Abstract
Objective
The protection conferred by a series of synthetic organoselenium compounds against genotoxicity and oxidative stress induced by a reference mutagen cyclophosphamide (CP) was assessed.
Method
Genotoxicity was induced in mice by CP treatment (25 mg/kg b.w.) for 10 consecutive days. Organoselenium compounds (3 mg/kg b.w.) were administered orally in a concomitant and pretreatment schedule. DNA damage in peripheral blood lymphocytes and frequency of chromosomal aberration in the bone marrow cells were measured. Liver tissues were collected for analysis of the activity of antioxidant and detoxifying enzymes, lipid peroxidation (LPO) level, glutathione content, and histopathology.
Results
Exposure to CP not only led to a significant increase in the percent of chromosomal aberration and DNA damage, but also enhanced generation of hepatic reactive oxygen species (ROS) and LPO level. The organoselenium compounds demonstrated marked functional protection against CP-induced genotoxicity. DNA damage and chromosomal aberration along with ROS generation were attenuated in the organoselenium-treated mice compared with the CP-treated control mice. CP caused marked depression in the activities of the selenoenzymes (glutathione peroxidase (GPx) and thioredoxin reductase (TRxR)) and other detoxifying and antioxidant enzymes, while treatment with organoselenium compounds restored all these activities towards normal.
Discussion
The protective effect of these compounds may be primarily associated with the improvement of the activity of antioxidant and detoxifying enzymes (including the selenoenzymes, GPx, and TRxR) that are known to protect the DNA and other cellular components from oxidative damage.
Introduction
Mutation is certainly related to many other relevant degenerative chronic diseases which, in turn, are among the main causes of death in the human population.Citation1 Reactive oxygen species (ROS) like H2O2, O2−•, and •OH produce a series of DNA lesions viz. base damage, single or double strand break, as well as DNA–DNA or DNA–protein crosslinks, which are associated with the DNA mutations and subsequently leads to many human diseases, including cancer.Citation2,Citation3
Selenium, as an essential element, is required for the activity of selenoenzymes, which have important antioxidative properties that prevent oxidative damage to DNA and other biomolecules.Citation4 It forms the active center of selenoenzymes such as glutathione peroxidase (GPx) and thioredoxin reductase (TRxR) that catalyze essential redox reactions and perform the function of an antioxidant in a number of metabolic and immunologic processes.Citation5 It has also been revealed that organic selenium is more efficiently absorbed in the bodyCitation6 and is less toxic than inorganic selenium compounds.Citation7 Therefore, the organic form of selenium is more advantageous for an organism than the inorganic form. Synthetic organoselenium compound like 1,4-phenylenebis(methylene)selenocyanate (p-XSC) was also known to inhibit 7,12-dimethylbenz(a)anthracene-induced DNA adduct formation in mammary glands of female Sprague-Dawley rats.Citation8
Here, we have focused on the effects of organoselenium compounds on DNA damage and chromosomal aberrations, induced by an indirect-acting mutagen cyclophosphamide (CP). Generally, the clinical use of CP, a commonly used oxazaphosphorine alkylating agent, has been extended from neoplastic diseases to organ transplantation and diverse disorders and as an immunosuppressive agent.Citation9 Wide utilization of CP as a reference mutagen is well recognized due to its ability to induce genotoxicity in a metabolically active state.Citation10,Citation11 The International Agency for Research on Cancer (IARC) has provided several evidences to classify CP as carcinogen for human and animals.Citation12 We had earlier designed and synthesized a series of naphthalimide-based organoselenocyanates which were found to be substantially less toxic than dietary sodium selenite, when tested in normal Swiss albino mice.Citation13 The aim of this present study is to reveal the protective efficacy of those naphthalimide-based organoselenocyanates against the toxicity imparted by CP. For this purpose, comet assay and chromosomal aberrations were used as a parameter for evaluating the protective effects of these compounds against genotoxicity induced by CP. The study was also complemented with measurements of ROS in liver tissue to examine the modulatory effect of the synthesized compounds on oxidative stress. It was also ascertained whether any alterations occurred in the activity of the selenoenzymes, GPx and TRx, due to organoselenium treatment. Additionally, activities of other antioxidant and detoxifying enzymes, such as glutathione-S-transferase (GST), superoxide dismutase (SOD), catalase (CAT), and glutathione content were measured as antioxidant endpoints. To assess the extent of cellular oxidative damage, level of thiobarbituric acid reactive substances (TBARS) was measured in liver tissues. Finally, histopathological study was done, to confirm the protective efficacy of the compounds against CP-induced toxicity.
Materials and method
Animals
Adult (5–6 weeks) Swiss Albino female mice (23 ± 2 g), bred in the animal colony of Chittaranjan National Cancer Institute were used in this study. The animals were maintained at controlled temperature under an alternating light and dark condition. Standard food pellets (EPIC rat and mice pellet) from Kalyani Feed Milling Plant, Kalyani, West Bengal, India and drinking water was provided ad libitum. The standard food pellets contain wheat flour –22.5%, roasted bengal gram flour –60%, skim milk powder –5%, casein –4%, refined groundnut oil –4%, salt mixture with starch –4%, wheat flour –22.5%. There was no added selenium in the food as supplement. The experiments were carried out following the guidelines laid down by our institutional ethical Committee for Care and Use of Laboratory Animals (Reg. no. 175/99/CPCSEA).
Synthesis of the compounds
The compounds 4a-d were prepared as described previously.Citation13 Compound 4e was prepared from the intermediate 3a by refluxing it with potassium thiocyanate (KSCN) in ethanol for 5 hours and purified by column chromatography ().
Figure 1. Synthesis of the compounds. (A) Synthesis of compounds 4a-c and 4e. Reagents and conditions: (i) H2N(CH2)5OH, water/EtOH, reflux, (ii) PBr3, ethyl acetate,70°C, (iii) KSeCN, acetone, rt, (iv) KSCN, ethanol, reflux. (B) Synthesis of compound 4d. Reagents and conditions: (i) Br(CH2)5Br, NaOMe/MeOH, (ii) KSeCN, acetone, rt.
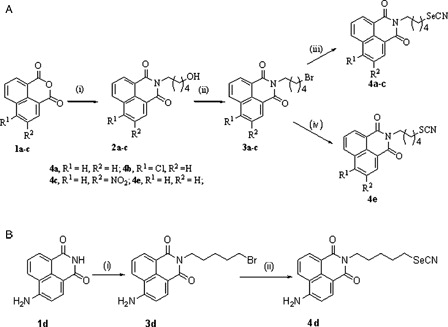
Experimental design
Animals were divided into four main groups. Groups III and IV were again subdivided into five different groups. Number of animals in each group was limited to six (n = 6) (). Animals of Gr. I received distilled water intraperitoneally (i.p.) and 5.5% propylene glycol in water orally for 10 days. Gr. II was administered CP i.p. at a dose of 25 mg/kg b.w. in water for 10 days. The five subdivided groups of Gr. III (concomitant treatment) were given five different test compounds (4a, 4b, 4c, 4d, and 4e) for 10 days. According to the selenium content, compound 4a was given at a dose of 3.0 mg/kg b.w., 4b at a dose of 3.2 mg/kg b.w., 4c at the dose of 3.3 mg/kg b.w., and 4d at a dose of 3.1 mg/kg b.w. Compound 4e, the sulfur analog of compound 4a, was given at a dose of 3.0 mg/kg b.w. The thiocyanate compound (4e) was tested for its biological activity in order to ensure the specific protective efficacy of the selenocyanate (–SeCN) moiety. CP was given as in Gr. II. Similarly, the five subdivided groups of Gr. IV (pretreatment) received the five different test compounds (4a, 4b, 4c, 4d, and 4e) at a dose of 3.0, 3.2, 3.3, 3.1, and 3.0 mg/kg b.w., respectively, for 15 days prior to CP treatment and then continued along with CP for 10 days.
The mice were sacrificed on Day 11, and the parameters described below were studied.
Experiments
Determination of hepatic ROS production
Measurement of hepatic ROS generation was done by employing the method of Driver et al.Citation14 with slight modifications. Liver tissues were homogenized in Locke's buffer (pH 7.4 containing 140 mM NaCl, 5 mM KCl, 10 mM HEPES, 1 mM CaCl2, 1 mM MgCl2, and 10 mM glucose) to yield a 10% w/v homogenate. A total of 250 µl of tissue homogenate was taken and loaded with 10 µM 2′,7′-dichlorodihydrofluorescein diacetate (DCFH-DA) to make a final volume of 3 ml and incubated for 45 minutes. ROS levels were measured using spectrofluorimeter (Varian Cary Eclipse, with an excitation set at 485 nm and emission at 530 nm) as a change in fluorescence intensity because of the conversion of non-fluorescent DCFH-DA to the highly fluorescent compound 2′,7′-dichlorofluorescein.
Measurement of extent of DNA damage by comet assay
DNA damage was measured by single cell gel electrophoresis or comet assay. Briefly, lymphocytes were separated from heparinized peripheral blood by Histopaque. The isolated lymphocytes were suspended in 0.5% (w/v) low-melting agarose. Subsequently, cells were layered over a frosted microscopic slide previously coated with a layer of 1.0% normal melting agarose. The slides were then immersed in a lysis buffer of pH 10 and left overnight. Slides were transferred into a horizontal electrophoresis chamber containing alkaline solution (300 mM NaOH, 1 mM Na2EDTA; pH 13.0). A presoaking of 20 minutes was done in order to unwind DNA. Electrophoresis was then carried out for 20 minutes (300 mA, 20 V). Slides were then washed thrice with neutralizing buffer (Tris buffer 0.4 M, pH 7.5) followed by staining with ethidium bromide (final concentration 20 µg/ml). Finally, slides were examined under a Leica fluorescence microscope and subjected to image analysis using comet assay software programme Komet 5.5. To avoid any selection bias, at least 100 cells from each sample were counted and the results were expressed as: (i) percentage of cells with tail (tailed cells) in each group and (ii) average tail length due to DNA migration in each group. DNA damage was quantified by tail length measurement.Citation15
Chromosomal aberration test
Mice were injected (i.p.) with 0.03% colchicine at 1 ml/100 g body weight 90 minutes before sacrifice. Marrow of the femur was flushed in 1% sodium citrate solution at 37°C and fixed in acetic acid/ethanol (1:3). Slides were prepared by the conventional flame drying techniqueCitation16 and followed by Giemsa staining for scoring of bone marrow chromosome aberrations. A total of 300 bone marrow cells were analyzed, 60 from each of 5 mice of a set.
Estimation of GPx activity
GPx activity in liver tissue sample was determined by NADPH oxidation using a coupled reaction system consisting of reduced glutathione, glutathione reductase, and hydrogen peroxide.Citation17 The enzyme activity was expressed as μmol NADPH utilized min−1 mg of protein−1 using extinction coefficient of NADPH at 340 nm as 6200 M−1 cm−1.
Estimation of TRxR activity
Hepatic TRxR activity in liver tissue was measured by Thioredoxin Reductase Assay Kit (Sigma Aldrich, Bangalore, India). A colorimetric method was used for the determination of the TRxR activity. In this process 3,5′-dithiobis (2-nitrobenzoic) acid (DTNB) was reduced with NADPH to 5-thio-2-nitrobenzoic acid (TBN). This TBN produced a strong yellow color which was measured at 412 nm.
Quantitative estimation of lipid peroxidation
Lipid peroxidation (LPO) was estimated in liver microsomal fraction. The level of lipid peroxides formed was measured using thiobarbituric acid and expressed as nmol of TBARS formed per mg of protein using an extinction coefficient of 1.56 × 105 M−1 cm.Citation18
Estimation of reduced glutathione level
Reduced glutathione (GSH) level was estimated in liver cytosol spectrophotometrically by the determination of dithiobis (2-nitro)-benzoic acid (DTNB) reduced by –SH groups by measuring the absorbance at 412 nm. The level of GSH was expressed as nmol/mg of protein.Citation19
Estimation of GST activity
The GST activity in liver cytosol was determined from the increase in absorbance at 340 nm with 1-chloro-2-4-dinitrobenzene (CDNB) as the substrate and specific activity of the enzyme expressed as formation of 1-chloro-2-4-dinitrobenzene (CDNB)-GSH conjugate min−1 mg of protein−1.Citation20
Estimation of CAT activity
The activity of CAT in the liver tissue sample was determined spectrophotometrically at 240 nm and expressed as unit/mg of protein where the unit is the amount of enzyme that liberates half the peroxide oxygen from H2O2 per second at 25°C.Citation21
Estimation of SOD activity
The SOD activity was determined by quantification of Pyrogallol auto oxidation inhibition and expressed as unit/mg of protein. Auto oxidation of Pyrogallol in Tris-HCl buffer (50 mM, pH 7.5) is measured by increase in absorbance at 420 nm.Citation22,Citation23 One unit of enzyme activity is defined as the amount of enzyme necessary for inhibiting the reaction by 50%.
Histopathological studies
Liver tissues were fixed overnight in 10% buffered neutral formalin, processed to paraffin wax, sectioned at 5 µm, and stained with hematoxylin–eosin for examination by light microscopy.
Estimation of protein
Protein was estimated spectrophotometrically by the Lowry method.Citation24
Statistical analysis
The data are presented as mean ± SD. Comparison between groups was done using one-way analysis of variance (ANOVA). When a P value obtained from ANOVA was significant, Tukey's test was applied to test for differences among groups. Differences were considered significant if P < 0.05.
Results
Organoseleniums inhibited the ROS level
CP treatment markedly increased the ROS level almost three-fold (P < 0.05) compared to Gr. I. Significant (P < 0.05) preservation of ROS level was observed by the treatment with 4a-d. Highest reduction in ROS level was detected with compound 4d and in case of pretreatment the reduction was three-fold (P < 0.05) compared to Gr. II. No significant (P > 0.05) changes were observed with 4e treatment ().
Figure 3. Effect of organoselenium compounds on ROS induced by CP in hepatic tissue. Results are expressed as means ± SD (n = 6). Comparisons are made between – (i) Gr. I vs. Gr. II: P < 0.05; (ii) Gr. I vs. Gr. III: P < 0.05 for 4a, 4b, 4c, 4d, 4e; (iii) Gr. I vs. Gr. IV: P < 0.05 for 4a, 4b, 4c, 4e and P > 0.05 for 4d; (iv) Gr. II vs. Gr. III: P < 0.05 for 4a, 4b, 4c, 4d and P > 0.05 for 4e; (v) Gr. II vs. Gr. IV: P < 0.05 for 4a, 4b, 4c, 4d and P > 0.05 for 4e; (vi) Gr. III vs. Gr. IV: P < 0.05 for 4a, 4b, 4c, 4d and P > 0.05 for 4e (One-way ANOVA followed by Tukey's test).
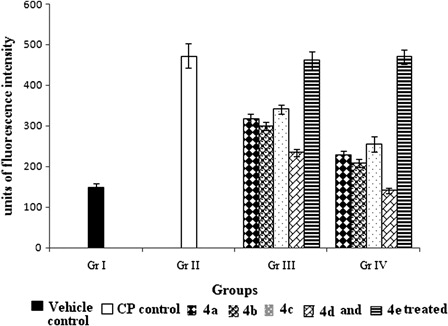
Protection against CP-induced DNA damage
CP treatment resulted in a significant DNA damage. Prominent induction of comet tail length was noted with respect to the vehicle control group. After CP treatment (Gr. II) incidence of the damaged cells as well as tail length of the comet was increased by six-fold and seven-fold (P < 0.05), respectively. Concomitant and pretreatment with compound 4a-d lowered the percentage of damaged cells as well as the tail length of the damaged cells ().
Table 1. Effect of compounds (4a-e) on DNA damage in lymphocytes of CP-treated mice
Protection from chromosomal damage by organoselenium treatment
CP treatment led to significant increase in percentage of aberrant cells (54.73 ± 1.02) compared to vehicle control (8.26 ± 0.70). Concomitant and pretreatment with organoselenium compounds significantly attenuated the CP-induced incidence of aberrant cells in a compound specific manner (). It should also be noted that, pretreatment with the compounds (4a-d) always proved to be advantageous over concomitant treatment in minimizing chromosomal damage.
Figure 4. Effect of organoselenium compounds on chromosomal aberration induced by CP in bone marrow cells. Results are expressed as means ± SD (n = 6). Comparisons are made between – (i) Gr. I vs. Gr. II: P < 0.05; (ii) Gr. I vs. Gr. III: P < 0.05 for 4a, 4b, 4c, 4d, and 4e; (iii) Gr. I vs. Gr. IV: P < 0.05 for 4a, 4b, 4c, 4d, and 4e; (iv) Gr. II vs. Gr. III: P < 0.05 for 4a, 4b, 4c, and 4d and P > 0.05 for 4e; (v) Gr. II vs. Gr. IV: P < 0.05 for 4a, 4b, 4c, and 4d and P > 0.05 for 4e; (vi) Gr. III vs. Gr. IV: P < 0.05 for 4a, 4b, 4c, and 4d and P > 0.05 for 4e (one-way ANOVA followed by Tukey's test).
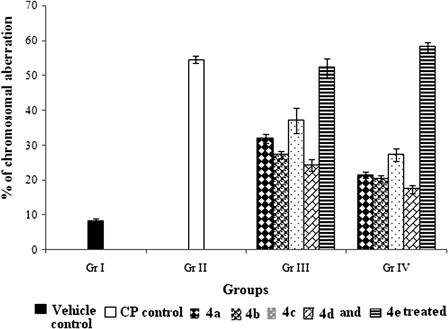
Increase in activity of selenoenzymes (GPx and TRxR) by organoseleniums
GPx activity decreased by 40% in CP-treated group (Gr. II) compared to Gr. I (A). TRxR, which represents a promising target for CP treatment, showed a dramatic inhibition of 70% (P < 0.05) in the CP-treated group in comparison to the vehicle control group (Gr. I; B). Treatment with organoselenium compounds enhanced the activities of both the enzymes. Pretreatment with 4d showed 71.7 and 159% increase (P < 0.05) in GPx and TRxR activity, respectively, relative to Gr. II. No evidence of compound 4e having any effect (P > 0.05) on the activities of these two enzymes was found.
Figure 5. Effect of organoselenium compounds on (A) GPx and (B) TRxR, in hepatic tissue. Results are expressed as means ± SD (n = 6). For both GPx and TRx comparisons are made between – (i) Gr. I vs. Gr. II: P < 0.05; (ii) Gr. I vs. Gr. III: P < 0.05 for 4a, 4b, 4c, 4d, and 4e; (iii) Gr. I vs. Gr. IV: P < 0.05 for 4a, 4b, 4c, and 4e and P > 0.05 for 4d; (iv) Gr. II vs. Gr. III: P < 0.05 for 4a, 4b, 4c, and 4d and P > 0.05 for 4e; (v) Gr. II vs. Gr. IV: P < 0.05 for 4a; 4b, 4c, 4d and P > 0.05 for 4e; (vi) Gr. III vs. Gr. IV: P < 0.05 for 4a, 4b, 4c, 4d and P > 0.05 for 4e (one-way ANOVA followed by Tukey's test).
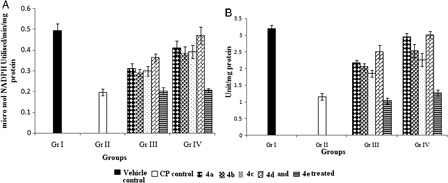
Estimation of LPO level
LPO, as a mark of cellular injury, was assessed across groups. There was a significant (P < 0.05) increase of 64.3% in hepatic microsomal LPO level in CP-treated animals in Gr. II compared with Gr. I. Treatment with organoselenium compounds could efficiently protect liver from LPO (A).
Figure 6. Effect of organoselenium compounds on hepatic (A) TBARS level and (B) GSH level in CP-treated mice. Results are expressed as means ± SD (n = 6). For TBARS level, comparisons are made between – (i) Gr. I vs. Gr. II: P < 0.05; (ii) Gr. I vs. Gr. III: P < 0.05 for 4b, 4c, 4e and P > 0.05 for 4a, 4d; (iii) Gr. I vs. Gr. IV: P < 0.05 for 4e and P > 0.05 for 4a, 4b, 4c, 4d; (iv) Gr. II vs. Gr. III: P < 0.05 for 4a, 4b, 4c, 4d and P > 0.05 for 4e; (v) Gr. II vs. Gr. IV: P < 0.05 for 4a, 4b, 4c, 4d and P > 0.05 for 4e; (vi) Gr. III vs. Gr. IV: P > 0.05 for all the compounds. For GSH level, comparisons are made between (i) Gr. I vs. Gr. II: P < 0.05; (ii) Gr. I vs. Gr. III: P < 0.05 for 4a, 4b, 4c, 4d, and 4e; (iii) Gr. I vs. Gr IV: P < 0.05 for 4b, 4c, 4d, 4e and P > 0.05 for 4a; (iv) Gr. II vs. Gr. III: P < 0.05 for 4a, 4b, 4c, 4d and P > 0.05 for 4e; (v) Gr. II vs. Gr. IV: P < 0.05 for 4a, 4b, 4c, and 4d and P > 0.05 for 4e; (vi) Gr. III vs. Gr. IV: P < 0.05 for 4c and 4d, P > 0.05 for 4a, 4b, and 4e (one-way ANOVA followed by Tukey's test).
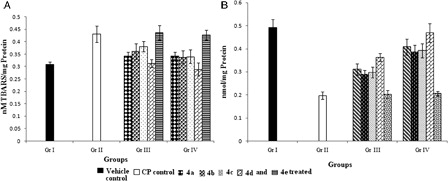
Glutathione and associated antioxidant enzyme system
In comparison with vehicle control group, significant (P < 0.05) inhibition (52.3%) of GSH level was observed in the CP-treated control group (B). The GSH-requiring detoxification enzyme GST, exhibited a significant (P < 0.05) decline in activity in Gr. II after CP treatment by 52.8% relative to Gr. I (A). A significant depletion was also observed in other antioxidant enzymes, CAT and SOD (by 59.8 and 31.4% (P < 0.05), respectively; B and C]. Treatment with organoseleniums (4a-d) restored all the levels towards normal. Pretreatment with the compounds (4a-d) showed better efficacy than concomitant treatment in elevating the activities of antioxidant enzymes. In this respect pretreatment with compound 4d was found to be most effective.
Figure 7. Effect of organoselenium compounds on hepatic (A) GST (B) CAT, and (C) SOD activity. Results are expressed as means ± SD (n = 6). For GST, comparisons are made between – (i) Gr. I vs. Gr. II: P < 0.05; (ii) Gr. I vs. Gr. III: P < 0.05 for 4a, 4b, 4c, 4d, and 4e; (iii) Gr. I vs. Gr. IV: P < 0.05 for 4a, 4b, 4c, and 4e and P > 0.05 for 4d; (iv) Gr. II vs. Gr. III: P < 0.05 for 4a, 4b, and 4d and P > 0.05 for 4c and 4e; (v) Gr. II vs. Gr. IV: P < 0.05 for 4a, 4b, 4c, and 4d and P > 0.05 for 4e; (vi) Gr. III vs. Gr. IV: P < 0.05 for 4a, 4b, 4c, and 4d and P > 0.05 for 4e. For CAT, comparisons are made between – (i) Gr. I vs. Gr. II: P < 0.05; (ii) Gr. I vs. Gr. III: P < 0.05 for 4a, 4b, 4c, 4d, and 4e; (iii) Gr. I vs. Gr. IV: P < 0.05 for 4a, 4b, 4c, and 4e and P > 0.05 for 4d; (iv) Gr. II vs. Gr. III: P < 0.05 for 4a, 4b, 4c, and 4d and P > 0.05 for 4e; (v) Gr. II vs. Gr. IV: P < 0.05 for 4a, 4b, 4c, and 4d and P > 0.05 for 4e; (vi) Gr. III vs. Gr. IV: P < 0.05 for 4a, 4b, and 4d and P > 0.05 for 4c and 4e. For SOD, comparisons are made between – (i) Gr. I vs. Gr. II: P < 0.05; (ii) Gr. I vs. Gr. III: P < 0.05 for 4a, 4b, 4c, 4d, and 4e; (iii) Gr. I vs. Gr. IV: P < 0.05 for 4a, 4b, 4c, 4d, and 4e; (iv) Gr. II vs. Gr. III: P < 0.05 for 4a, 4b, 4c, and 4d and P > 0.05 for 4e; (v) Gr. II vs. Gr. IV: P < 0.05 for 4a, 4b, 4c, 4d and P > 0.05 for 4e; (vi) Gr. III vs. Gr. IV: P < 0.05 for 4a, 4b, 4c, 4d and P > 0.05 for 4e (one-way ANOVA followed by Tukey's test).
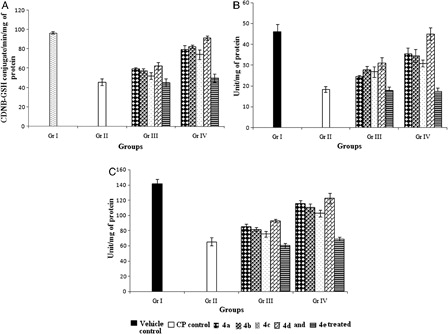
Histopathological study
While the vehicle control group revealed normal architecture of the hepatocytes, CP administration resulted in substantial diffuse swelling of the hepatocytes and narrowing of sinusoidal spaces. Normal appearance of these cells was restored in the organoselenium-treated mice (only the photomicrographs of compound 4d-treated mice have been shown). Concomitant treatment with organoselenium showed mild hepatic cell swelling around central vein, but in pretreatment group, liver appeared almost normal ().
Figure 8. Histopathology of liver. (A) Vehicle control group showing normal arrangement of hepatocytes with clear nuclei (H&E; ×400). (B) Treatment with CP showing diffuse marked swelling of hepatocytes and narrowing of sinusoidal spaces of hepatocytes indicated by arrow (H&E; ×400). (C) Concomitant treatment with compound 4d showing mild swelling of hepatocytes indicated by arrow (H&E; ×400). (D) Pretreatment with 4d showing almost normal hepatocytes and normal architecture (H&E; ×400).
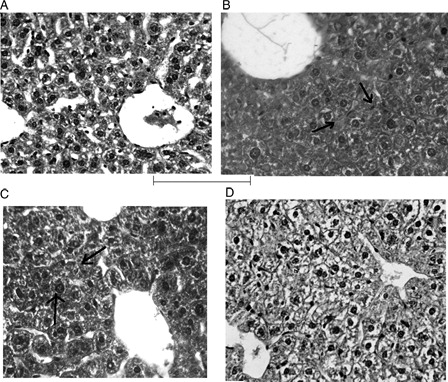
Discussion
The present study elicited that CP administration generates greater amount of ROS in liver homogenate of Swiss albino mice. This generation of ROS is possibly associated with the intracellular phosphoramide mustard and acrolein, the principal alkylating metabolites, formed by the hepatic activation of CP.Citation25 We have found that ROS generation was lowered by the treatment with organoselenocyanates. This may have resulted, in part, due to the direct scavenging of ROS by the organoselenium compounds.
Excessive production of ROS might be the prime cause of severe DNA damage as observed from the comet assay of lymphocytes. CP, an indirect-acting mutagen, damages chromosomes through generation of free radicals and alkylating DNA thereby producing mutation.Citation26 The mutagenic potency of CP was confirmed in the study by the enhanced percentage of chromosomal aberration noted in the bone marrow cells after CP administration for 10 days. Restoration of these levels toward normal after organoselenium treatment in Gr. III and IV mice suggests a protective activity and antigenotoxic efficacy of synthesized organoselenium compounds. This might be in part due to the direct scavenging activity of the compounds against the ROS, which is one of the major causes of DNA damage and chromosomal aberration.
A number of synthetic organoselenium compounds were found effective in maintaining the homeostasis of the host against the stress induced by carcinogen like 7,12-Dimethylbenz(a)anthracene (DMBA).Citation27,Citation28 The protective effect of organoselenium is believed to be primarily associated with the presence of selenium in GPx and TRxR, selenoenzymes that are known to protect DNA and other cellular components from oxidative damage.Citation29,Citation30 The present study shows that treatment with organoselenium compounds significantly up-regulates the activity of selenoenzymes (GPx and TRxR) to efficiently normalize the damaged conditions. Generally, selenium compounds (except selenomethionine) metabolized to a common intermediate selenide. This common intermediate is then used for the synthesis of selenoproteins (selenoenzymes) or excreated after stepwise methylation. On the other hand, selenomethionine first converted to selenocysteine via trans-selenation pathway and then metabolized to selenide by beta-lyase or directly by gamma-lyase. Beside this, through the unregulated pathway, selenomethionine can also be utilized for the synthesis of proteins without the body distinguishing between selenomethionine and methionine.Citation31 Thus, the enhancement of activities of the selenoenzymes after treatment with organoselenium compounds may have arisen due to the increase in selenoprotein level. This increased level of the selenoproteins, may in turn, up-regulate the total level of selenoenzymes in the cells.Citation32 Such altered levels of selenoenzymes may also influence other antioxidant and detoxifying enzyme systems.
GSH and its associated enzyme (GST) protect cellular macromolecules and organelles from peroxides. GSH is a versatile molecule and can directly scavenge electrophiles such as acroleinCitation33 or act as a co-substrate for GPX. Cytosolic GSTs are a superfamily of multifunctional enzymes involved in the cellular detoxification of many endobiotic and xenobiotic compounds. A prominent catalytic activity performed by eukaryotic GSTs is the conjugation of glutathione (GSH) to a number of toxic electrophilic compounds, thus promoting their excretion from the cell.Citation34 On the other hand, SOD catalyzes the dismutation of superoxide anion to H2O2, which is in turn, the substrate of CAT. In this investigation treatment with CP led to oxidative stress as evident from significant increase in the LPO level and depletion in the GSH level as well as in GST, SOD, and CAT activity. However, treatment with organoselenium significantly (P < 0.05) preserved the GSH level and GST, SOD, and CAT activities, as well as decreased the formation of LPO by-product, TBARS. This up-regulation of phase II detoxifying and antioxidant enzyme system is in line with the work done by El-Bayoumy et al.Citation35 where they showed that organoselenium compounds like p-XSC and its glutathione conjugate could modulate the genes of phase I as well as phase II detoxifying enzymes in the process of detoxification from carcinogen. In addition to the inhibition of oxidative stress, prevention of mutagen-induced cellular toxicities by up-regulating the detoxification enzymes, may be considered to be a result of the antigenotoxic property of the compounds. Increased activity of the selenoenzymes after selenium treatment provides additional protection to the host from oxidative damage by free radicals. This might spontaneously increase the activity of other antioxidant and detoxifying enzyme systems. Moreover increased GSH level could inactivate CP by directly binding to it via the sulfhydryl residuals.
Finally, the histopathological studies affirm that CP can damage liver tissues. This was manifested by the marked diffuse swelling of hepatocytes and narrowing of the sinusoidal spaces and may have been caused due to membrane damaging potential of the CP metabolites. These pathological changes correlated well with the altered enzyme activities. During treatment with the organoselenium compounds, these abnormal pathological conditions of tissue injury were normalized by protection of the tissues from oxidative damage.
In evaluating the protective effect of the synthesized organoselenium compounds against CP-induced cellular toxicity, it was observed that the pretreatment with the compounds gave better results than the concomitant treatment schedule. This reflects the preventive activity of the compounds against mutagen-induced toxicity. It was also observed that the compound 4d (having –NH2 group in the naphthalimide ring) was most effective in protecting the host. It is likely that the release of the cyanide from RSeCN in the presence of glutathione or protein thiol groups is the initial fate of organoselenocyanates.Citation36 At this point the chemical structure of the R group may influence the activity or subsequent release of the selenium moiety through the scission of R–Se bond, thereby altering biological availability.Citation37 One of the most noticeable features of this study is the difference between the biological activities of the organoselenocyanates with the organothiocyanate (4e). Compound 4e carried no promising protective efficacy against CP induce toxicity. These results validate the specificity of selenium in providing the protective efficacy of the synthesized organoselenium compounds. Furthermore, selenocyanates (–SeCN) were used as the carrier of selenium because they are known to be efficiently metabolized to selenols (–SeH) and therefore represent a convenient precursor compound. Generally, organoselenium compounds are known to convert to the similar metabolite selenol or to seleno alpha-keto acids.Citation38 Selenium as selenol (R–SeH; pKa 5.2) is more acidic than thiol (R-SH; pKa 8.5) and readily dissociated at physiological pH, which may contribute to its biological reactivity.Citation39
This study provides evidences for the protective efficacy of the organoseleniums against CP-induced ROS mediated genotoxicity. It is reasonable to assume that organoselenium compounds are able to protect cells from CP-induced toxicity through different possible pathways – (i) direct scavenging of the ROS, (ii) modulation of the antioxidant selenoenzymes GPx and TRxR; (iii) induction of the antioxidant enzymes like GST, SOD, CAT which provide further protection against the oxidative stress, and (iv) direct inactivation of CP through increased GSH level. However, additional investigations are required in this context to explore the other possible mechanisms involved with respect to the protective action of these synthetic organoseleniums.
Acknowledgements
This work was supported by Grant from CSIR (01(2160)/07/EMR II). Somnath Singha Roy gratefully acknowledges CSIR for Junior Research Fellowship. The authors also thank Dr Syamsundar Mandal, Department of Epidemiology and Biostatistics, CNCI, Kolkata for his valuable suggestion regarding statistical analysis, Dr Ila Das, Department of Cancer Chemoprevention, CNCI, Kolkata, and Santu Sarkar, IISER, Kolkata for their support in doing this work.
Conflict of interest
The authors alone are responsible for the content and writing of the paper.
References
- De Flora S, Izzotti A, Randerath K, Bartsch H, Nair J, Balansky R, et al. DNA adducts and chronic degenerative diseases. Pathogenetic relevance and implications in preventive medicine. Mutat Res 1996;366:197–238.
- Loft S, Poulsen HE. Cancer risk and oxidative DNA damage in man. J Mol Med 1996;74(6):297–312.
- Klaunig JE, Xu Y, Isenberg JS, Bachowski S, Kolaja KL, Jiang J, et al. The role of oxidative stress in chemical carcinogenesis. Environ Health Perspect 1998;106(1):289–95.
- El-Bayoumy K, Sinha R. Molecular chemoprevention by selenium: a genomic approach. Mutat Res 2005;591:224–6.
- Flohe L. The glutathione peroxidase reaction: molecular basis of the antioxidant functions of selenium in mammals. Curr Top Cell Regul 1985;27:437–78.
- Garcia MS. Organoselenium compounds as potential therapeutic and chemopreventive agents: a review. Curr Med Chem 2004;11:1657–69.
- Music I, Koziol-Montewka M, Tos-Luty S, Pasternak K, Tokaraska M, Kieczykowska M. Immunomodulatory effect of selenosemicarbazides and selenium inorganic compounds, distribution in organs after selenium supplementation. Bio Metals 1999;12:369–74.
- El-Bayoumy K, Chae YH, Upadhyaya P, Meschter C, Cohen LA, Reddy BS. Inhibition of 7,12-dimethylbenz(a)anthracene-induced tumors and DNA adduct formation in the mammary glands of female Sprague-Dawley rats by the synthetic organoselenium compound, 1,4-phenylenebis(methylene)selenocyanate. Cancer Res 1992;52:2402–7.
- Fraiser LH, Kanekal S, Kehrer JP. Cyclophosphamide toxicity. Characterising and avoiding the problem. Drugs 1991;42:781–95.
- Selvakumar E, Prahalathan C, Sudharsan PT, Varalakshmi P. Protective effect of lipoic acid on micronuclei induction by cyclophosphamide. Arch Toxicol 2006;80:115–9.
- Tripathi DN, Jena GB. Ebselen attenuates cyclophosphamide-induced oxidative stress and DNA damage in mice. Free Radic Res 2008;42(11):966–77.
- Sorsa M, Pyy L, Salomaa S, Nylund L, Yager JW. Biological and environmental monitoring of occupational exposure to cyclophosphamide in industry and hospitals. Mutat Res 1998;204:465–79.
- Singha Roy S, Ghosh P, Hossain SU, Chakraborty P, Biswas J, Mandal S, et al. Naphthalimide based novel organoselenocyanates: finding less toxic forms of selenium that would retain protective efficacy. Bioorg Med Chem Lett 2010;20:6951–55.
- Driver AS, Kodavanti PR, Mundy WR. Age related changes in reactive oxygen species production in rat brain homogenate. Neurotoxicol Teratol 2000;22(2):175–81.
- Singh NP, McCoy MT, Tice RR, Schneider EL. A simple technique for quantitation of low levels of DNA damage in individual cells. Exp Cell Res 1988;75:184–91.
- Preston RJ, Dean BJ, Galloway S, Holden H, McFee AF, Shelby M. Mammalian in vivo cytogenetic assays: analysis of chromosome aberrations in bone marrow cells. Mutat Res 1987;189(2):157–65.
- Paglia DE, Valentine WN. Studies on the quantitative and qualitative characterization of erythrocyte glutathione peroxidase. J Lab Clin Med 1967;70:158–69.
- Okhawa H, Ohishi N, Yagi K. Assay for lipid peroxides in animal tissue by thiobarbituric acid reaction. Annal Biochem 1979;95:351–8.
- Sedlack J, Lindsay RN. Estimation of total protein bound and non-protein sulphydryl groups in tissue with ellman reagent. Anal Biochem 1968;25:192–205.
- Habig WH, Pabst MJ, Jacoby WB. Glutathione-S-transferase, the first enzymatic step in marcapturic acid formation. J Biol Chem 1974;249:7130–39.
- Luck H. A spectrophotometric method for estimation of catalase. In: , Bergmeyer HV (ed.) Methods of enzymatic analysis. New York: Acad Press; 1963. p. 886–8.
- Marklund S, Marklund G. Involvement of the superoxide anion radical in autooxidation of pyrogallol and a convenient assay for superoxide dismutase. Eur J Biochem 1974;47:469–74.
- McCord JM, Fridovich I. Superoxide dismutase: an enzymatic function for erythrocuprein (hemoprotein). J Biol Chem 1969;244:6049–55.
- Lowry OH, Rosenbrough NJ, Farr AL, Randall RJ. Protein measurement with the folinphenol reagent. J Biol Chem 1951;193:265–76.
- Lee LK, Harman GS, Hohl RJ, Gingrieh RD. Fatal cyclophosphamide cardiomyopathy: its clinical course and treatment. Bone Marrow Transplant 1996;18:573–7.
- Povirk LF, Shuker DE. DNA damage and mutagenesis induced by nitrogen mustards. Mutat Res 1994;318:205–26.
- Talas ZS, Ozdemir I, Yilmaz I, Gok Y, Orun I. The investigation of the antioxidative properties of the novel synthetic organoselenium compounds in some rat tissues. Exp Biol Med. 2008;233:575–9.
- Talas ZS, Ozdemir I, Ates B, Gok Y, Yilmaz I. Modulating effects of selenium in adrenal medulla of rats exposed to 7,12-dimethylbenz[a]anthracene. Toxicol Ind Health. 2012 January 27. [Epub ahead of print].
- Trueba GP, Sanchez GM, Giuliani A. Oxygen free radical and antioxidant defense mechanism in cancer. Front Biosci 2004;9:2029–44.
- Schrauzer GN. Anticarcinogenic effects of selenium. Cell Mol Life Sci 2000;57:1864–73.
- Rubartelli A, Bajetto A, Allavena G, Wollman E, Sitia R. Secretion of thioredoxin by normal and neoplastic cells through a leaderless secretory pathway. J Biol Chem 1992;267:24161–4.
- Schrauzer GN. Selenomethionine: a review of its nutritional significance, metabolism and toxicity. J Nutr 2000;130:1653–6.
- Rotruck JT, Pope AL, Gasther HE, Hafeman DG, Hoekstra WG. Selenium biochemical role as a component of glutathione peroxidase. Science 1973;179:588–90.
- Armstrong RN. Structure, catalytic mechanism, and evolution of the glutathione transferases. Chem Res Toxicol 1997;10(1):2–18.
- El-Bayoumy K, Narayanan BA, Desai DH, Narayanan NK, Pittman B, Amin SG, et al. Elucidation of molecular targets of mammary cancer chemoprevention in the rat by organoselenium compounds using cDNA microarray. Carcinogenesis 2003;24(9):1505–14.
- Vadhanavikit S, Ganther HE. Metabolism of methylselenocyanate in the rat. FASEB J 1988;2:A1440.
- Clement Ip, El-Bayoumy K, Upadhyaya P, Ganther H, Vadhanavikit S, Thompson H. Comparative effect of inorganic and organic selenocyanate derivatives in mammary cancer chemoprevention. Carcinogenesis 1994;15(2):187–92.
- Facompre ND, El-Bayoumy K, Sun Y, Pinto JT, Sinha R. 1,4 phenylenebis(methylene)selenocyanate, but not selenomethionine, inhibits androgen receptor and Akt signaling in human prostate cancer cells. Cancer Prev Res 2010;3:975.
- Tinggi U. Selenium: its role as antioxidant in human health. Environ Health Prev Med 2008;13:102–8.