its mechanism of action in rats. Carbon tetrachloride (CCl4) challenge of rats at a dose of 1.2 ml/kg body weight-induced oxidative stress in the liver. This was evidenced by augmentation in lipid peroxidation, which was accompanied by a decrease in the activities of antioxidant enzymes and depletion in the level of reduced glutathione (P < 0.05). Parrallel to these changes, CCl4 challenge too, enhanced hepatic damage as evidenced by sharp increase in serum transaminases (e.g. alanine aminotransferase, aspartate aminotransferase, and lactate dehydrogenase) (P < 0.05). Additionally, the impairment of liver function corresponded to histolopathological changes. However, most of these changes were reversed in a dose-dependent fashion by pre-treatment of animals with A. paniculata (P < 0.05). The ability of A. paniculata to scavenge the 2,2-Diphenyl-2-picrylhydrazyl radical was determined through its EC50 value. The EC50 value of A. paniculata was 583.60 ± 4.25 µg/ml. In addition, A. paniculata was found to contain 65.37 ± 1.20 mg/g total phenolics expressed as gallic acid equivalent. From these studies, it is concluded that A. paniculata could be used as a hepatoprotective agent and possesses the potential to treat or prevent degenerative diseases where oxidative stress is implicated.
Introduction
Liver is the major organ responsible for detoxification and deposition of endogenous and exogenous substances. Hence, it is susceptible to diseases, such as hepatitis, cirrhosis, alcohol-related disorders, and cancer. Some of these diseases are caused by exposure of liver to environmental pollutants and xenobiotics, e.g. paracetamol, carbon tetrachloride (CCl4), thioacetamide, alcohol, etc. These toxicants cause damage to the liver by generating reactive oxygen species (ROS).Citation1,Citation2 ROS such as O•−2, H2O2, and •OH are continuously generated in the human body as a consequence of exposure to a plethora of exogenous chemicals from the environment and/or a number of endogenous metabolic processes involving redox enzymes and bioenergetic electron transfer.Citation3 Under normal circumstances, the ROS generated are detoxified by antioxidants present in the body and there is equilibrium between the ROS generated and the antioxidants present. Detrimental effects caused by ROS occur as a consequence of an imbalance between the formation and inactivation of these species. However, owing to ROS overproduction and/or inadequate antioxidant defense, this equilibrium is hampered favoring the ROS upsurge that culminates in oxidative stress.Citation4 ROS readily attack and induce oxidative damage to various biomolecules including proteins, lipids, mitochondria, lipoproteins, and DNA.Citation5 This oxidative damage is a crucial aetiological factor implicated in several chronic human diseases such as diabetes mellitus, liver cirrhosis, cancer, atherosclerosis, arthritis, neurodegenerative diseases, and also in the aging process.Citation6 A potent scavenger of these ROS may serve as a possible preventive intervention for free radical associated diseases such as cancer, diabetes, and others.Citation7
CCl4-induced liver damage has been extensively studied and used widely as a model for screening hepatoprotective agents. It is well known that CCl4 is metabolized in the liver to highly reactive trichloromethyl radical, which initiates free radical-mediated lipid peroxidation (LPO) of cytoplasmic membrane phospholipids. This causes functional and morphological changes in the cell membrane leading to accumulation of lipid-derived oxidant, and thus, cause the liver injury.Citation8 Due to the fact that damaging effects of CCl4 are oxidative stress mediated, numerous investigations have been carried out on hepatoprotective effects of antioxidative compounds.Citation9,Citation10 Therefore, in recent years, considerable attention has been directed toward identification of plants with antioxidant and hepatoprotective activity that may be used for human consumption.
Andrographis paniculata (hempedu bumi) from the family of Acanthaceae is known as ‘King of the Bitters’. It is a plant, which possesses many medicinal values in treating several diseases and for health-care maintenance.Citation11–Citation19 It is used in folk medicine for treatment of infectious fever-causing diseases, colic pain, and liver disease.Citation11–Citation13 Many studies have been carried out to investigate the biological and pharmacological effects of A. paniculata such as antimicrobial,Citation14 antifilarial,Citation15 antiangiogenic,Citation16 antidiabetic,Citation17,Citation18 and antioxidant.Citation11,Citation12,Citation19 The active compounds of A. paniculata contain diterpene lactones, known as andrographolides. It is isolated from the aerial part of the plant.Citation11,Citation12 However, hepatoprotective activity of A. paniculata and its mechanism of action have not been fully investigated so far. Therefore, this study aimed to evaluate the hepatoprotective effects of A. paniculata and its mechanism of action. Since A. paniculata, has been shown to inhibit various kinds of injuries and neoplasm, particularly those mediated through the generation of ROS, it is possible that treatment of animals with A. paniculata may suppress CCl4-mediated oxidative damage. We report herein the in vivo protective effects of A. paniculata against CCl4-induced oxidative tissue damage in rats.
Materials and methods
Chemicals
2,2-Diphenyl-2-picrylhydrazyl (DPPH), l-ascorbic acid, gallic acid, Folin-ciocalteu, ethylenediamine tetraacetic acid (EDTA), trichloroacetic acid, thiobarbituric acid (TBA), Tris HCl, Tween 20, 2,6-dichloroindophenol, flavin adenine dinucleotide, 1-chloro-2,4-dinitrobenzene, 5,5′-dithio-bis-2-nitrobenzoic acid, glutathione reductase, glutathione oxidized, glutathione reduced, beta-nicotinamide adenine dinucleotide phosphate-reduced tetrasodium salt (NADPH), sodium pyruvate, bicinchoninic acid, glycyl glycine, l-gamma-glutamyl-p-nitroanilide, beta-nicotinamide adenine dinucleotide phosphate hydrate (NADP), glucose-6-phosphate, protein assay kit (BCA1), dimethyl sulfoxide (DMSO), and CCl4 were purchased from either Sigma Chemical Company, USA or Aldrich, USA. All other chemicals and solvents used were of analytical grade.
Plant material
Plant material (A. paniculata) was purchased from Papar, Sabah. The plant was identified and authenticated by Mr Jonny Gisil (botanist) from Institute for Tropical Biology and Conservation, University Malaysia Sabah.
Preparation of plant extract
The aerial parts of A. paniculata were washed with tap water and then oven dried at 35–40°C. The dried plants were ground in a blender to obtain the fine powder. Five grams of dried powders were extracted with 100 ml of 80% ethanol at 40°C for 4 hours. The material obtained was centrifuged and filtered through Whatman paper No. 1. The filtrate was then rotary evaporated using Rotavapour® and then lyophilized to yield crude extract (18.63 ± 0.85%). The lyophilized powder was stored in a covered bottle at −80°C until use.
Determination of the total phenolic content
Total phenolic content was determined by the Folin–Ciocalteau method.Citation20 The mixture contained 1.5 ml of Folin-ciocalteau's reagent (1:10) and 200 µl of plant extract. The mixture was mixed and allowed to stand for 5 minutes at room temperature. Then, 1.5 ml of sodium carbonate (60 g/l) was added to the mixture. After incubation in dark condition for 90 minutes at room temperature, the absorbance was measured at 725 nm against the blank using a spectrophotometer (model 4001/4). Gallic acid was used as a standard for determining the phenol content by the Folin–Ciocalteau method. The results were reported in gallic acid equivalents (GAE) per gram of sample. The calibration equation for gallic acid was y = 0.0059x + 0.0043 (RCitation2 = 0.9998), where y is the absorbance and x is the concentration of gallic acid in μg/ml. All the measurements were taken in triplicate and means and standard deviation values were calculated.
Determination of DPPH scavenging assay
The capacity to scavenge the ‘stable’ free radical DPPH was monitored.Citation21 Briefly, all the extracts were dissolved in DMSO at a concentration of 5 mg/ml. Various concentrations of plant extracts (0.3 ml) were mixed with 2.7 ml of ethanolic solution containing DPPH radicals (6 × 10−5 mol/l). The mixture was shaken vigorously and left to stand for 60 minutes in the dark. The reduction of the DPPH radical was determined by measuring the absorption at 517 nm using a spectrophotometer (model 4001/4). The radical scavenging activity (RSA) was calculated as a percentage of DPPH discoloration using the equation: % RSA = ((Acontrol × Asample)/Acontrol) × 100, where Acontrol is the absorbance of the solution without the extract and Asample is the absorbance of the solution containing extract with different concentrations. The extract concentration giving 50% inhibition (EC50) was calculated from the graph of RSA percentage against extract concentration. Ascorbic acid was used as standard and triplicates were performed.
Animals
Animal experiments were performed under standard ethical principles as per the university regulations and of the Federal laws for experiment on animals. Adult Sprague–Dawley male rats (8–12 weeks old), weighing 120–150 g were purchased from the Animal house facility of Health Campus, Universiti Sains Malaysia. The animals were kept in the room (25 ± 2°C) under 12-hour dark/light cycle and acclimatized for a week prior to use. Food and water were available ad libitum. The freeze dried extract was dissolved in distilled water daily prior to oral (gavage) administration.
Experimental protocol
For studying, the effect of A. paniculata on CCl4-mediated hepatic oxidative stress, toxicity, antioxidant enzymes, and histopathological studies, 30 adult male Sprague–Dawley rats (8–12 weeks), weighing 120–150 g, were taken and divided randomly into five groups having 6 rats in each group and treated as follows:
1. | Group I: received saline. | ||||
2. | Group II: CCl4 (1.2 ml/kg body weight (b.w.) by mouth (p.o.)) on 13th and 14th days. | ||||
3. | Group III: A. paniculata (100 mg/kg b.w. p.o.) for 14 days + CCl4 (1.2 ml/kg b.w. p.o.) on 13th and 14th days. | ||||
4. | Group IV: A. paniculata (200 mg/kg b.w. p.o.) for 14 days + CCl4 (1.2 ml/kg b.w. p.o.) on 13th and 14th days. | ||||
5. | Group V: A. paniculata (300 mg/kg b.w. p.o.) for 14 days + CCl4 (1.2 ml/kg b.w. p.o.) on 13th and 14th days. |
Determination of serum biochemistry
Blood was collected from the posterior vena cava with a 23-gauge needle into plain tubes. The samples were centrifuged at 3000 rpm for 10 minutes to obtain the serum. The sera were stored in a −80°C freezer until further analysis. The absolute and relative weights (organ-to-body weight ratio) of the liver were measured. Enzyme activities of alanine aminotransferase (ALT) and aspartate aminotransferase (AST) were analyzed by Reflotron® system (Basel, Switzerland). Lactate dehydrogenase (LDH) activity was measured by monitoring the rate of oxidation of NADH due to the reduction of pyruvate to lactate by enzyme.Citation22 The reaction mixture contained 0.05 ml serum, 2.75 ml phosphate buffer (0.1 M, pH 7.4), 0.1 ml sodium pyruvate (0.01 M), and 0.1 ml NADH (0.002 M). The decrease in absorbance was recorded at 340 nm for 3 minutes at 30 seconds interval. The result was expressed as nmol NADH oxidized/minute/mg protein using molar extinction coefficient of 6.22 × 103/M/cm.
Preparation of post-mitochondrial supernatant
The standard procedure of Mohandas et al.Citation23 was adopted for the preparation of tissue fractionations for all biochemical estimations. Liver tissues were quickly removed, cleaned free of extraneous material, and perfused immediately with ice-cold saline (0.85% w/v, sodium chloride). Liver tissues were then homogenized in chilled phosphate buffer (0.1 M, pH 7.4) containing KCl (1.17% w/v) by using a homogenizer (Polytron PT 1200E, Switzerland). The homogenate was centrifuged at 3000 rpm for 20 minutes at 4°C in a refrigerated centrifuge (5804 R, Eppendorf, Germany) to separate the nuclear debris. The aliquot so obtained was centrifuged at 10 000 rpm for 30 minutes at 4°C to obtain post-mitochondrial supernatant (PMS) which was used for the determination of malondialdehyde (MDA) and reduced glutathione (GSH) content as well as a source of antioxidant enzymes.
Determination of LPO
LPO in PMS was done following the method of Buege and AustCitation24 with some modification, by measuring the rate of production of thiobarbituric acid-reacting substance (TBARS) expressed as MDA equivalents. PMS 1.0 ml (10% w/v) was mixed with 0.5 volume of trichloroacetic acid (10% w/v) and centrifuged at 10 000 rpm for 30 minutes at 4°C. The 1.0 ml of aliquot (supernatant) was mixed with 2.0 ml TBA (0.67% w/v), prepared by dissolving 0.67 g of TBA in warm distilled water. All the tubes were placed in a boiling water bath for a period of 30 minutes. At the end, the tubes were cooled in an ice bath. The absorbance was measured at 535 nm using a spectrophotometer (model 4001/4). The result was expressed as nmol MDA formed/g tissue by using the molar extinction coefficient of 1.56 × 105/M/cm.
Determination of reduced glutathione
GSH in liver was determined by the method of Jollow et al.Citation25 An aliquot of 1.0 ml of PMS (10% w/v) was precipitated with 1.0 ml of sulfosalicylic acid (4% w/v). The samples were kept at 4°C for at least 1 hour and then centrifuged at 3000 rpm for 30 minutes at 4°C. The assay mixture contained 0.2 ml filtered aliquot, 2.6 ml phosphate buffer (0.1 M, pH 7.4), and 0.2 ml 5,5′-dithio-bis-2-nitrobenzoic (DTNB) acid (4 mg/ml of phosphate buffer 0.1 M, pH 7.4) to make a total volume of 3.0 ml. The yellow color was developed and read immediately at 412 nm with a spectrophotometer. The result was expressed as μmol reduced GSH/g tissue by using the molar extinction coefficient of 13.6 × 10/M/cm.
Determination of catalase activity
Catalase (CAT) activity was assayed by the method of Claiborne.Citation26 The reaction mixture contained 0.01 ml PMS (10% w/v), 0.99 ml phosphate buffer (0.1 M, pH 7.4), and 1.0 ml H2O2 (0.019 M). The decrease in absorbance was recorded at 240 nm at every 30 seconds for 3 minutes. The result was expressed as μmol H2O2 consumed/minute/mg protein by using the molar extinction coefficient of 6.4 × 10/M/cm.
Determination of glutathione peroxidase activity
Glutathione peroxidase (GPX) activity was measured according to the procedure of Mohandas et al.Citation23 The reaction solution contained 0.01 ml PMS (10% w/v), 1.58 ml phosphate buffer (0.1 M, pH 7.4), 0.1 ml EDTA (0.5 mM, pH 8.0), 0.1 ml sodium azide (1.0 mM), 0.1 ml GSH (1.0 mM), 0.1 ml NADPH (0.1 mM), and 0.01 ml hydrogen peroxide (30%) in a total volume of 2.0 ml. The disappearance of NADPH at 340 nm was recorded for 3 minutes at 30 seconds interval. Enzyme activity was calculated as nmol NADPH oxidized/minute/mg protein using the molar extinction coefficient 6.22 × 103/M/cm.
Determination of glutathione reductase activity
Glutathione reductase (GR) activity was measured according to the method of Carlberg and Mannervik.Citation27 The assay mixture consisted of 0.025 ml PMS (10% w/v), 1.725 ml phosphate buffer (0.1 M, pH 7.4), 0.1 ml EDTA (0.5 mM), 0.05 ml oxidized glutathione (1.0 mM), and 0.1 ml NADPH (0.1 mM). The disappearance of NADPH at 340 nm was recorded for 3 minutes at 30 seconds interval using a spectrophotometer (model 4001/4) and calculated as nmol NADPH oxidized/minute/mg protein using the molar extinction coefficient 6.22 × 103/M/cm.
Determination of glutathione S-transferase activity
Glutathione S-transferase (GST) activity was determined by the method of Habig et al.Citation28 using 1-chloro 2,4 dinitrobenzene as a substrate. The reaction mixture contained 0.1 ml PMS (10% w/v), 1.75 ml phosphate buffer (0.1 M, pH 7.4), 0.1 ml GSH (1.0 mM), and 0.05 ml 1-chloro-2,4-dinitrobenzene (CDNB) (1.0 mM) to a total volume of 2.0 ml. The change of the absorbance was recorded at 340 nm for 3 minutes at every 30 seconds intervals. The enzyme activity was calculated as nmol CDNB conjugate formed/minute/mg protein using the molar extinction coefficient of 9.6 × 103/M/cm.
Determination of NAD(P)H:quinone oxidoreductase activity
NAD(P)H:quinone oxidoreductase activity (QR) was measured according by the method of Benson et al.Citation29 as described by Iqbal et al.Citation30 The reaction solution consisted 0.01 ml PMS (10% w/v), 1.1 ml Tris-HCl buffer (25 mM, pH 7.4), 0.7 ml bovine serum albumin (1 mg/ml), 0.02 ml Tween-20 (1% w/v), 0.1 ml FAD (0.15 mM), 0.02 ml NADPH (30 mM), and 0.05 ml 2,6-dichlorophenolindophenol (2.4 mM) in a final volume of 2.0 ml. The disappearance of 2,6-dichlorophenolindophenol was recorded at 600 nm for 3 minutes at 30 seconds intervals. The activity was expressed as nmol 2, 6-dichlorophenolindophenol reduced/minute/mg protein using the molar extinction coefficient of 2.1 × 104/M/cm.
Determination of glucose-6-phosphate dehydrogenase activity
Glucose-6 phosphate dehydrogenase (G6PD) activity was measured by the method of Zaheer et al.Citation31 The total volume of 3.0 ml consisted of 0.1 ml PMS (10% w/v), 0.5 ml Tris-HCl buffer (0.2 M, pH 7.6), 0.05 ml NADP (0.1 mM), 0.05 ml glucose-6-phosphatase (0.8 mM), 0.25 ml MgCl2 (8 mM), and 2.05 ml distilled water. The change of the absorbance was recorded at 340 nm for 3 minutes at 30 seconds intervals. The activity was expressed as nmol NADP reduced/minute/mg protein using the molar extinction coefficient of 6.22 × 103/M/cm.
Determination of gamma-glutamyl transpeptidase activity
Gamma-Glutamyl transpeptidase activity (gamma-GGT) was measured according to the method of Orlowski and Meister.Citation32 In all, 0.2 ml PMS (10% w/v) was mixed with 0.8 ml of substrate mixture consisting of glycyl glycine (40 mM), magnesium chloride (11 mM), and l-gamma-glutamyl-p-nitroanilide (4 mM) in Tris-HCl buffer (185 mM, pH 8.25). The mixture was allowed to stand for 10 minutes at 37°C in water bath. Then, 1.0 ml of trichloro acetic acid (25 % w/v) was added into each tube and subjected to centrifugation at 8000 rpm for 20 minutes. The absorbance was measured at 405 nm by a spectrophotometer (model 4001/4). The activity was expressed as nmol p-nitroanaline released/minute/mg protein using the molar extinction coefficient of 1.74 × 103/M/cm.
Histopathological examination
A portion of the liver was cut and fixed in 10% phosphate buffered formaldehyde solution. After embedding in paraffin wax, thin sections of 4 µm thickness of liver tissue were cut and stained with hematoxylin–eosin (H&E), using standard techniques. The thin sections of liver were made into permanent slides and mounted. The slides were examined under a light microscope with photographic facility and photomicrographs were taken.
Determination of protein
Total protein contents were determined by bicinchoninic acid and protein assay kit (BCA1) using a BSA as standard.
Statistical analysis
The results were expressed as mean ± SD. All statistical comparison was made by means of one-way analysis of variance. The homogeneity of the variance was tested by Levene's test. The data were analyzed by using computer program SPSS (Release 17.0, SPSS). A P value less than 0.05 was considered as significant. The EC50 were determined using non-linear regression analysis of the dose response curve by using Graph Pad Prism 5.
Results
Total phenolic content
The ethanolic extract of A. paniculata was found to contain 65.37 ± 1.20 mg/g total phenolics expressed as gallic acid equivalent (GAE, mg/g of extract).
Effect of A. paniculata on DPPH radical scavenging
Scavenging of DPPH represents the free radical reducing activity of A. paniculata extract based on one-electron reduction. As shown in , dose-dependent response was observed for DPPH RSA of A. paniculata extract with EC50 of 583.6 ± 4.25 µg/ml. The EC50 of ascorbic acid was 10.06 ± 1.42 µg/ml.
Liver index
There were no significant alterations in final body weight and liver index in both CCl4-treated and A. paniculata extract pre-treated rats ().
Table 1. Protective effects of A. paniculata on body and liver index
Effects of A. paniculata on CCl4-induced hepatotoxicity
The effects of pre-treatment of rats with A. paniculata extract on CCl4-induced elevation of serum ALT and AST are shown in . CCl4 induced hepatotoxicity, as evident from the increased level of ALT and AST compared with saline-treated control (P < 0.05). Interestingly, pre-treatment of animals with A. paniculata extract suppressed the level of ALT and AST (P < 0.05) dose dependently. These findings showed that the pre-treatment of animals with A. paniculata extract could effectively suppress the elevated level of ALT and AST in rats intoxicated with CCl4. As shown in , the lactate dehydrogenase (LDH) level was also elevated significantly in the CCl4-treated animals. Pre-treatment of animals with A. paniculata extract at a dose level of 200 mg/kg body weight and 300 mg/kg body weight significantly reduced the level of LDH.
Figure 2. Protective effects of A. paniculata on CCl4 induced hepatotoxicity: serum enzymes (ALT, alanine aminotransferase; AST, aspartate aminotransferase; and LDH, lactate dehydrogenase). Dose regimens and treatment protocols are described in material and method section. Each bar represents the mean ± SD, n = 6; bars with different asterisks differ significantly at P < 0.05 level.
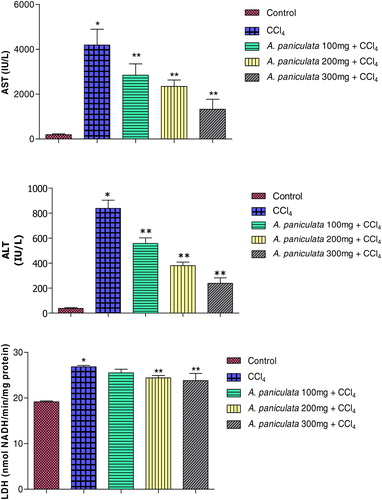
Effects of A. paniculata on LPO and GSH
Dose-dependent effects of A. paniculata extract on CCl4-induced LPO and GSH are shown in . The MDA and GSH levels in PMS were significantly (P < 0.05) altered in the CCl4-treated group. The MDA level was increased in the CCl4 treated group (51%). On the other hand, the pre-treatment of animals with A. paniculata extract at dose level of 100 mg/kg body weight, 200 mg/kg body weight and 300 mg/kg body weight significantly (P < 0.05) decreased the MDA level. In the CCl4-treated group, the GSH level was depleted significantly compared with the saline-treated control group by 36%. Pre-treatment of animals with A. paniculata extract has protected the GSH depletion induced by CCl4.
Table 2. Protective effects of A. paniculata on hepatic glutathione and LPO of rats challenged with CCl4
Effects of A. paniculata on antioxidant enzymes
As shown in , significantly (P < 0.05) lower activities of CAT, GPX, QR, GST, GR, and G6PD enzymes and no changes in gamma-GGT were observed in the liver of CCl4-administrated rats compared to the saline-treated control group. The observed decrease in the activities for CAT, GPX, QR, GST, GR, and G6PD were 30, 37, 49, 13, 40, and 20%, respectively, compared to saline-treated control. Activities of CAT, GPX, QR, GST, GR, and G6PD in liver were significantly restored by pre-treatment of animals with A. paniculata extract in a dose-dependent manner.
Table 3. Protective effects of A. paniculata on hepatic antioxidant profile of rats challenged with CCl4
Effects of A. paniculata on histopathological alterations
Histopathological studies in both CCl4-treated and A. paniculata extract pre-treated rats were performed to ascertain the cause of hepatic cell death and to substantiate the biochemical findings. Our histopathological findings support the biochemical studies. As shown in , the histopathological examination of saline-treated control liver revealed normal cellular architecture with distinct hepatic cells, central vein, and sinusoidal spaces. In contrast, the CCl4-treated group showed degenerative changes such as fatty changes, necrotic cells, cellular hypertrophy, sinusoidal dilatation, and inflammatory cell infiltration. However, most of these changes were alleviated by prophylactic treatment of animals with A. paniculata dose dependently.
Figure 3. Histopathological changes in rat livers (H&E). Dose regimens and treatment protocols are described in material and method section. (A) Control liver; (B) rat liver challenged with CCl4; (C) rat liver challenged with CCl4 and pre-treated with A. paniculata (100 mg/kg b.w.); (D) rat liver challenged with CCl4 and pre-treated with A. paniculata (200 mg/kg b.w.); (E) rat liver challenged with CCl4 and pre-treated with A. paniculata (300 mg/kg b.w.), magnification 100 ×.
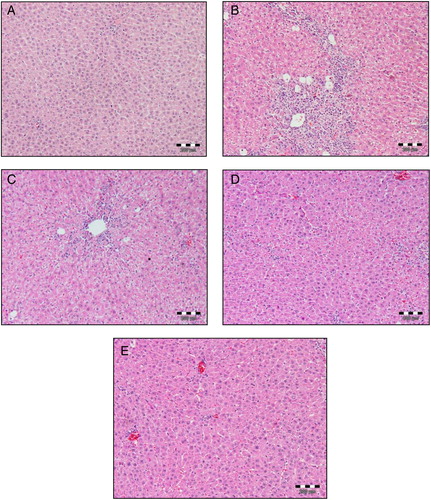
Discussion
Several clinical disorders implicate a deficient natural antioxidant defense as their etiological/ pathological factor.Citation3–Citation7 The onset/progression of these disorders can be held/delayed by supplementing with the antioxidant defense.Citation3–Citation7 Therefore, a lot of scientific research focused on exploring safe and effective antioxidant compounds. Plant extract and plant-derived antioxidant compounds potentiate body's antioxidant defense or act as antioxidant and are antioxidants of choice because of their safety over the synthetic ones.Citation7–Citation10 Thus, a huge body of research is based on discovering plants with antioxidant potential. A. paniculata is a plant that possesses many medicinal values in treating several diseases and for health care maintenance.Citation11–Citation19 In this study, we investigated hepatoprotective effects of A. paniculata and its mechanism of action against CCl4 induced oxidative damage in rats. Results obtained in the present study suggest that the aerial part of A. paniculata has antioxidant activity consistent with results of previous studies.Citation33CitationCitation35 The ability of DPPH free radicals scavenging activity was tested in the present study. The ability of the extract to scavenge these radicals suggests that it contains compounds that are electron donors, which can react with free radical to convert them to a more stable product. Thus, scavenging of DPPH may be related to inhibition of LPO, which is consistent with results of other studies.Citation11,Citation12,Citation19
We further tested the ability of A. paniculata extract to inhibit oxidative stress and allied damage in CCl4-induced hepatotoxicity model. The amounts of endogenous hepatic antioxidants such as GSH and antioxidant enzymes including CAT, GPX, GR, GST, and G6PD as well as MDA levels showed a clear correlation with CCl4-induced hepatotoxicity. A 2-week-long pre-treatment with A. paniculata extract significantly prevented CCl4-induced oxidative stress and also inhibited hepatic injury. CCl4 is a well known xenobiotic-induced hepatotoxicity model.Citation36–Citation45 CCl4 is metabolized in the liver by cytochrome P450 enzymes such as cytochrome CYP2E1, CYP2B2, or CYP2B2 and possible CYP3A, to highly reactive metabolites: trichloromethyl radical (CCl3•) and trichloromethyl peroxyl radical (CCl3OO•). CCl3• can attack nucleic acid, protein and lipid of cell and initiate cellular impairment such as lipid metabolism.Citation8,Citation46 CCl3OO• initiates LPO by attacking the polyunsaturated fatty acids especially those associated with phospholipid. Thus, it affects the permeability of the cell membrane and causes loss of cellular calcium ions and results in cell damage.Citation46 The elevated activity of ALT and AST in serum indicates the cellular leakage and loss of functional integrity of the plasma membrane of hepatocytes.Citation47–Citation49 In the present study, the levels of ALT, AST, and LDH were significantly increased in the CCl4-treated group compared to the control group. Conversely, pre-treatment of animals with A. paniculata extract significantly lowered the levels of ALT, AST, and LDH, which has suggested a significant protection of liver injury.
The elevation of MDA levels, which is one of the end products of peroxidationCitation50 in the liver tissue, and the depletion of hepatic GSH levels are important indicators of oxidative stress in CCl4-treated rats.Citation51 In the current study, hepatic MDA was significantly increased in the CCl4-treated group. Interestingly, pre-treatment of rats with A. paniculata (repeated dose) prior to CCl4 treatment significantly reduced the extent of LPO caused by CCl4. This inhibition in LPO could be one of the protective mechanisms of A. paniculata against the toxicity caused by CCl4. GSH is an important non-enzymatic antioxidant for detoxification of reactive toxic metabolites in the cells and necrosis is initiated when the GSH is depleted.Citation52 Parallel to lipid peroxidation, GSH was significantly depleted in the CCl4-treated group. Animals pre-treated with A. paniculata exhibited significant protection by showing an increased level of GSH. These results are consistent with previous studies which have shown that herbs or purified herbal constituents protect against CCl4 induced hepatotoxicity through the reduction of MDA and elevation of tissue GSH.Citation36–Citation45
Cellular antioxidant enzymes inside the cells are important defense systems for the protection against oxidative stress. CCl4 administration to rats depletes the enzymatic and non-enzymatic antioxidant armoury in the liver including CAT, GR, GST, GPX, G6PD, and GSH levels. These results are consistent with previous observations of others, where CCl4 was shown to deplete hepatic enzymatic and non-enzymatic antioxidant enzymes.Citation36–Citation45 In contrast, multiple dose of pre-treatment of A. paniculata restored the antioxidant enzyme activities in a dose-dependent manner compared to the CCl4-treated group. This clearly suggests a significant restoration of liver injury. However, there were no significant changes in the activity of gamma-GGT in CCl4-treated and A. paniculata pre-treated animals. Similar observations for the activity of gamma-GGT in CCl4-treated animals have also been reported previously by Idéo et al.Citation53
Furthermore, QR is a flavoprotein which utilizes NADH or NADPH as a reducing cofactor and detoxifies the reactive quinones and quinone-imines to less reactive and less toxic hydroquinones.Citation30 As observed in the present study treatment of animals with CCl4 led to depletion of QR activity, which is consistent with the findings of Fukao et al.Citation43 Thus, the inhibition of QR activity might cause the accumulation of toxic quinones in the body which leads to the perpetual generation of oxidative stress. This provides an explanation for the reported increased in LPO of lipid-rich membranes in animals exposed to CCl4.Citation36–Citation45 A similar decrease in the activity of QR has also been noticed in animals treated with Fe-NTA.Citation30 The observed abrogation in the reduction of QR activity in animals pre-treated with A. paniculata provide a further strong and clear indication that this agent imparts its preventive action, a least in part, via its antioxidant property.
Our next studies were directed toward further evaluating the protective effect of A. paniculata against CCl4 induced hepatic histopathological alterations to ascertain the cause of hepatic cell death and to substantiate the biochemical findings. Our histopathological studies support the biochemical findings. In fact, histopathological changes, seen in the liver of rats treated with CCl4 were characterized by massive fatty change, necrosis of hepatocytes, inflammatory infiltration, and sinusoidal dilatation. However, most of these changes were alleviated by prophylactic treatment of animals with A. paniculata. Our results confirmed previous findings of other researchers who had found degenerative changes in liver of rats exposed to CCl4.Citation36–Citation45
Reviewing all the observations, our results indicate that A. paniculata could be used as hepatoprotective agent and possesses potential to be used to treat or prevent degenerative disease where oxidative stress is implicated. The precise mechanism(s) of inhibitory effects of A. paniculata are still incompletely understood; it is likely that antioxidant and free radical scavenging action of A. paniculata, at least in part, may be related to the modulation of CCl4-induced oxidative hepatic damage.
Conflict of interest statement: The authors declare that there are no conflicts of interest.
Acknowledgements
Authors are thankful to Ministry of Higher Education, Government of Malaysia for providing grant-in-aid No.FRG0166-SP-2008 for scientific research to support these studies. Authors are also thankful to Prof. Datin Seri Panglima Dr Ann Anton, Director of Biotechnology Research Institute for her support and encouragement. The authors are thankful to Prof. Dr Amran Ahmed, School of Science and Technology, University Malaysia Sabah for his suggestions and advice for statistical analysis. We also acknowledge Dr Mie Mie Sien, School of Medicine, University Malaysia Sabah for histopathological studies. The authors would also like to express special thanks to Dr Khoo Yong Pheng for the animal care, suggestions, help, and discussion.
References
- Kodavanti PR, Joshi UM, Young YA, Meydrech EF, Mehendale HM. Protection of hepatotoxic and lethal effects of CCl4 by partial hepatectomy. Toxicol Pathol 1989;17:494–505.
- Symth R, Turton JA, Clarke CJ, York MJ, Dare TO, Lane CS, et al. Identification of superoxide dismutase as a potential urinary marker of carbon tetrachloride induced hepatic toxicity. Food Chem Toxicol 2008;46:2972–83.
- Chitra K, Pillai KS. Antioxidants in health. Indian J Physiol Pharmacol 2002;46:1–5.
- Kohen R, Gati I. Skin low molecular weight antioxidants and their role in aging and in oxidative stress. Toxicology 2000;148:149–57.
- Farber JL. Mechanisms of cell injury by activated oxygen species. Environ Health Perspect 2004;102:17–24.
- Gutteridge JMC. Free radicals in disease processes: a complication of cause and consequence. Free Radic Res Commun 1995;19:141–58.
- Iqbal M, Okazaki Y, Okada S. Curcumin attenuates oxidative damage in animals treated with a renal carcinogen, ferric nitrilotriacetate: implications for cancer prevention. Mol Cell Biochem 2009;324:157–64.
- Recknagel RO, Glende EA, Dolak JA, Waller RL. Mechanisms of carbon tetrachloride toxicity. Pharmacol Ther 1989;43:139.
- Ilavarasan R, Vasudevan M, Anbazhagan S, Venkataraman S. Antioxidant activity of Thespesia populnea bark extracts against carbon tetrachloride induced liver injury in rats. J Ethnopharmacol 2003;87:227–30.
- Lee KJ, Jeong HG. Protective effects of Playtycodi radix on carbon tetrachloride induced hepatotoxicity. Food Chem Toxicol 2002;40:517–25.
- Akowuah GA, Zhari I, Mariam A. Analysis of urinary andrographolides and antioxidant status after oral administration of Andrographis paniculata leaf extract in rats. Food Chem Toxicol 2008;46:3616–20.
- Akowuah GA, Zhari I, Mariam A, Yam MF. Absorption of andrographolides from Andrographis paniculata and its effect on CCl4-induced oxidative stress in rats. Food Chem Toxicol 2009;47:2321–6.
- Singha PK, Roy S, Dey S. Protective activity of andrographolide and arabinogalactan proteins from Andrographis paniculata Nees. against ethanol-induced toxicity in mice. J Ethnopharmacol 2007;111:13–21.
- Singha PK, Roy S, Dey S. Antimicrobial activity of Andrographis paniculata. Fitoterapia 2003;74:692–4.
- Zaridah MZ, Idid SZ, Omar AW, Khozirah S. In vitro antifilarial effects of three plant species against adult worms of subperiodic Brugia malayi. J Ethnopharmacol 2001;78:79–84.
- Sheeja K, Guruvayoorappan C, Kuttan G. Antiangiogenic activity of Andrographis paniculata extract and andrographolide. Int Immunopharmacol 2007;7:211–21.
- Hossain MA, Roy BK, Ahmed K, Chowdhury AMS, Rashid MA. Antidiabetic activity of Andrographis paniculata. Dhaka Univ J Pharm Sci 2007;6:15–20.
- Reyes BAS, Bautista ND, Tanquilut NC, Anunciado RV, Leung AB, Sanchez GC, et al. Anti-diabetic potentials of Momordica charantia and Andrographis paniculata and their effects on estrous cyclicity of alloxan-induced diabetic rats. J Ethnopharmacol 2006;105:196–200.
- Neogy S, Das S, Mahapatra SK, Mandal N, Roy S. Amelioratory effect of Andrographis paniculata Nees on liver, kidney, heart, lung and spleen during nicotine induced oxidative stress. Environ Toxicol Pharmacol 2008;25:321–8.
- Velioglu YS, Mazza G, Gao L, Omah BD. Antioxidant activity and total phenolics in selected fruits, vegetables, and grain products. J Agric Food Chem 1998;46:4113–7.
- Hatano T, Kagawa H, Yasuhara T, Okuda T. Two new flavonoids and other constituents in licorice root: their relative astringency and radical scavenging effects. Chem Pharm Bull 1988;36:2090–7.
- Wootton IP. Microanalysis in medical biochemistry. London: J.A.Churchill; 1964. p. 101.
- Mohandas J, Marshall JJ, Duggin GG, Horvath JS, Tiller DJ. Differential distribution of glutathione and glutathione related enzymes in rabbit kidney: possible implications in analgesic neuropathy. Cancer Res 1984;44:5086–91.
- Buege JA, Aust SD. Microsomal lipid peroxidation. In: , Packer L (ed.) Methods in enzymology, vol 52. New Jersey: Academic Press; 1978. p. 302–10.
- Jollow DJ, Mitchell JR, Zampagilone N, et al. Bromobenzene- induced liver necrosis: protective role of glutathione and evidence for 3,4-bromobenzene oxides as a hepatotoxic intermediate. Pharmacology 1974;11:151–69.
- Claiborne A. Catalase activity. In: , Green Wald RA (ed.) CRC handbook of methods for oxygen radical research. Boca Raton: CRC Press; 1985. p. 283–4.
- Carlberg I, Mannervik B. Glutathione reductase levels in rat brain. J Biol Chem 1975;250:5475–80.
- Habig WH, Pabst MJ, Jokoby WB. Glutathione S-transferase: the first enzymatic step in mercapturic acid formation. J Biol Chem 1974;249:7130–9.
- Benson AM, Hunkeler AJ, Talalay P. Increase of NADPH: quinone reductase activity by dietary antioxidants: possible role in protection against carcinogenesis and toxicity. Proc Natl Acad Sci USA 1980;77:5216–20.
- Iqbal M, Sharma SD, Rahman A, Trikha P, Athar M. Evidence that ferric nitrilotriacetate mediates oxidative stress by down-regulating DT-diaphorase activity: implications for carcinogenesis. Cancer Lett 1999;141:151–7.
- Zaheer N, Tiwari KK, Krishnan PS. Exposure and solubilization of hepatic mitochondrial shunt dehydrogenases. Arch Biochem Biophys 1965;109:646–8.
- Orlowski M, Meister A. γ-Glutamyl cyclotransferase distribution, isozymic forms and specificity. J Biol Chem 1973;248:2836–44.
- Barros L, Baptista P, Ferreira ICFR. Effects of Lactarius piperatus fruiting body maturity stage on antioxidant activity measured by several biochemical assays. Food Chem Toxicol 2007;45:1731–7.
- Rafat A, Philip K, Muniandy S. Antioxidant potential and content of phenolic compounds in ethanolic extracts of selected parts of Andrographis paniculata. J Med Plants Res 2010;3:197–202.
- Lin FL, Wu SJ, Lee SC, Ng LT. Antioxidant, antioedema and analgesic activities of Andrographis paniculata extracts and their active constituent andrographolide. Phytother Res 2009;23:958–64.
- Slater TF, Cheeseman KH, Ingold KU. Carbon tetrachloride toxicity as a model for studying free-radical mediated liver injury. Philos Trans R Soc B Biol Sci 1985;311:633–45.
- Jayakumar T, Sakthivel M, Thomas PA, Geraldine P. Pleurotus ostreatus, an oyster mushroom, and decreases the oxidative stress induced by carbon tetrachloride in rat kidneys, heart and brain. Chem-Biol Interact 2008;176:108–20.
- Khan MR, Rizvi W, Khan GN, Khan RA, Shaheen S. Carbon tetrachloride-induced nephrotoxicity in rats: protective role of Digera muricata. J Ethnopharmacol 2009;122:91–9.
- Ogeturk M, Kus I, Colakoglu N, Zararsiz I, Ilhan N, Sarsilmaz M. Caffeic acid phenethyl ester kidneys against carbon tetrachloride toxicity in rats. J Ethnopharmacol 2005;97:273–80.
- Khan MR, Ahmed D. Protective effects of Digera muricata (L.) Mart. on testis against oxidative stress of carbon tetrachloride in rat. Food Chem Toxicol 2009;47:1393–9.
- Singh N, Kamath V, Narasimhamurthy K, Rajini PS. Protective effect of potato peels extract against carbon tetrachloride-induced liver injury in rats. Environmen Toxicol Pharmacol 2008;26:241–6.
- Srivastava A, Shivanandappa T. Hepatoprotective effect of the root extract of Decalepis hamiltonii against carbon tetrachloride-induced oxidative stress in rats. Food Chem 2010;118:411–7.
- Fukao T, Hosono T, Misawa S, Seki T, Ariga T. Chemoprotective effect of diallyl trisulfide from garlic against carbon tetrachloride-induced acute liver injury of rats. Biofactors 2004;21:1–4.
- Kumar SS, Kumar BR, Mohan GK. Hepatoprotective effect of Trichosanthes cucumerina Var cucumerina L. on carbon tetrachloride induced liver damage in rats. J Ethnopharmacol 2009;123:347–50.
- Hwang YP, Choi JH, Jeong HG. Protective effect of the Aralia continentalis root extract against carbon tetrachloride-induced hepatotoxicity in mice. Food Chem Toxicol 2009;47:75–81.
- Weber LWD, Boll M, Stamfl A. Hepatotoxicity and mechanism of action of haloalkanes: carbon tetrachloride as a toxicological model. Crit Rev Toxicol 2003;33:105.
- Chandan BK, Saxena AK, Shukla S, Sharma N, Gupta DK, Suri KA, et al. Hepatoprotective potential of Aloe barbadensis Mill. Against carbon tetrachloride induced hepatotoxicity. J Ethnopharmacol 2007;111:560–6.
- Sreelatha S, Padma PR, Umadevi M. Protective effects of Coriandrum sativum extracts on carbon tetrachloride-induced hepatotoxicity in rats. Food Chem Toxicol 2009;47:702–8.
- Molander DW, Wroblewsk F, La Due JS. Transaminase compared with cholinesterase and alkaline phosphatase an index of hepatocellular integrity. Clin Res Proc 1955;3:20–4.
- Vaca CE, Wilhelm J, Harms-Rihsdahl M. Interaction of lipid peroxidation product with DNA. A review. Mut Res: Rev Genet Toxicol 1988;195:137–49.
- Ohkawa H, Ohishi N, Yagi K. Assay of lipid peroxides in animal tissue by thiobarbituric acid reaction. Anal Biochem 1979;95:351–8.
- Recknagel RO, Glende EA, Britton RS. Free radical damage and lipid peroxidation. In: , Meeks RG (ed.) Hepatotoxicology. Florida: CRC Press; 1991. p. 401–36.
- Idéo G, Morganti A, Dioguardi N. γ-Glutamyl transpeptidase: a clinical and experimental study. Int J Gastroenterol 1972;5:326.