Abstract
Oxidative stress caused by increased production of free radicals and impaired functions of antioxidants remains as the major factor associated with the pathophysiology of many neuropsychiatric diseases.
Objective
The objective of the present study was to analyze the oxidative stress markers in urine sample since the collection of blood from these children is highly meticulous and also to evaluate whether these urinary markers can be correlated with the severity of autism.
Methods
The subjects of the study were 45 autistic children with different grades of severity (low functioning autism (LFA), medium functioning autism (MFA), and high functioning autism (HFA) according to Childhood Autism Rating Scale (CARS), n = 15 children in each group and 50 healthy children (age and sex matched). The boys and girls ratio involved in this study was 4:1, and they were of age 4–12 years. We determined the urinary levels of oxidative stress markers like thiobarbituric acid-reacting substances, lipid hydroperoxides, 4-hydroxy nonenal, protein carbonyls, sulfhydryl groups, total antioxidant capacity, total peroxide content, oxidative stress index, and also UA/Cr ratio in autistic children.
Results
The study observed a significant elevation in the level of oxidative stress markers in autistic children when compared with normal children. The level of antioxidants excreted in urine was found to be significantly low in autistic children. These findings when correlated with the degrees of severity, oxidative stress markers showed positive correlation with increasing order of severity (LFA > MFA > HFA), whereas antioxidants showed negative correlation.
Discussion
The study reveals that the urinary levels of oxidative stress markers can be considered as the measure of oxidative stress index in autistic children. The significant correlation between the severity of autism with urinary lipid peroxidation products also support the use of oxidative stress markers and antioxidants as biomarkers of autism.
Introduction
Autism is a severe developmental disorder in children with poorly understood etiology.Citation1 It is a heterogeneous disorder, both etiologically and phenotypically. Autism is characterized by impairment in social, behavioral, and communicative functions. The condition is highly prevalent, and in recent years there has been an apparent increase in the diagnosis of this disorder.Citation2 While the cause of autism remains elusive, it is considered as a multifactorial disorder that is influenced by genetic, environmental, and immunological factors as well as increased vulnerability to oxidative stress.Citation3 Increasing evidences suggest that oxidative stress plays a pivotal role in the development and clinical manifestation of autism.Citation4,Citation5 In fact, oxidative stress has also been implicated in the pathogenesis of other neuropsychiatric diseases, including schizophreniaCitation6 and major depressive disorder,Citation7 anxiety disorders such as panic disorder,Citation8 and obsessive-compulsive disorder.Citation9
Under normal conditions there is a dynamic equilibrium between the production of reactive oxygen species (ROS) and the antioxidant capacity of a cell.Citation10 ROS includes superoxide (O2.−), hydroxyl, peroxyl, alkoxy, and nitric oxide (NO) free radicals.Citation10 Superoxide is the first reduction product of molecular oxygen, and it is an important source of hydroperoxides and deleterious free radicals.Citation11 Hydrogen peroxide (H2O2) reacts with transition metals such as iron, via the Fenton reaction to produce highly reactive hydroxyl radicals.Citation12 Most of the toxic effects are due to hydroxyl radical formation, which also initiates lipid peroxidation. Lipid peroxidation is a chain reaction between polyunsaturated fatty acids and ROS, that produces alpha, beta-unsaturated aldehydes such as 4-hydroxy nonenal (4-HNE) and malondialdehyde (MDA).Citation13 These aldehydic secondary products of lipid peroxidation are generally accepted as markers of oxidative stress. The reaction of the primary lipid peroxidation products and lipid hydroperoxides (LHP) with proteins is not well characterized. This is presumably because of low stability of LHP, which decomposes rapidly via radical intermediates to aldehydic products.Citation14 It is suggested that the reaction of LHP with protein does not involve alterations of the lipid moiety.Citation15 Nevertheless, no chemical characterization of such products has been carried out. Lipid hydroperoxides are also found to inhibit plasma lecithin: cholesterol acyl transferase.Citation16 The ability of linoleic-13-hydroperoxides to convert bovine serum albumin to carbonyl derivatives in the presence of either Cu2+ or Fe3+ has been demonstrated.Citation17 These lipid peroxidation products are also excreted in urine, where the level of excretion depends on the level produced.
Early studies using ROS generated by ionizing radiation suggested that activated oxygen has the potential to react with functional groups of nearly all amino acids as well as cleaving the polypeptide back bone.Citation18 The most studied types of protein oxidation are those that result in the formation of reactive carbonyl groups (ketones and aldehydes) because these can be tracked most easily experimentally. Carbonyl groups can be the product of a reaction between amino acid side chains (usually Lys, Arg, Pro, or Thr) and hydroxyl radicals. They can also be formed when the products of the reactions between ROS and lipids (4-HNE and MDA) react with amino acid side chains.Citation19 Sulfhydryls are common targets for the free radicals as sulfhydryl oxidation is a key mechanism of free radical-mediated toxicity at the molecular level.Citation20 Antioxidants, on the other hand, counteract the effects of these free radicals and thereby protect cell membranes from lipid peroxidation. The ability of a tissue or fluid to buffer the effects of ROS is called total antioxidant capacity (TAC).Citation21
There is a hypothesis that the imbalance between oxidant and antioxidant systems might be involved in the pathophysiology of autism like other psychiatric diseases such as schizophrenia, bipolar disorders, etc. Hence, the aim of the present study is to determine the levels of thiobarbituric acid-reacting substances (TBARS), 4-HNE, lipid hydroperoxides, protein carbonyls, sulfhydryl groups, uric acid, creatinine, total antioxidant capacity, total peroxides, and oxidative stress index (OSI) in the urine of autistic children in comparison with that of normal children. There are many studiesCitation22 reporting the redox status in the blood of autistic children, but little is known about the urinary content of antioxidants and oxidants. Also, while dealing with autistic children, drawing blood from them may also cause stress on them. This made us prefer urine samples for the study. The study is also focused on evaluating the correlation between the levels of redox compounds excreted and the severity of the disorder.
Subjects and methods
Patients
Autistic children currently attending a special school called Aikya in Chennai, Tamil Nadu, India were the subjects of this study. The institution used check of autism in toddlers to assess autism in children. Autistic children were classified according to the method adopted from Childhood Autism Rating Scale (CARS) as low-functioning autism (LFA), medium-functioning autism (MFA), and high-functioning autism (HFA). Age- and sex-matched healthy normal children served as control subjects. Each group comprised of 15 children and 45 normal children were enrolled in the study for comparison. The boys and girls ratio involved in this study was 4:1, and they were 4–12 years of age. The clinical history is shown in . Children with any special medication for gluten sensitivity or other complications were not included in the study.
Table 1. Clinical history of autistic and normal children taken for the study
CARS classification
This is a 15-item scale which aids in the identification of children with autism and this distinguishes them from other children with compromised development but without autism. Its importance is based on its ability to differentiate mild to moderate from severe autism.Citation23 It is brief and is appropriate for being used in the case of any child over the age of 2 years. It was developed over a 15-year period on the basis of 1500 autistic children. The scale incorporates diagnostic criteria based on the work of many scientists, which was compiled by Schopler et al.Citation24 The scale evaluates behavior in 14 domains that are generally affected in autism, plus a single category for general impression of autism.Citation25 These 15 items are as follows: relating to people, imitation, emotional response, body use, object use, adaptation to change, visual response, listening response, taste, smell, and touch response and use, fear or nervousness, verbal communication, nonverbal communication, activity level, level and consistency of intellectual response, and, finally, general impressions. The scores assigned to each domain vary from 1 (within the limits of normality) to 4 (severe autistic symptoms). The total score varies from 15 to 60 and the cutoff point for autism is 30.Citation24
Collection of urine sample
With the concurrence of parents of the children, urine samples were collected as the first morning void from the autistic and normal children. For the purpose, clean, sterile, and disposable urine collection containers were provided. The samples were stored immediately at 4°C until analysis. All the estimations were done within 2 hours of sample collection. The study protocol has been approved by the Ethical Committee which included a clinician and consent was obtained from the parents of autistic children.
Methods
Lipid peroxides in terms of TBARS and lipid hydroperoxides (LHP) were measured by the method of Draper and HadleyCitation26 and Jiang et al.,Citation27 respectively. 4-HNE was measured by UV spectrophotometric method at 350 nm.Citation28 The protein carbonyl (PC) content was determined by the reaction with 2, 4-dinitrophenyl hydrazine as described by Levine et al.Citation29 Total, protein-bound and non-protein sulfhydryl groups were determined with Ellman's reagent by the method adopted by Sedlak and Lindsay.Citation30 TAC was determined by the method of Miller et al.Citation31 The absorbance at 734 nm and the % of inhibition was calculated and plotted as a function of concentration of antioxidants and of trolox for the standard reference data. Total peroxide concentration was determined using the ‘FOX 2’ methodCitation32 with minor modifications. The ‘FOX 2’ test system is based on oxidation of ferrous ion to ferric ion by various types of peroxides contained within the samples, to produce a colored ferric-xylenol orange complex whose absorbance was measured at 560 nm. The ratio of total peroxides to total antioxidant capacity, the oxidative stress index, was calculated. Uric acid was estimated by the method of Whelton et al.Citation33 Urinary creatinine level was determined by the Jaffe reaction.Citation34 Lowry's methodCitation35 was adopted to determine the concentration of protein.
Statistical analysis
The statistical significance of mean values between different groups was determined by applying Student's t-test and the P value <0.05 was considered as significant. The parameters were also analyzed by means of Spearman rank correlation test and the rs values were calculated to find the correlation.
Results
The study observed a significant increase (P < 0.001) in the level of excretion of lipid peroxides, lipid hydroperoxides, and the protein carbonyls in the urine of all the three groups of autistic children on comparison with age- and sex-matched normal children (). When the levels of excretion of TBARS, LHP, and PC in HFA group children were compared to LFA, the data obtained showed a significant elevation (P < 0.001) in LFA. , shows the level of 4-HNE in the urine of all the three groups of autistic children which is found to be high when compared with that of normal children. The level of protein sulfhydryl, non-protein sulfhydryl, and total sulfhydryl groups were also measured in the urine of autistic children (). It showed a significant decrease when compared with those of normal children. Among the three groups of autistic children, LFA showed much more significant decrease (P < 0.001) in the level of sulfhydryl groups when compared with those of MFA (P < 0.01) and HFA (P < 0.05). When the urinary levels of total antioxidants and total peroxides was measured, the LFA and MFA group of autistic children showed a significant decrease (P < 0.001) when compared with age- and sex-matched normal children. LFA group of autistic children showed a significant decrease in the level of excretion of TAC when compared with HFA and control (). Total peroxides were found to be excreted significantly in all the three groups of autistic children when compared with normal children. Following this, the OSI was calculated. It showed significant increase (P < 0.001) in all the autistic children when compared with normal children. The data obtained in the present study showed that the amount of uric acid excreted by LFA and MFA group of children was significantly high when compared with that of normal children. HFA, despite showing an elevation in the excretion of uric acid, was significantly lesser (P < 0.05) when compared with that of normal children. The level of creatinine excreted was found to be significantly lower (P < 0.001) in LFA, MFA, and HFA (P < 0.01) when compared with that of normal children. , The uric acid/creatinine (UA/Cr) ratio in all the three groups of autistic children was found to be significantly higher when compared with age- and sex-matched normal children. , representing the Spearman's rank correlation of OSI vs. CARS, showed interesting evidence that TBARS, LHP, PC, total peroxides, OSI, and UA/Cr had positive correlation with the degree of severity of autism. The total antioxidant capacity and sulfhydryl groups showed negative correlation.
Figure 1. Level of 4-HNE in the urine of autistic children compared with age and sex-matched normal children. Results were expressed as mean ± SD. *P < 0.001 (control vs. LFA, MFA, and HFA).
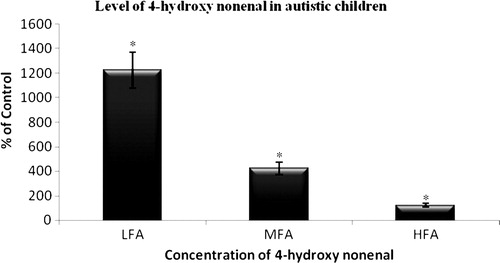
Table 2. Level of lipid peroxides, lipid hydroperoxides, and PC in the urine of autistic children compared with age- and sex-matched normal children
Table 3. Level of protein sulfhydryl, non-protein sulfhydryl, and total sulfhydryl groups in the urine of autistic children compared with age- and sex-matched normal children
Table 4. Level of TAC, total peroxides, and OSI in the urine of autistic children compared with age- and sex-matched normal children
Table 5. Level of uric acid, creatinine, and UA/Cr ratio in the urine of autistic children compared with age- and sex-matched normal children
Table 6. Spearman rank correlation between parameters vs. CARS
Discussion
The oxidative stress in autism may be caused by an imbalance between the generation of ROS by endogenous/exogenous pro-oxidants and the defense mechanism against ROS by antioxidants. The overall result of the present study revealed that there are significant abnormalities in the urinary levels of biomarkers of oxidative stress in autistic children when compared with normal children. Further, the results suggest that there is a significant positive correlation between urinary oxidative stress markers (TBARS, 4-HNE, lipid hydroperoxides, and protein carbonyls) and significant inverse correlation between antioxidant principles (TAC, sulfhydryl groups) excreted in urine and autism severity measured using CARS.
Elevated urinary lipid peroxidation products in autistic children showed in this study suggests the role of oxidative stress on the pathogenesis of autism. Investigators have previously reported that lipid peroxidation products are high in the plasma of children with autism. Lipids, which comprise biological membranes, are easily oxidized, particularly if highly unsaturated. This oxidation of membrane lipid leading to cell injury could be the result of many factors like environmental toxins, metal toxins, genetic factors, etc. Autistic children, the susceptible candidates to these factors, show an increased oxidative stress in vivo which is reflected by elevated urinary excretion of TBARS, 4-HNE, and LHP as presented in the study. Isoprostanes are produced from the free-radical oxidation of arachidonic acid through non-enzymatic oxidation of cell membrane lipids. Ming et al. (2005)Citation36 reported increased excretion of 8-isoprostanes F2alpha in the urine of children with autism, which again supports the results of the study.
In our previous study, we have reported that the autistic children are targets of metal (Cu, Hg, and Pb) toxicity.Citation37 There are reports describing that the metal-catalyzed oxidation of polyunsaturated fatty acids leads to the formation of several products that have been shown to form PC derivatives with proteins. These include (i) MDA, which reacts with lysine residues of proteins to form stable carbonyl derivatives;Citation38 (ii) alpha, beta-unsaturated aldehydes, such as 4-HNE, which can undergo Michael addition-type reactions with the epsilon-amino group of lysine residues, the sulfhydryl group of cysteine residues, and the imidazole group of histidine residues;Citation39 and (iii) lipid peroxides, which can undergo metal ion-catalyzed conversion to alkoxyl and peroxyl radicals that can react directly with side chains of some amino acids residues to form carbonyl derivatives by mechanisms analogous to those obtained with H2O2.Citation40 The present study indicates a similar condition of metal-catalyzed oxidation of polyunsaturated fatty acids in autistic children with the consequence of making them the victims of oxidative stress. We could also find a significant increase in the concentration of lipid hydroperoxides in the urine of autistic children where lipid hydroperoxides are also formed due to metal-catalyzed oxidation of polyunsaturated lipids as a consequence of copper toxicity which was observed in our previous study.Citation37 Protein carbonyls are the most widely used markers of oxidative modification of proteins which is found to be elevated in the urine of autistic children. Proteins constitute the major ‘working force’ for all forms of biological work. Their exact conformation and pattern of folding are tightly connected to their activity and function. Reactive oxygen and nitrogen species (ROS & RNS) are formed during normal metabolism and in higher fluxes under pathological conditions. They cause cellular damage, an important part of which is the oxidation of amino acid residues on proteins, forming protein carbonyls, found to be elevated in the urine of autistic children when compared with age- and sex-matched normal children.
The –SH (reduced thiol) groups that exist both intracellularly and extracellularly either in free form (reduced glutathione) or bound to proteins (protein bound thiols) play major role in maintaining the antioxidant status of the body.Citation41 The thiols are the major antioxidants in body fluids which are known to reduce highly reactive free radicals thus protecting the biomolecules. Such thiols have been studied and determined in different disease conditions and found to be lower when compared with healthy controls.Citation41,Citation42 The present study also reports decreased excretion of thiol groups in the urine of autistic children when compared with normal children suggesting the prevalence of reduced antioxidant defense in tissues and fluids in vivo. This might be due to either low level of formation or high-level consumption for defense action of antioxidants in autistic children, which could be accounted for oxidative stress.
Among different body fluids, urine is suitably used for the assessment of TAC.Citation43,Citation44 Moreover, the antioxidant levels in human urine are reported to be higher than in blood plasma.Citation43,Citation45,Citation46 This is due to the presence of greater quantity of uric acid in urine than in plasma. Uric acid is the major contributor of TAC in blood plasmaCitation46 and also dominates total antioxidants of urine as it is present in higher concentrations in urine than in plasma.Citation43,Citation45 The present study shows a significant decrease in the level of total antioxidants in the urine of the autistic children, whereas the level of total peroxides showed a significant increase which naturally indicates the increased OSI in autistic children thereby reflecting the role of oxidative stress in the pathogenesis of autism.
Uric acid has been considered as a marker of oxidative stress,Citation47 and may have a potential therapeutic role as an antioxidant.Citation48 On the other hand, like other strong reducing substances such as ascorbate, uric acid can also act as a pro-oxidant,Citation49 particularly at elevated levels. A significantly higher (P < 0.001) urinary UA/Cr ratio in autistic children is observed when compared with normal children. Uric acid is a product of purine catabolism including ATP which is found to be elevated in autistic children. This may be due to enhanced xanthine oxidase activity and might contribute to oxidative stress in autistic children, and might have resulted in deprivation of energy for neuromuscular function.
Hypoxia, or hypoxiation, is a pathological condition in which the body as a whole (generalized hypoxia) or a region of the body (tissue hypoxia) is deprived of adequate oxygen supply. A mismatch between oxygen supply and its demand at the cellular level may result in a hypoxic condition. It is known that abnormalities in brain development during pre- and peri-natal stages arise under the influence of different stress factors. The role of hypoxia as a stress factor in ischemia and asphyxia is well known. The role of hypoxia as a stress factor, however, is not well understood, though according to modern conceptions, it is included in the pathogenesis of almost any disorder. Several studies have described cerebral hypo perfusion in autismCitation50 and this decreased blood flow has been reported to correlate with core autistic symptoms leading to relative cerebral hypoxia. Hypoxia can impair mitochondrial function and diminish ATP production.Citation51 Brief hypoxia impairs cerebral oxidative metabolism leading to an anaerobic glycolysis to generate ATP. During anaerobic conditions 1 molecule of glucose yields only 2 molecules of ATP as opposed to producing 38 molecules of ATP during aerobic conditions. During prolonged hypoxia, cardiac output falls, cerebral blood flow is compromised and a combined hypoxic−ischemic insult produces further failure of oxidative phosphorylation and ATP production which is sufficient to cause cellular damage. Lack of ATP and increase excitotoxic cellular damage leads to an accumulation of adenosine diphosphate and adenosine monophosphate, which is then catabolized to adenosine, inosine, and hypoxanthine. If there is uninterrupted tissue hypoxia with reperfusion injury, hypoxanthine is oxidized to xanthine and uric acid in the presence of xanthine oxidase. This leads to increase in uric acid production, which comes out in blood from tissues and is excreted in urine.
The present investigation also emphasizes that the oxidative status measured by the urinary stress markers can be correlated with the severity of autism (LFA > MFA > HFA). There also exists a negative correlation between antioxidant status and degree of severity, i.e. lower the level of antioxidants the more severe is autism. These data support the use of oxidative stress markers and antioxidants as biomarkers of autism. The study also reveals that urine samples can be used as source for the analysis of oxidative stress markers and antioxidants, such as sulfhydryl groups, to monitor the level of oxidative stress in autistic children.
Acknowledgment
The author MD Lakshmi Priya thanks Indian Council of Medical Research, New Delhi for the financial assistance. The authors thank AIKYA (the school for special children), Mandaveli, Chennai, Tamil Nadu, India and the parents of autistic children for their cooperation.
References
- Lord C, Cook EH, Leventhal BL, Amaral DG. Autism spectrum disorders. Neuron 2000;28(2):355–63.
- Yeargin-Allsopp M, Rice C, Karapurkar T, Doernberg N, Boyle C, Murphy C. Prevalence of autism in a US metropolitan area. J Am Math Assoc 2003;289:49–55.
- Abha Chauhan, Ved Chauhan. Review: oxidative stress in autism. Pathophysiology 2006;13:171–81.
- Chauhan A, Chauhan V, Brown WT, Cohen IL. Oxidative stress in autism: increased lipid peroxidation and reduced serum levels of ceruloplasmin and transferrin-the antioxidant protein. Life Sci 2004;75:2539–49.
- McGinnis WR. Oxidative stress in autism. Altern Ther Health Med 2004;10:22–36.
- Prabakaran S, Swatton JE, Ryan MM, Huffaker SJ, Huang JT, Griffin JL, et al. Mitochondrial dysfunction in schizophrenia: evidence for compromised brain metabolism and oxidative stress. Mol Psychiatry 2004;9:684–97.
- Bilici M, Efe H, Koroglu MA, Uydu HA, Bekaroglu M, Deger O. Antioxidative enzyme activities and lipid peroxidation in major depression: alterations by antidepressant treatments. J Affect Disord 2001;64:43–51.
- Kuloglu M, Atmaca M, Tezcan E, Ustundag B, Bulut S. Antioxidant enzyme and malondialdehyde levels in patients with panic disorder. Neuropsychobiology 2002;46:186–9.
- Kuloglu M, Atmaca M, Tezcan E, Gecici O, Tunckol H, Ustundag B. Antioxidant enzyme activities and malondialdehyde levels in patients with obsessive-compulsive disorder. Neuropsychobiology 2002;46:27–32.
- Stohs SJ. The role of free radicals in toxicity and disease. J Basic Clin Physiol Pharmacol 1995;6:205–28.
- Fridovich I. Biological effects of the superoxide radical. Arch Biochem Biophys 1986;247:1–11.
- McCord JM, Day ED. Superoxide dependent production of hydroxyl radical catalyzed by iron-EDTA complex. FEBS Lett 1978;86:139–42.
- Hartley DP, Kolaja KL, Reichard J, Petersen DR. 4-Hydroxynonenal and malondialdehyde hepatic protein adducts in rats treated with carbon tetrachloride: immunochemical detection and lobular localization. Toxicol Appl Pharmacol 1999;161:23–33.
- Girotti AW. Lipid hydroperoxide generation, turnover, and effector action in biological systems. J Lipid Res 1998;39:1529–42.
- Fruebis J, Parthasarathy S, Steinberg D. Evidence for a concerted reaction between lipid hydroperoxides and polypeptides. Proc Natl Acad Sci. USA 1992;89:10588–92.
- Bielicki JK, Forte TM. Evidence that lipid hydroperoxides inhibit plasma lecithin:cholesterol acyltransferase activity. J Lipid Res 1999;40:948–54.
- Tien M, Berlett BS, Levine RL, Chock PB, Stadtman ER. Peroxynitrite-mediated modification of proteins at physiological carbon dioxide concentration: pH dependence of carbonyl formation, tyrosine nitration, and methionine oxidation. Proc Natl Acad Sci. USA 1999;96:7809–14.
- Garrison WM. Reaction mechanisms in the radiolysis of peptides, polypeptides, and proteins. Chem Rev 1987;87:381–98.
- Berlett BS, Stadtman ER. Protein oxidation in aging, disease, and oxidative stress. J Biol Chem 1997;272:20313–6.
- Armstrong DA, Buchanan JD. Reactions of O2., and H2O2 and other oxidants with sulfhydryl enzymes. Photochem Photobiol 1978;28:743–55.
- Cao G, Prior RL. Comparison of different analytical methods for assessing total antioxidant capacity of human serum. Clin Chem 1998;44:1309–15.
- Laura Vergani, Lanza Cristina, Rivaro Paola, Abelmoschi M Luisa, Genti Shyti, Veneselli Edvige, et al. Metals, metallothioneins and oxidative stress in blood of autistic children. Res Autism Spectr Disord 2011;5(1):286–93.
- Magyar CI, Pandolfi V. Factor structure evaluation of the childhood autism rating scale. J Autism Dev Disord 2007;37:1787–94.
- Schopler E, Reichler R, Renner BR. The childhood Autism Rating Scale (CARS). 10th ed. Los Angeles, CA: Western Psychological Services; 1988.
- Rellini E, Tortolani D, Trillo S, Carbone S, Montecchi F. Childhood Autism Rating Scale (CARS) and Autism Behavior Checklist (ABC) correspondence and conflicts with DSM-IV criteria in diagnosis of autism. J Autism Dev Disord 2004;34:703–8.
- Draper HH, Hadley M. Malondialdehyde determination as index of lipid peroxidation. Methods Enzymol 1990;46:421–31.
- Jiang ZY, Hunt JV, Wolff SP. Ferrous ion oxidation in the presence of xylenol orange for detection of lipid hydroperoxides in low density lipoprotein. Anal Biochem 1992;202:384–9.
- Kinter M. Free Radicals – A practical approach. In: , Punchard NA, KeUy GJ, (eds.) Oxford: Oxford University Press; 1996. p. 136.
- Levine RL, Garland D, Oliver CN, Amici A, Climent I, Lenz A, et al. Methods Enzymol 1990;186:464–85
- Jozef Sedlak, Raymond H Lindsay. Estimation of total, protein-bound and nonprotein sulfhydryl groups in tissue with Ellman's reagent. Anal Biochem 1968;25:192–205.
- Miller NJ, Rice , Evans CA, Davies MJ, Gopinathan V, Milner A. A novel method for measuring antioxidant capacity and its application to monitoring the antioxidant status in premature neonates. Clin Sci 1993;84:407–12.
- Miyazawa T. Determination of phospholipid hydroperoxides in human blood plasma by a chemiluminescence-HPLC assay. Free Radiac Biol Med 1989;7:209–17.
- Whelton A, Watson P, Rock RC. Nitrogen metabolites and renal function. In: , Burtis CA, Aswood ER, (eds.) Teitz text book of clinical chemistry. 2nd ed. Philadelphia, WB: Saunders; 1993. p. 1513–75.
- Butler AR. The Jaffe reaction-identification of the colored species. Clin Chim Acta 1975;10:227–32.
- Lowry OH, Rosebrough NJ, Farr AL, Randall RJ. Protein measurement with the folin phenol reagent. J Biol Chem 1981;193:265–75.
- Ming X, Stein TP, Brimacombe M, Johnson WG, Lambert GH, Wagner GC. Increased excretion of a lipid peroxidation biomarker in autism. Prostaglandins, Leukot Essent Fatty acids 2005;73:379–84.
- Lakshmi , Priya MD, Geetha A. Level of trace elements (copper, zinc, magnesium and selenium) and toxic elements (lead and mercury) in the hair and nail of children with autism. Biol Trace Elem Res 2011;142(2):148–58.
- Burcham PC, Kuhan YT. Introduction of carbonyl groups into proteins by the lipid peroxidation product, malondialdehyde. Biochem Biophys Res Commun 1996;220:996–1001.
- Bruenner BA, Jones AD, German JB. Direct characterization of protein adducts of the lipid peroxidation product 4-hydroxy-2-nonenal using electrospray mass spectrometry. Chem Res Toxicol 1995;8:552–9.
- Kato Y, Mori Y, Makino Y, Morimitsu Y, Hiroi S, Ishikawa T, et al. Formation of Nepsilon-(hexanonyl) lysine in protein exposed to lipid hydroperoxide. A plausible marker for lipid hydroperoxide-derived protein modification. J Biol Chem 1999;274:20406–14.
- Prakash M, Upadhya S, Prabhu R. Protein thiols oxidation and lipid peroxidation in patients with uremia. Scand J Clin Lab Invest 2004;64:599–604.
- Mallikarjunappa S, Prakash M. Urine protein thios in chronic renal failure patients. Indian J Nephrol 2007;17(1):7–9.
- Kirschbaum B. Total urine antioxidant capacity. Clinica Chimica Acta 2001;305:167–73.
- Koracevic D, Koracevic G, Diordjevic V, Andrejevic S, Cosic V. Method for the measurement of antioxidant activity in human fluids. J Clin path 2001;54:356–61.
- Lissi E, Salim Hanna M, Pascual C, Del Castillo D. Evaluation of total antioxidant potential (TRAP) and total antioxidant reactivity from luminol-enhanced chemiluminescence measurements. Free Radic Biol Med 1995;18:153–8.
- Ziobro A, Bartosz G. A comparison of the total antioxidant capacity of some human body fluids. Cell Mol Biol Lett 2003;8:415–9.
- Becker BF. Towards the physiological function of uric acid. Free Radic Biol Med 1993;14(6):615–31.
- Glantzounis GK, Tsimoyiannis EC, Kappas AM, Galaris DA. Uric acid and oxidative stress. Curr Pharm Des 2005;11(32):4142–51.
- Proctor P. Electron-transfer factors in psychosis and dyskinesia. Physiol Chem Phys 1972;4(4):349–60.
- Zilbovicius M, Boddaert N, Belin P, Poline JB, Remy P, Mangin JF, et al. Temporal lobe dysfunction in childhood autism: a PET study. Am J Psychiatry 2000;157:1988–93.
- Magalhães J, Ascensão A, Soares JM, Ferreira R, Neuparth MJ, Marques F, et al. Acute and severe hypobaric hypoxia increases oxidative stress and impairs mitochondrial function in mouse skeletal muscle. J Appl Physiol 2005;99:1247–53.