Abstract
Objectives
The aim of the study was to investigate the renoprotective effect of hesperidin (HDN), a citrus flavanoid with anti-oxidant properties against gentamicin (GEN)-induced nephrotoxicity in male Wistar albino rats.
Methods
Urea, uric acid, creatinine, thiobarbituric acid reactive substances (TBARS), nitric oxide (NO), total cholesterol, free fatty acids, and triglyceride levels were measured in the serum. Further, glutathione (GSH), superoxide dismutase (SOD), and glutathione peroxidase (GPx) activities were determined in the erythrocytes. Morphological changes in renal tissues were examined using light microscopy.
Results
Acute renal injury was evidenced by: (1) increased serum urea, uric acid, creatinine, TBARS, and NO, (2) decreased SOD, GPx, and GSH levels, and (3) necrosis in proximal tubules and glomerular atrophy. HDN supplementation to GEN-induced rats significantly decreased serum urea, uric acid, creatinine, TBARS, NO generation, but SOD, GPx activities, and GSH content increased when compared with GEN alone. Moreover, HDN supplementation resulted in complete reversal of GEN-induced tubular necrosis, and an increase in total cholesterol, free fatty acids, and triglycerides to normal levels.
Discussion
Our results suggested that HDN acts as a potent scavenger of free radicals in the kidney to prevent the toxic effects of GEN both at the biochemical and histopathological levels.
Introduction
Among the classes of aminoglycoside antibiotics, gentamicin (GEN) is widely used in clinical practice for the treatment of life-threatening Gram-negative bacterial infections.Citation1 The drug remains familiar due to its low cost, low level of resistance among enterobacteria, and its effectiveness against resistant β-lactam microorganisms.Citation2 However, a major complication in the use of aminoglycoside antibiotics is their potential ototoxicity and nephrotoxicity.Citation3 It was reported that GEN is one of the most common causes of acute renal failure, which occurs among 30% of the patients receiving the drug for more than 7 days.Citation4 A pharmacological study also revealed that the once-daily dosing regimen of gentamicin in patients was noted to be as effective and was less nephrotoxic than more frequent/multiple dosing per day.Citation5
GEN generates destructive reactive oxygen species (ROS) like superoxide anions, hydroxyl radicals, and hydrogen peroxides, as well as reactive nitrogen species (RNS) in the renal cortical mitochondria of rats which are considered to be the important mediators in GEN-induced nephrotoxicity.Citation6 Experiments with humans and animals have shown that ROS and RNS may perform a vital role in cellular damage and necrosis via several complex mechanisms including peroxidation of membrane lipids, protein denaturation, and DNA damage.Citation1 Nephrotoxicity induced by GEN is a complex phenomenon characterized by an increase in serum creatinine and blood urea nitrogen, a decrease in glomerular filtration rate (GFR), severe proximal renal tubular necrosis, glomerular hypertrophy, glomerular congestion, and inflammation followed by renal deterioration and renal failure.Citation7,Citation8
The administration of several compounds with antioxidant properties, including melatonin,Citation9 diallyl sulfide,Citation10 thymoquinone,Citation11 quercetin,Citation8 curcumin,Citation12 caffeic acid phenethyl ester,Citation13 and resveratrolCitation14 have all been successfully used to prevent or ameliorate GEN-induced nephrotoxicity. Flavonoids comprise a large group of secondary metabolites that occur widely throughout fruits, vegetables, nuts, seeds, and beverages such as tea and wine. Hesperidin (HDN, 5,7,3′-trihydroxy-4′-methoxyflavanone-7-rhamnoglucoside) belongs to the class of flavonoids called flavanones and is found mainly in citrus fruit.Citation15 A number of researchers have examined the antioxidant activity and radical scavenging, anti-inflammatory, antiallergic, antihypertensive, antimicrobial, hypolipidemic, anticarcinogenic, and vasodilatory properties of HDN.Citation16–Citation19 Although, it has been reported that HDN is effective against disease and chemically induced toxicity, the effects on GEN-induced nephrotoxicity have not been investigated to date. Therefore, the aim of the present study was to evaluate the possible protective effects of HDN on GEN-induced nephrotoxicity in male Wistar albino rats. The structure of HDN is given in .
Materials and methods
Drugs and chemicals
HDN was obtained from Sigma Chemical Company (St Louis, MO, USA) and GEN from Ranbaxy Laboratories Ltd (Mumbai, India). All other biochemicals and chemicals used in the study were of analytical grade purchased from Himedia Laboratories and Sisco Research Laboratories (Mumbai, India).
Animals
All the experiments were carried out with male Wistar albino rats weighing 180–200 g, obtained from the Central Animal House at the Rajah Muthiah Institute of Health Sciences (Annamalai University, Tamil Nadu, India). The rats were housed six per cage and maintained on a 12 hours day and night cycle at room temperature of 22°C. The animals were fed a commercial pellet diet (Hindustan Lever Ltd, Bangalore, India) and water ad libitum. The experiments using animals were conducted according to the guidelines of the Committee for the Purpose of Control and Supervision of Experiments on Animals (CPCSEA), New Delhi, India, and approved by the Animal Ethical Committee of Annamalai University (approval no.160/1999 CPCSEA).
Experimental design
HDN was suspended in normal saline (0.9% NaCl) and administered orally at a dose of 200 mg/kg (body weight) as recommended by Tirkey et al.Citation20 GEN was injected intraperitoneally (i.p.) in the animals at a dose of 100 mg/kg (body weight) which is the normal dose to induce nephrotoxicity.Citation1 The injections were carried out daily between 09:00 and 09:30 to minimize the circadian variation in GEN-induced nephrotoxicity.Citation21
The animals were assigned randomly to four groups containing six rats each:
Group I (control) rats treated with i.p. injection of physiological saline (0.5 ml/kg (body weight)) for 22 days; Group II (GEN) rats treated with i.p. injections of saline for the first 14 days and then GEN (100 mg/kg (body weight)) alone for the last 8 days; Group III (HDN) rats received HDN (200 mg/kg (body weight)) via daily intragastric intubations for the entire experimental period; and Group IV (HDN + GEN) rats received HDN (200 mg/kg (body weight)) orally for 22 days and GEN (100 mg/kg (body weight)) injected i.p. from 15 to 22 days. The schematic representation of the treatment schedule is given in .
Processing of blood and tissue samples
At the end of the experimental period, all animals were fasted overnight and sacrificed by cervical dislocation after anaesthetizing with ketamine hydrochloride (30 mg/kg (body weight); intramuscularly). The blood was collected by sinooccular puncture for the assay of serum parameters. Kidney tissues were excised immediately, rinsed in ice-chilled normal saline, decapsulated and divided longitudinally into two equal-sized pieces. One piece was placed in formaldehyde solution for routine histopathological examination by light microscopy, and the other was placed in physiological saline and stored at −40°C until assayed.
Parameters assessed for renal function
Body weight
The weight of the animals was measured before and after treatment.
Serum biochemical assays
Blood was collected in a dry and clean test tube and allowed to coagulate at ambient temperature for 30 minutes. Serum was separated by centrifugation at 2000 rpm for 10 minutes. The concentrations of urea, uric acid, and creatinine were analyzed in the serum of control and experimental animals. Measurements were carried out for urea by the method from Natelson et al.Citation22 for uric acid from Caraway,Citation23 and for serum creatinine using the alkaline picrate methodCitation24 with a diagnostic kit (Qualigens, Mumbai, India). TBARS were measured collectively as malondialdehyde (MDA), and the other end products of lipid peroxidation were determined spectrophotometrically.Citation25 Serum NO levels were measured after conversion of nitrate to nitrite by nitrate reductase, and nitrite was measured by using the Griess reaction, as described previously.Citation26 The serum lipid profile including total cholesterol, triglycerides, and free fatty acids were determined by using the methods from Zlatkis et al.,Citation27 Foster and Dunn,Citation28 and Falholt et al.,Citation29 respectively. Enzymatic antioxidants including superoxide dismutase (SOD) and glutathione peroxidase (GPx) activities were assayed using the methods from Sun et al.Citation30 and Paglia and ValentineCitation31 in rat erythrocytes. Reduced glutathione (GSH) concentration in rat erythrocytes was measured according to the method from Beutler et al.Citation32
Histopathological examination
The kidney tissues of the rats from all the groups were fixed in 10% formaldehyde. Then, they were dehydrated using ethanol and embedded in paraffin wax. Fine sections (3–5 µm thick) were cut using a microtome, mounted on glass slides and counter-stained with hematoxylin and eosin for light microscopic analysis using an Olympus BX51 microscope (DSS Imagetech Pvt. Ltd, Chennai, India). All histopathological changes were examined by a pathologist who was ignorant of the treatment groups. Renal histological damage such as tubular necrosis, hyaline casts, marginal localization of chromatin in the nuclei, mononuclear cell infiltration, intertubular haemorrhage, picnosis, atrophied glomeruli, and obliterative arteriolopathy were assessed with a score previously described as follows: slight (+), moderate (++), or severe (+++) changes.Citation8
Results
The GEN-treated group showed significantly higher kidney weights (P < 0.05) and significantly lower body weights (P < 0.05) in comparison with the control rats, whereas treatment with HDN significantly reversed the effects caused by GEN ().
Table 1. Effect of HDN on body weight (g) and kidney weight (g) of rats with/without GEN treatment
shows the levels of serum urea, uric acid, creatinine, MDA, and NO in the control and experimental groups. Serum creatinine, uric acid, urea, MDA, and NO levels were significantly increased (P < 0.05) in GEN-induced rats when compared with the controls. Pretreatment of rats with HDN significantly reduced the levels of serum urea, uric acid, creatinine, MDA, and NO (P < 0.05) when compared with the GEN-induced rats.
Table 2. Effect of HDN on urea, uric acid, creatinine, TBARS, and NO in the serum of control and GEN-treated rats
The effect of HDN on the concentrations of serum cholesterol, triglycerides, free fatty acids, SOD, GPx, and GSH levels in the control and experimental groups is illustrated in . The levels of serum cholesterol, triglycerides, and free fatty acids were significantly increased in GEN-induced nephrotoxic rats (P < 0.05), but were significantly decreased in the SOD, GPx activities, and GSH content (P < 0.05). The administration of HDN with the GEN injection resulted in significantly decreased serum cholesterol, triglycerides, and free fatty acids levels (P < 0.05), and increased SOD, GPx activities, and GSH content (P < 0.05) when compared with GEN alone.
Table 3. Effect of HDN on total cholesterol, free fatty acids, triglycerides, SOD, GPx, and GST in the control and GEN-treated rats
shows the photomicrographs of control rats with normal histological features in the cortex and medulla of rat kidney tissues. Rats treated with GEN show desquamation, degeneration, and vacuolization of proximal tubules, a huge number of hyaline casts in renal tubules, marginal localization of chromatin in the nuclei, mononuclear cell infiltration, intertubular haemorrhage, picnosis, atrophied glomeruli, and obliterative arteriolopathy (A–F). In rats treated with HDN and GEN, the renal tubules were normal with mild mononuclear cell infiltration, slight degenerative epithelial changes in glomeruli and tubules, and sporadic hyaline casts in tubules (A–C). The tubules and glomerular were normal among the groups treated with HDN alone (D). Thus, HDN reversed most of the histopathological alterations induced by GEN as seen from sections from the HDN + GEN-treated rats ().
Figure 3. Photomicrographs of rat kidney specimens stained with haematoxylin and eosin (magnification ×100) in the control group with normal histology. (A) cortex and (B) medulla.
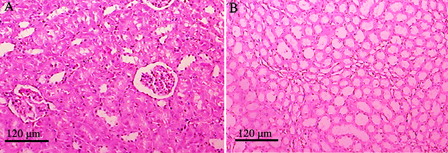
Figure 4. Histopathological view of rat renal sections in the GEN-treated group stained with hamatoxylin and eosin (magnification ×100). (A) Desquamation, degeneration, and vacuolization of proximal tubules (arrows); (B) huge numbers of hyaline casts in renal tubules (thick arrows) and marginal localization of chromatin in the nuclei (inset – thin arrows); (C) mononuclear cell infiltration (rectangled); (D) intertubular haemorrhage (blood filled spaces – thick arrow) and picnosis (thin arrow); (E) atrophied glomeruli (arrows); and (F) obliterative arteriolopathy.
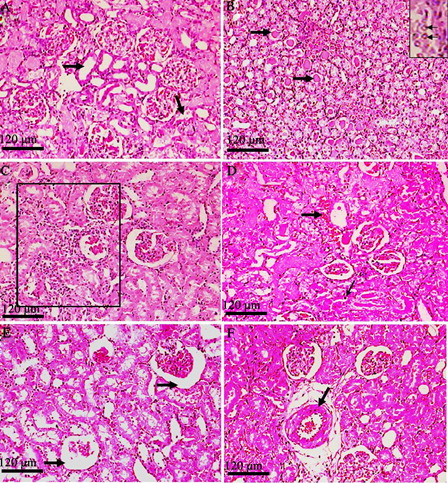
Figure 5. Histopathological view of rat renal sections in GEN + HDN (A–C) group and HDN alone (D) stained with haematoxylin and eosin (magnification ×100). (A) Sporadic hyaline casts in tubules (medulla); (B) mild mononuclear cell infiltration; (C) slight degenerative epithelial changes in glomeruli and tubules; and (D) normal structure of glomerulus and tubules.
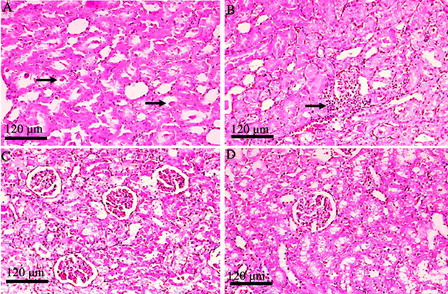
Table 4. Grading of histopathological alterations obtained from rat kidney tissues of different experimental groups
Discussion
Aminoglycoside antibiotics such as GEN are widely used as an agent for the treatment of life threatening Gram-negative bacterial infections. However, a major limitation of this drug is an increased risk for nephrotoxicity. An increasing body of evidence suggests that ROS are considered to be important mediators of GEN-induced acute renal failure.Citation6,Citation33 Several investigators have reported that a high level of ROS plays a role in a variety of renal diseases such as glomerulonephritis and tubulointerstitial nephritis, renal insufficiency, proteinuria, and hypertension.Citation34 It has been reported that several naturally occurring antioxidants from plants such as fruits, vegetables, and herbs are known to protect renal damage against GEN-induced toxicity.Citation33 In this study, we examined the effect of HDN, a citrus flavonoid, which is a potent antioxidant and free radical scavenger, against GEN-induced acute renal failure in male Wistar albino rats.
It has been suggested that antioxidant activities of flavonoids are due to their hydrogen-donating and free-radical scavenging properties.Citation35 Flavonoids can inhibit free radical formation and the propagation of free radical reactions through the chelation of metal ions. The free-radical scavenging activity of HDN may be attributed to the presence of 3′hydroxy and the 4′methoxy group on the aromatic B ring (), which donates hydrogen and an electron to neutralize the hydroxyl and superoxide free radicals.Citation36
The results of the present study confirm that GEN at a dose of 100 mg/kg (body weight) produces nephrotoxicity, as indicated by the increase in serum urea, uric acid, and creatinine levels. In addition, the increase in both serum creatinine and urea were correlated to the reduction in GFR, which indicates impairment to the glomerular functions. These alterations in the biochemical parameters correlate closely with the renal histopathological results (). Similar observations of GEN-induced nephrotoxicity have been reported previously.Citation4,Citation7
In the earlier phase of renal damage, the serum creatinine levels are a more significant parameter than the urea levels.Citation37 Kopple et al.Citation5 reported that in humans, serum creatinine might be a more accurate measure of the GFR than creatinine clearance. The protective effect of HDN on serum urea and creatinine concentration (at reduced levels) can be attributed to its antioxidant properties, as it has been reported that ROS may be involved in the impairment of GFR.Citation4
GEN-induced rats showed increased lipid peroxidation in the renal tissues as indicated by the higher levels of TBARS (MDA and other lipid peroxidation products). This increase results in decreased levels of polyunsaturated fatty acids, which in turn serve as a substrate for free radical attack.Citation38 In addition, the significantly lower levels of TBARS in the serum of the HDN and HDN + GEN groups, as compared with the GEN group, indicate attenuation of lipid peroxidation. This was probably due to less damage by the oxygen-free radicals when HDN was present. The involvement of oxygen-free radicals in renal tissue injuries is well established.Citation39 The results of the present study revealed that GEN significantly increases NO and TBARS in serum (P < 0.05), suggesting that oxidative stress and NO production when induced by GEN, play a role in GEN-induced renal damage as previously reported.Citation1,Citation40 The correlation between oxidative stress and nephrotoxicity has been well demonstrated in many investigational animal models.Citation1,Citation14
Various animal experiments have shown that a certain basal amount of NO may be significant for maintaining normal renal function.Citation41 Previous studies have demonstrated that excessive production of NO and its metabolite, peroxynitrite, plays a major role in oxidative stress and tissue damage in the pathophysiology of acute renal failure.Citation42 Elevated levels of NO are known to hamper DNA repair proteins, thereby inhibiting the ability of the cell to restore damaged DNA.Citation43 Data from the present study revealed that GEN significantly increases the NO level in rat serum when compared with the control group, suggesting that GEN administration may lead to the induction of inducible NO synthase, resulting in an increased production of NO, which then leads to the formation of toxic peroxynitrite.Citation44 Oral supplementation of HDN to the GEN-induced group significantly decreased the NO level, which suggests that HDN may block the occurrence of NO and thereby acts with a supportive effect on the antioxidant system. It has been demonstrated that flavonoids inhibit the expression of isoforms of inducible NO synthase, cyclooxygenase, and lipooxygenase, which are responsible for the production of NO, prostanoids, and leukotrienes, as well as inflammatory mediators such as cytokines, chemokines, or adhesion molecules.Citation45
GEN-induced nephrotoxicity was associated with low activities of GPx and SOD levels in rat erythrocytes, indicating GEN-induced oxidative damage. The increased production of ROS in GEN-induced nephrotoxicity may cause inactivation of these antioxidant enzymes.Citation1,Citation46 Flavonoids are believed to produce beneficial protective effects either by acting against oxidative damage or by activation of the antioxidant defense enzyme system.Citation47 One of the citrus flavonoids, HDN, exerts a beneficial effect by increasing the activities of GPx and SOD content, and this may be due to the antioxidant property of HDN.Citation20 In addtion, the levels of GSH in rat erythrocytes were significantly reduced after the GEN injection compared with the control group. The decrease in the concentration of GSH after the GEN treatment might be due to the increased consumption of GSH in the non-enzymatic removal of oxygen radicals. Furthermore, GSH reduces peroxides and maintains protein thiols in the reduced state.
The present study demonstrates a significant elevation in serum lipid profile (cholesterol, triglycerides and free fatty acids) in nephrotoxic rats which could be attributed to alteration in lipid profile and modifications in lipoprotein levels.Citation48,Citation49 Oral administration of HDN (200 mg/kg (body weight)) significantly restored the lipid profile to normal levels, and showed its antihyperlipidaemic property. This observation confirms the previous report by Monforte et al.Citation50
According to the histopathological findings, GEN-treated rats showed extreme tubular necrosis, basal membrane interruption, desquamated epithelial cells, picnotic nuclei, and damaged glomerular structure in their kidneys. Similar structural changes in rat kidney tissues during GEN-induced nephrotoxicity and the protection provided by various plant flavonoids have already been reported by Kumar et al.Citation51 and Al-Majed et al.Citation52 Furthermore, GEN-treated rats showed severe tubular necrosis which might be due to enhanced accumulation of GEN for a longer period in proximal tubular cells, and may induce free radicals which also contribute to cell injury.Citation1 Treatment with HDN significantly reduced the tubular necrosis by enhancing the cellular antioxidants.
Conclusion
The present study indicated that HDN markedly enhanced the activities of GSH-Px and SOD antioxidant enzymes and reduced the elevated levels of TBARS, NO, and the lipid profile, indicating that HDN treatment decreases oxidative stress through its antioxidant properties. However, further investigations on the mechanism of action of HDN are required to strengthen its usefulness in future clinical applications for patients with renal failure.
References
- Parlakpinar H, Sahna E, Acet A, Mizrak B, Polat A. Effect of caffeic acid phenethyl ester (CAPE) on myocardial ischemia-reperfusion induced apoptotic cell death. Toxicol 2005;207:169–77.
- Edson RS, Terrell CL. The aminoglycosides. Mayo Clin Proc 1999;74:519–28.
- Begg EJ, Barclay ML. Aminoglycosides – 50 years on. Br J Clin Pharmacol 1995;39:597–603.
- Pedraza-Chaverri J, Maldonado PD, Medina-Campos ON, Olivares-Corichi IM, Granados-Silvestre MA, Hernandez-Pando R. Garlic ameliorates gentamicin nephrotoxicity: relation to antioxidant enzymes. Free Radic Biol Med 2000;29:602–11.
- Kopple JD, Ding H, Letoha A, Ivanyi B, Qing DP, Dux L. L-Carnitine ameliorates gentamicin induced renal injury in rats. Nephrol Dial Transplant 2002;17:2122–31.
- Walker PD, Barri Y, Shah SV. Oxidant mechanisms in gentamicin nephrotoxicity. Ren Fail 1999;21:433–42.
- Cuzzocrea S, Mazzon E, Dugo L, Serraino I, Di Paola R, Britti D, et al. A role for superoxide in gentamicin-mediated nephropathy in rats. Eur J Pharmacol 2002;450:67–76.
- Abdel-Raheem IT, Abdel-Ghany AA, Mohamed GA. Protective effect of quercetin against gentamicin-induced nephrotoxicity in rats. Biol Pharm Bull 2009;32:61–7.
- Ozbek E, Turkoz Y, Sahna E, Ozugurlu F, Mizrak B, Ozbek M. Melatonin administration prevents the nephrotoxicity induced by gentamicin. BJU Int 2000;85:742–6.
- Kalayarasan S, Prabhu PN, Sriram N, Manikandan R, Arumugam M, Sudhandiran G. Diallyl sulfide enhances antioxidants and inhibits inflammation through the activation of Nrf2 against gentamicin-induced nephrotoxicity in Wistar rats. Eur J Pharmacol 2009;606:162–71.
- Sayed-Ahmed MM, Nagi MN. Thymoquinone supplementation prevents the development of gentamicin-induced acute renal toxicity in rats. Clin Exp Pharmacol Physiol 2007;34:399–405.
- Ali BH, Al-Wabel N, Mahmoud O, Mousa HM, Hashad M. Curcumin has a palliative action on gentamicin-induced nephrotoxicity in rats. Fundam Clin Pharmacol 2005;19:473–7.
- Vardi N, Parlakpinar H, Ozturk F, Acet A. Gentamicin-induced nephrotoxicity and protective effect of caffeic acid phenethyl ester in rats. Fundam Clin Pharmacol 2005;19:173–7.
- Morales AI, Rodriquez-Barbero A, Vicente-Sanchez C, Mayoral P, Lopez-novoa JM, Perez-Barriocanal F. Resveratrol inhibits gentamicin induced mesangial cell contraction. Life Sci 2006;78:2373–7.
- Wilmsen PK, Spada DS, Salvador M. Antioxidant activity of the flavonoid hesperidin in chemical and biological systems. J Agr and Food Chem 2005;53:4757–61.
- Emin JA, Oliveira AB, Lapa AJ. Pharmacological evaluation of the anti-inflammatory activity of a citrus bioflavonoid, hesperidin, and the isoflavonoids duartin and claussequinone in rats and mice. J Pharm Pharmacol 1994;46:118–22.
- Suarez J, Herrera MD, Marhuenda E. In vitro scavenger and antioxidant properties of hesperidin and neohesperidin dihydrochalcone. Phytomedicine 1998;5:469–73.
- Garg A, Garg S, Zaneveld LJ, Singla AK. Chemistry and pharmacology of the citrus bioflavonoid hesperidin. Phytother Res 2001;15:655–69.
- Tommasini S, Calabro ML, Stancanelli R, Donato P, Costa C, Catania S, et al. The inclusion complexes of hesperetin and its 7-rhamnoglucoside with (2-hydroxypropyl)-b-cyclodextrin. J Pharmaceut Biomed 2005;39:572–80.
- Tirkey N, Pilkhwal S, Kuhad A, Chopra K. Hesperidin, a citrus bioflavonoid, decreases the oxidative stress produced by carbon tetrachloride in rat liver and kidney. BMC Pharmacol 2005;5:2–7.
- Pariat C, Courtois P, Cambar J, Prior A, Bouquet S. Circadian variation in renal toxicity of gentamicin in rats. Toxicol lett 1988;40:175–82.
- Natelson S, Scott ML, Beffa C. A rapid method for the estimation of urea in biologic fluids. Am J Clin Pathol 1951;21:275–81.
- Caraway WT. Uric acid. In: , Seligson D, (ed.) Standard methods of clinical chemistry. New York: Academic press; 1963. p. 239–47.
- Owen JA, Iggo B, Scandrett FJ, Stewart CP. The determination of creatinine in plasma or serum, and in urine; a critical examination. Biochem J 1954;58:426–37.
- Yagi K. Assay of blood plasma or serum. Methods Enzymol 1984;105:328–31.
- Cortas NK, Wakid NW. Determination of inorganic nitrate in serum and urine by a kinetic cadmium-reduction method. Clin Chem 1990;36:1440–3.
- Zlatkis A, Zak B, Bogle GJ. A method for the determination of serum cholesterol. J Clin Med 1953;41:486–92.
- Foster LB, Dunn RT. Stable reagents for determination of serum triglycerides by colorimetric hantzsch condensation method. Clin Chem. 1973;19:338–40.
- Falholt K, Lund B, Falholt W. An easy colorimetric method for routine determination of free fatty acids in plasma. Clin Chim Acta 1973;46:105–11.
- Sun Y, Oberley LW, Ying L. A simple method for clinical assay of superoxide dismutase. Clin Chem 1988;34:497–500.
- Paglia DE, Valentine WN. Studies on the quantitative and qualitative characterisation of erythrocyte glutathione peroxidase. J Lab Clin Med 1967;70:158–69.
- Beutler E, Duron O, Kelly BM. Improved method for the determination of blood glutathione. J Lab Clin Med 1963;61:882–8.
- Karahan I, Atessahin A, Yilmaz S, Ceribasi AO, Sakin F. Protective effect of lycopene on gentamicin induced oxidative stress and nephrotoxicity in rats. Toxicol 2005;215:198–204.
- Martin CJ, Goeddeke-Merickel CM. Oxidative stress in chronic kidney disease. Nephrol Nurs J 2005;32:683–5.
- Van Acker SA, Van den Berg DJ, Tromp MN. Structural aspects of antioxidant activity of flavonoids. Free Rad Biol Med 1996;20:331–42.
- Cao G, Sofic E, Prior RL. Antioxidant and prooxidant behaviour of flavonoids: structure–activity relationships. Free Radic Biol Med 1997;22:749–60.
- Erdem A, Gundogan NU, Usubutun A, Kilinc K, Erdem SR, Kara A. The protective effect of taurine against gentamicin-induced acute tubular necrosis in rats. Nephrol Dial Transplant 2000;15:1175–82.
- Hayashi T, Watanabe Y, Kumano K, Kitayama R, Yasuda RT, Saikawa I, et al. Protective effect of piperacillin against nephrotoxicity of cephaloridine and gentamicin in animals. Antimicrob Agents Chemother 1988;32:912–8.
- Maestro DRF. An approach to free radicals in medicine and biology. Acta Physiol Scand 1980;92:153–9.
- Yaman I, Balikci E. Protective effects of Nigella sativa against gentamicin-induced nephrotoxicity in rats. Exp Toxicol Pathol 2010;62:183–90.
- Fujihara CK, Sena CR, Malheiros DM, Mattar AL, Zatz R. Short-term nitric oxide inhibition induces progressive nephropathy after regression of initial renal injury. Am J Physiol Renal Physiol 2006;290:632–40.
- Passauer J, Pistrosch F, Bussemaker E. Nitric oxide in chronic renal failure. Kidney Int 2005;67:1665–7.
- Watanabe K, Hess A, Bloch W, Michel O. Expression of inducible nitric oxide synthase (iNOS/NOS II) in the vestibule of guinea pigs after the application of cisplatin. Anticancer Drugs 2000;II:29–32.
- Amore A, Coppo R. Role of apoptosis in pathogenesis and progression of renal diseases. Nephron 2000;862:99–104.
- Tunon MJ, Garcia-Mediavilla MV, Sanchez-Campos S, Gonzalez-Gallego J. Potential of flavonoids as anti-inflammatory agents: modulation of proinflammatory gene expression and signal transduction pathways. Curr Drug Metab 2009;10:256–71.
- Polat A, Parlakpinar H, Tasdemir S, Colak C, Vardi N, Ucar M. Protective role of aminoguanidine on gentamicin-induced acute renal failure in rats. Acta Histochem 2006;108:365–71.
- El-Sayed el SM, Abo-Salem OM, Abd-Ellah MF, Abd-Alla GM. Hesperidin, an antioxidant flavonoid, prevents acrylonitrile-induced oxidative stress in rat brain. J Biochem Mol Toxicol 2008;22:268–73.
- Rashid F, Kaleem M, Sheema S, Bano B. Comparative effect of olive oil and fish oil supplementation in combating gentamicin induced nephrotoxicity in rats. Indian J Clin Biochem. 2005;20:109–14.
- Devbhuti P, Sengupta C, Saha A. Studies on amikacin induced lipid profile and peroxidation parameters and their control with ascorbic acid. Int J of Curr Pharmaceut Res. 2010;2:76–81.
- Monforte MT, Trovato A, Kirjavainen S, Forestieri AM, Galati EM, Lo Curto RB. Biological effects of hesperidin, a Citrus flavonoid. (note II): hypolipidemic activity on experimental hypercholesterolemia in rat. Farmaco 1995;50:595–9.
- Kumar KV, Shifow AA, Naidn MUR, Ratnaker KS. Carvedilol: a beta-blockerwith antioxidant property protects against gentamicin-induced nephrotoxicity in rats. Life Sci 2000;66:2603–11.
- Al-Majed AA, Mostafa AM, Al-Rikabi AC, Al-Shabanah OA. Protective effects of oral arabic gum administration on gentamicin-induced nephrotoxicity in rats. Pharmacol Res 2002;46:445–51