Abstract
Objectives
The objective of this study was to investigate the effects of catechin and epicatechin on the activity of the endogenous antioxidant enzymes superoxide dismutase (SOD) and glutathione peroxidase (GPx) (as well as the total antioxidant capacity (TAC)) of rats after intra-peritoneal (i.p.) administration.
Methods
Twenty-four Wistar rats were randomly divided into two groups: the experimental group which was administered daily with a 1:1 mixture of epicatechin and catechin at a concentration of 23 mg/kg body weight for 10 days and the control group which was injected daily with an equal amount of saline. Blood and urine samples were collected before and after the administration period, as well as 10 days after (follow-up).
Results
Intra-peritoneal administration of catechins led to a potent decrease in GPx levels and a significant increase in SOD levels. TAC was significantly increased in plasma and urine. Malonaldehyde levels in urine remained stable. In the animals treated with catechins, SOD activity showed a moderate negative correlation with GPx activity.
Discussion
Boosting the activity of the antioxidant enzymes could be a potential adjuvant approach for the treatment of the oxidative stress-related diseases.
Introduction
Oxidative stress is the biomolecular damage caused by free radical attack against components of living organisms.Citation1 Some of the most important physiological conditions or diseases, related to increased cellular oxidative stress, are aging,Citation2 obesity,Citation3 hypertension,Citation4 arteriosclerosis,Citation4 type 2 diabetes,Citation4 the metabolic syndrome,Citation5 rheumatoid arthritis,Citation6 and cancer.Citation7
Against oxidative stress, the human organism deploys an interactive network of antioxidants, which can be categorized into four groups (four lines of defense) according to their function.Citation8 The first line of defense consists of preventive antioxidants, which suppress the formation of free radicals (e.g. antioxidant enzymes). The second line of defense consists of antioxidants that scavenge free radicals by suppressing chain initiation and/or stopping multiple chain reactions (e.g. vitamin C, uric acid, albumin, and vitamin E). The third category includes repair antioxidants and de novo antioxidants (e.g. proteinases and nucleases). Finally, the fourth line of defense is adaptive, where the signal for the production and reactions of free radicals causes the formation and transfer of appropriate antioxidants in the correct location. The antioxidant enzymes primarily account for the intracellular defense (located in the mitochondria and the cytoplasm of the cells), where low-molecular antioxidants protect various components mainly in the extracellular fluids (e.g. plasma).
In many cultures around the world (such as Persian, Greek, Asian, Indian, etc.), herbal extracts, rich in phenolic compounds, have been and are being used as treatment for diseases correlated to oxidative stress.Citation9 Natural sources for these compounds include fruits and vegetables such as grapes, raspberries, onions, tomatoes, red wine, tea, etc.Citation10 Their antioxidant properties are well defined by in vitro experimentsCitation11,Citation12 and there are indications of their beneficial effects in the prevention of diseases, when they are part of the diet.Citation13 Flavonoids are free-radical scavengers acting as hydrogen- or electron-donating agents. This property is mainly attributed to their ideal structural chemistry. For example, the structural arrangements of catechins (specifically the ortho 3′,4′-dihydroxy moiety in the B ring) gives them a greater antioxidant activity when compared with other molecules, such as vitamin C and E, or other flavonoids, such as rutin, apigenin, naringenin, etc.Citation10 Moreover, flavonoids can exert many other biological effects (e.g. inhibit enzymatic activities of cyclooxygenases, lipooxygenases, metalloproteinases, and NADPH oxidases) that may be more important in vivo than their antioxidants effects.Citation14
Specifically, in the last 10 years, a strong interest has been raised in the use of flavonoids and their derivatives for the therapeutic use, such as anti-inflammatory, anticancer, anti-ischemic, and antithrombotic components. Besides from presenting potent antioxidant properties in vitro, these compounds have also the ability to modulate the activity of the antioxidant defense enzymes such as superoxide dismutase (SOD), glutathione peroxidase (GPx), and catalase.Citation15–Citation19 Flavonoids could be used alone or in combination with synthetic drugs or antibiotics to produce a new generation of phytopharmaceuticals,Citation20 which could have prominent applications in human diseases accompanied by free radical injury. Based on this concept, intra-peritoneal (i.p.) or intravenous administration of these compounds can provide evidence for their future use, as drugs against oxidative stress and thus against all the chronic diseases related to oxidative stress.
The aim of this study was to evaluate the effects of catechin and epicatechin, as in vivo modulators, on the activity of the antioxidant enzymes, SOD, and GPx, as well as on the functionality of the antioxidant defense system.
Methods and materials
Chemicals and apparatus
(−)-Epicatechin, (+)-catechin, SOD assay kit, GPx assay kit, 2-thiobarbituric acid, 3-methylbutanol, ammonium dichromate, alpha-tocopherol, and hydrogen peroxide of high purity were purchased from Sigma-Aldrich (St Louis, MO, USA). 1,1,3,3-tetraethoxypropane, phosphoric acid, 3-methylbutanol, and hydrogen peroxide of high purity were purchased from Fluka (St Louis, MO, USA). SOD, GPx assay kit, malonaldehyde (MDA), as well as total antioxidant capacity (TAC) measurements were performed on a UV/Vis microplate and cuvette spectrophotometer (Thermo Fischer Scientific Inc. Waltham, MA, USA). Analysis of MDA was performed by liquid chromatography-mass spectrophotometry (Shimadzu LC-MS 2010EV, Shimadzu Scientific Instruments Inc., Columbia, MD, USA).
Animals
The experiment was conducted in 24 adult female Wistar rats (14 weeks old–3.5 months, average body weight 197 ± 12 g). Experiments on animals were handled with human care in accordance with the National Institutes of Health guidelines and the European Union directive for the care and the use of laboratory animals (Greek presidential decree no. 160 1991) and according to the permission number 20EEP02 (University of Ioannina). Animals were maintained in metabolic cages, one animal per cage, at an ambient temperature of 25°C, under a photoperiod of 12 hours of light and 12 hours of darkness. Animals were provided ad libitum with rat chow (Viozois S.A., Animal Feed Company of Epirus, Greece) and water.
In vivo treatment
The animals were randomly divided, into two groups (12 animals per group) and received treatment for 10 consecutive days. The control animals (control group-CG) were given a single dose of 1 cm3 saline (NaCl 0.9%) for the experimental period (Days 1–10). Animals in the experimental group (EG) were given 1 cm3 mixture of catechin and epicatechin (Days 1–10).
Catechins were diluted in sterile ddH2O for i.p. injection. Each dose was composed of 2.25 mg of catechin and 2.25 mg of epicatechin (ratio 1:1 catechin and epicatechin), which corresponds to 23 mg of catechins per kg of body weight.
Rats were left for acclimatization in the metabolic cages for 5 days before the start of the experiment (Day 0). The amount of food consumption and water intake as well as urine output and fecal weight were monitored daily. Rats were daily weighted and urine and blood samples (2 ml from the jugular vein of the rats) were collected at Day 0, 11, and 22. Blood samples were centrifuged, at 400 × g for 30 minutes, so that plasma and red blood cells (RBCs) be separated. All samples were stored at −80°C until used.
All treatments were given in a single i.p. dose, between 11.00 and 12.00 hours each day of the administration period. Animals were left for monitoring in the metabolic cages, for 10 more days after treatment (Days 12–21). The total experimental time was set at 22 days.
Biochemical analysis
Estimation of TAC in plasma, RBCs, and urine was performed according to the Blue CrO5 assay.Citation21 SOD and GPx activity in RBCs was determined spectrophotometrically using the Sigma-Biochemica 19160 kit, and GPx Cellular Activity Assay kit, CGP1, respectively, (Sigma-Aldrich Ltd). MDA levels, in urine, were determined by LC-MS of the adduct obtained with thiobarbituric acid (TBA) reagent, based on the methodology proposed by Hong et al.Citation22 Briefly, MDA levels were measured by LC-MS of the adduct obtained with TBA reagent (Sigma-Aldrich T5500) as a 23 mmol/l solution in water. A 10 mmol/l stock standard of MDA was prepared by dissolving 247 µl of 1,1,3,3-tetraethoxypropane in 100 ml of aqueous ethanol (40% ethanol by volume). TBA–MDA adducts were prepared in glass tubes with a polypropylene stopper. In each tube, 300 µl of phosphoric acid (0.5 M) was mixed with 50 µl of urine and 150 µl of TBA reagent. The tubes were then heated to 95°C and maintained at that temperature for 1 hour. Thereafter, the tubes were chilled on ice for 5 minutes. Methanol (500 µl) was added and the tubes were centrifuged at 5000 × g for 5 minutes. The samples were then transferred to glass autosampler vials and 20 µl aliquots were analyzed by LC-MS.
Statistical analysis
Data are expressed as mean ± SD. The statistical significance of difference, between data means, was determined by one-way analysis of variance and Student's t-test was used for statistical evaluation of differences between groups, at different time intervals. Bivariate correlation and linear regression analysis was also performed (SPSS version 17.0, Statistical Package for the Social Sciences software, SPSS, Chicago, IL, USA). P values, P < 0.05, were considered as significant.
Results
The activity of the antioxidant enzymes, SOD and GPx, are presented in . The activity of SOD was significantly increased (P < 0.05) in the EG at the end of the administration period and, moreover, the activity remained elevated and significantly increased at the end of the experiment at Day 22 (P < 0.05). At Day 11, GPx activity was significantly reduced (P < 0.05) by almost 35% compared to Day 0. The reduction of GPx activity was continued until the end of the experiment (Day 22, 51% reduction). The activity of GPx was also significantly reduced, when compared with control on Day 11 and on Day 22 (by 41 and 64%, respectively).
Figure 1. Antioxidant enzymes activities in control and EG. Enzyme activity for GPx is expressed as U/g hemoglobin and for SOD as % inhibition rate. aSignificantly different from Day 0, P < 0.05. bSignificantly different between groups, P < 0.05. Data are expressed as mean ± SD. GPx, glutathione peroxidase; SOD, superoxide dismutase.
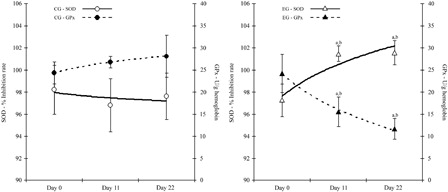
The TAC of plasma, RBCs, and urine is presented in . TAC in RBCs of the rats was not significantly altered by epicatechin and catechin administration, throughout the experimental period but TAC in plasma was significantly increased (15%) on Day 11 (P < 0.05). Levels returned to normal at Day 22. In the control group, TAC in plasma, RBCs, and urine remained stable, in all time points measured. On the other hand, TAC in urine showed a steep and significant increase from Day 0 (P < 0.05), reaching 29% at Day 11. Furthermore, the levels of TAC in urine were continued to be significantly elevated at Day 22 at 35%, compared to Day 0 (P < 0.05).
Table 1. TAC and urine MDA concentration in CG and EG
MDA levels were determined, by LC-MS, in urine of the rats. As shown in , catechins administration had no effect on MDA levels, which remained stable during the course of the experiment. No significant difference, from control or from Day 0, was observed at any time point.
Animals’ weight as well as metabolic factors such as food consumption, water intake, fecal weight, and urine output, was also measured. Epicatechin and catechin administration did not exert any significant influence on final body weight. Thus, body weight did not change compared to that of control rats. The cumulative food intake in the EG, between the administration period, and the monitoring period did not differ ().
Table 2. Food consumption, water intake, urine output, and fecal weight in the CG and EG during the experimental period (Days 0–21)
The EG, SOD activity showed a moderate negative correlation (r = −0.629, P < 0.001) with GPx activity. Linear regression demonstrated a significant negative relationship (F(1, 42) = 27.487, P < 0.001). The SOD activity increased approximately by 1.4% for each 10 U/g hemoglobin decrease of GPx activity ().
Discussion
The objective of this study was to determine whether i.p. administration of catechin and epicatechin could affect the activity of the antioxidant enzymes, SOD and GPx, as well as the TAC in RBCs, blood plasma, and urine.
The antioxidant enzymes are agents that promote reactions for the removal of reactive species (e.g. O2•,.H2O2, etc.). They constitute the first line of defense against oxidative stress. In conditions of increased oxidative stress, the upregulation of the enzyme activity or even, a possible protection of the enzymes’ substrate could be of great importance. Oxidative stress disturbing homeostasis can be resolved by the application of catechins and epigallocatechin gallate (EGCG)Citation18 and there is growing evidence that, the protection, offered by flavonoids and their in vivo metabolites, is not mediated primarily by H-donating antioxidant processes, but is likely to be partly mediated through specific actions, within signaling pathways.Citation23
Various in vitro studies provide evidence of the ability of catechins to regulate the enzymatic activity. Treatment with catechin markedly increases SOD activity with similar changes being observed in the gene expression of SOD in rat brain astrocytes.Citation15 It also enhances the activity of antioxidant enzymes, catalase, and SOD to scavenge intracellular reactive oxygen species and repair oxidized DNA, in murine microglia cells.Citation24 When compared with other natural compounds (sodium ascorbate, l-carnitine, EGCG, genistein, paraquat, quercetin, 12-O-tetradecanoylphorbol-13-acetate, and trolox) catechin fails to increase the relatively SOD1 (copper/zinc), GPx1 (cytosolic GPx), and CAT (catalase) promoter activity in Chinese hamster lung fibroblast cells (V79 cells).Citation25 EGCG upregulates heme oxygenase-1 via the phosphatidylinositol 3-kinase/Akt and ERK pathways, in the endothelial cellsCitation26 and this was also observed by Na et al.,Citation27 in human breast epithelial cells (MCF10A). According to the authors, treatment of MCF10A cells with EGCG induces the expression of glutamate–cysteine ligase, manganese superoxide dismutase (MnSOD), and heme oxygenase-1 (HO-1) through the release of Nrf2.
The in vivo data are scarce. Agarwal et al.,Citation18 addressed the effects of crude green tea extract (a commercially available catechin powder) in reducing the oxidative stress seen in patients of Acid Fast Bacilli (AFB) positive pulmonary tuberculosis and observed a significant decrease in catalase and GPx activity and a significant increase in SOD activity. In fruit flies supplemented with green tea catechins in their diet, real-time polymerase chain reaction analysis indicated that the genes of copper–zinc containing SOD (CuZnSOD), manganese containing SOD (MnSOD), and catalase, were upregulated.Citation17 Various mixtures of tea polyphenols (mainly catechins) were evaluated against radiation-induced damage in mice. Their administration orallyCitation28 or intragastricallyCitation29 significantly decrease radiation-induced lipid peroxidation (MDA levels) and elevates endogenous antioxidant enzymes (SOD). Due to the different effects of the various mixtures tested the authors concluded that multiple or synergistic mechanisms must be involved in the regulation of the enzyme activity and the decrease in MDA levels.
Although SOD activity was significantly increased after the administration period, MDA levels remained stable in both groups in our study. TAC of urine in the EG was elevated probably due to the increased presence of catechins and their metabolites.
Even though GPx activity is decreased after catechins administration TAC is not being affected. Agarwal et al.,Citation18 proposed that, GPx activity reduction was due to the shielding of glutathione (GSH), which is the GPx substrate, by crude catechin extract against free radicals. Kumar et al.Citation30, showed that incubation of erythrocytes (from normal and hypertensive subjects) with (−)-epicatechin in various doses, demonstrates a protective effect on GSH oxidation; this effect being more pronounced in hypertensive patients. We observed an inverse moderate relationship in the modulation of SOD and GPx activity (rCitation2 = −0.629, P < 0.001). It is possible that the organism in order to maintain homeostasis of the antioxidant defense system, after the application of catechins and the upregulation of SOD activity, activated the downregulation of GPx activity and thus restored the redox balance. There is also evidence of in vitro H2O2 generation by oxidation of compounds (polyphenols, green tea extracts, ascorbate, etc.) added in the cell culture media,Citation14 but there are no data from in vivo studies supporting the same. Moreover, an excessive generation of H2O2 would reduce the TAC of the rats’ plasma; instead, a significant increase was recorded in our study. More research is needed to define the exact effect of catechins on GPx gene expression and activity.
Catechin and epicatechin administration modulated the activity of SOD and GPx but the overall TAC of the RBCs and of the rat's plasma remained stable. Catechins are considered as potent antioxidants and many of their biological actions have been attributed to that. It would have been expected that since catechins are potent antioxidants in vitro, they would have exerted their classical hydrogen-donating antioxidant activity leading to an increase in TAC; as it is seen in the TAC of plasma. The modulation of the enzymes activity may provide evidence that, catechins exert their primary antioxidant activity by specific action within specific molecular pathways, rather than as scavengers of free radicals.
No statistical significant differences were recorded in the mean food consumption, water intake, urine output, and fecal weight in the CG and EG during the administration period (Days 0–10) and the monitoring period (Days 12–21). Urine output as well as fecal weight was higher in the EG and statistical significant from the CG in both periods, although catechins administration did not result in any significant change of the initial values recorded. Rats in the CG slightly increased their water intake which also affected their urine output. Overall, the water equilibrium (water intake-urine output) remained stable in both groups at all time points.
Oxidative stress is a prominent feature of many acute and chronic diseases and even of the normal aging process. The normal function of the antioxidant enzymes guarantees the preservation of cell integrity and thus they can be considered as potential therapeutic targets of oxidative stress-related diseases. Various antioxidants are available for therapeutic use but most of them have failed in clinical studies of diseases correlated with oxidative stress.Citation31 Our results suggest that catechins exert their activity not only by H-donating antioxidant processes but likely through mechanisms and pathways that directly or indirectly regulate the expression of the enzymatic antioxidants. The understanding of these pathways could be important, in developing pharmacological strategies against oxidative stress-related diseases.
Acknowledgements
The author gratefully acknowledges the assistance of Dr Christodoulos Sioutis, bacteriologist for his assistance on blood analysis, Dr Ioannis Zioris, chemist, for his technical and scientific assistance in LC-MS analysis, and Mr Dimitris Palitskaris for reviewing and editing the manuscript.
References
- Halliwell B, Whiteman M. Measuring RS and oxidative damage in vivo and in cell culture: how should you do it and what do the results mean? Br J Pharamacol 2004;142:231–5.
- Kregel KC, Zhang HJ. An integrated view of oxidative stress in aging: basic mechanisms, functional effects, and pathological considerations. Am J Physiol Regul Integr Comp Physiol 2007;292:R18–36.
- Keaney JF, Larson MG, Vasan RS, Wilson PWF, Lipinska I, Corey D, et al. Obesity and systemic oxidative stress: clinical correlates of oxidative stress in the Framingham study. Arterioscler Thromb Vasc Biol 2003;23:434–9.
- Ceriello A, Motz E. Is oxidative stress the pathogenic mechanism underlying insulin resistance, diabetes, and cardiovascular disease? The common soil hypothesis revisited. Arterioscler Thromb Vasc Biol 2004;24:816–23.
- Roberts CK, Sindhu KK. Oxidative stress and metabolic syndrome. Life Sci 2009;84:705–12.
- Hitchon CA, El-Gabalawy HS. Oxidation in rheumatoid arthritis. Arthritis Res Ther 2004;6:265–78.
- Valko M, Rhodes CJ, Moncol J, Izakovic M, Mazur M. Free radicals, metals and antioxidants in oxidative stress-induced cancer. Chem Biol Interact 2006;160:1–40.
- Noguchi N, Watanabe A, Shi H. Diverse functions of antioxidants. Free Radic Res 2000;33:809–17.
- Kaefer CM, Milner JA. The role of herbs and spices in cancer prevention. J Nutr Biochem 2008;19:347–61.
- Kähkönen MP, Hopia AI, Vuorela HJ, Rauha JP, Pihlaja K, Kujala TS, et al. Antioxidant activity of plant extracts containing phenolic compounds. J Agric Food Chem 1999;47:3954–62.
- Rice-Evans CA, Miller NJ, Paganga G. Antioxidant properties of phenolic compounds. Trends Plant Sci 1997;2:152–9.
- Natsume M, Osakabe N, Yasuda A, Baba S, Tokunaga T, Kondo K, et al. In vitro antioxidative activity of (2)-epicatechin glucuronide metabolites present in human and rat plasma. Free Radic Res 2004;38:1341–8.
- Ortega RM. Importance of functional foods in the mediterranean diet. Public Health Nutr 2006;9:1136–40.
- Halliwell B. Are polyphenols antioxidants or pro-oxidants? What do we learn from cell culture and in vivo studies? Arch Biochem Biophys 2008;476:107–12.
- Chan P, Cheng JT, Tsai JC, Lien GS, Chen FC, Kao PF, et al. Effect of catechin on the activity and gene expression of superoxide dismutase in cultured rat brain astrocytes. Neurosci Lett 2002;328:281–4.
- Ying CJ, Sun XF, Zhang SL, Zhang XP, Mao LM, Zuo XZ, et al. ROS related enzyme expressions in endothelial cells regulated by tea polyphenols. Biomed Environ Sci 2004;17:33–9.
- Li YM, Chan HYE, Huang Y, Chen ZY. Green tea catechins upregulate superoxide dismutase and catalase in fruit flies. Mol Nutr Food Res 2007;51:546–54.
- Agarwal A, Prasad R, Jaina A. Effect of green tea extract (catechins) in reducing oxidative stress seen in patients of pulmonary tuberculosis on DOTS Cat I regimen. Phytomedicine 2010;17:23–7.
- Simos Y, Karkabounas S, Verginadis I, Charalampidis P, Filiou D, Charalabopoulos K, et al. Intra-peritoneal application of catechins and EGCG as in vivo inhibitors of ozone-induced oxidative stress. Phytomedicine 2011;18:579–85.
- Wagner H, Ulrich-Merzenich G. Synergy research: approaching a new generation of phytopharmaceuticals. Phytomedicine 2009;16:97–110.
- Charalampidis PS, Veltsistas P, Karkabounas S, Evangelou A. Blue CrO5 assay: a novel spectrophotometric method for the evaluation of the antioxidant and oxidant capacity of various biological substances. Eur J Med Chem 2009;44:4162–68.
- Hong YC, Lee KH, Yi CH, Ha EH, Christiani DC. Genetic susceptibility of term pregnant women to oxidative damage. Toxicol Lett 2002;129:255–62.
- Williams RJ, Spencer JPE, Rice-Evans C. Flavonoids: antioxidants or signaling molecules? Free Radic Biol Med 2004;36:838–49.
- Huang Q, Wu LJ, Tashiro SI, Onodera S, Ikejima T. Elevated levels of DNA repair enzymes and antioxidative enzymes by (1)-catechin in murine microglia cells after oxidative stress. J Asian Nat Prod Res 2006;8:61–71.
- Ullmann K, Wiencierz AM, Muller C, Thierbachi R, Steege A, Toyokuni S, et al. A high-throughput reporter gene assay to prove the ability of natural compounds to modulate glutathione peroxidase, superoxide dismutase and catalase gene promoters in V79 cells. Free Radic Res 2008;42:746–53.
- Wu CC, Hsu MC, Hsieh CW, Lin JB, Lai PH, Wung BS. Upregulation of heme oxygenase-1 by epigallocatechin-3-gallate via the phosphatidylinositol 3-kinase/Akt and ERK pathways. Life Sci 2006;78:2889–97.
- Na HK, Kim EH, Jung JH, Lee HH, Hyun JW, Surh YJ. (−)-Epigallocatechin gallate induces Nrf2-mediated antioxidant enzyme expression via activation of PI3 K and ERK in human mammary epithelial cells. Arch Biochem Biophys 2008;476:171–7.
- Hu Y, Guo DH, Liu P, Cao JJ, Wang YP, Yin J, et al. Bioactive components from the tea polyphenols influence on endogenous antioxidant defense system and modulate inflammatory cytokines after total-body irradiation in mice. Phytomedicine 2011;18:970–5.
- Hu Y, Cao JJ, Liu P, Guo DH, Wang YP, Yin J, et al. Protective role of tea polyphenols in combination against radiation-induced haematopoietic and biochemical alterations in mice. Phytother Res 2011;25:1761–9.
- Kumar N, Kant R, Maurya PK. Concentration-dependent effect of (−) epicatechin in hypertensive patients. Phytother Res 2010;24:1433–6.
- Halliwel B. Free radicals and antioxidants–quo vadis? Trends Pharmacol Sci 2011;32:125–30.